Abstract
Graphene/semiconductor heterojunction anodes can significantly enhance the output voltage by the photovoltaic effect. However, a significant challenge arises from the high intrinsic work function of heterojunction surfaces, which limits efficient electron emission. In this study, we explored the potential of low work function materials modified by Cs/Cs-O adsorption as anodes for thermionic (TI) converters through first principles calculations. The results demonstrate that the work functions of the graphene/MoS2 and the graphene/n-type Si surfaces with only Cs coating can decrease to 1.48 eV and 2.46 eV, respectively. The multiple Cs-O atoms co-adsorption enhances the dipole moment, resulting in a further reduction of the work function of the graphene/MoS2 surface to 1.25 eV. In addition, the impact of work function on the performance of TI converters is revealed by using concentrated solar energy as heat source. The highest conversion efficiency achieves 15.25% for the Cs-4O: Gr/MoS2 anode. This study establishes a robust foundation for further advancement of the TI converters with graphene/semiconductor heterojunction anodes.
Graphical abstract








Similar content being viewed by others
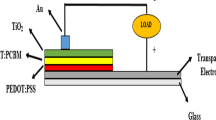
Data availability
The data that support the findings of this study are available from the corresponding author, Gang Xiao, upon reasonable request.
References
Escobar, P.V., Oyarzun, D.I., and Arias, A. 2021. Experimental study of a hybrid solar thermoelectric generator energy conversion system. Energy Conversion and Management 238: 113997. https://doi.org/10.1016/j.enconman.2021.113997.
Hatsopoulos, G.N., and Kaye, J. 1958. Measured thermal efficiencies of a diode configuration of a thermo electron engine. Journal of Applied Physics 29: 1124–1125. https://doi.org/10.1063/1.1723373.
Omair, Z., Scranton, G., Pazos-Outón, L.M., et al. 2019. Ultraefficient thermophotovoltaic power conversion by band-edge spectral filtering. Proceedings of the National Academy of Sciences USA 116: 15356–15361. https://doi.org/10.1073/pnas.1903001116.
Schwede, J.W., Sarmiento, T., Narasimhan, V.K., et al. 2013. Photon-enhanced thermionic emission from heterostructures with low interface recombination. Nature Communications 4: 1576. https://doi.org/10.1038/ncomms2577.
Deng, Y., Qiu, B., Lu, K., et al. 2020. Performance evaluation and efficiency enhancement of a space thermionic fuel element for thermal energy conversion and utilization. Applied Thermal Engineering 173: 115237. https://doi.org/10.1016/j.applthermaleng.2020.115237.
Datas, A., Ramos, A., Martí, A., et al. 2016. Ultra high temperature latent heat energy storage and thermophotovoltaic energy conversion. Energy 107: 542–549. https://doi.org/10.1016/j.energy.2016.04.048.
Qiu, H., Xu, H., Ni, M., et al. 2022. Photo-thermo-electric modeling of photon-enhanced thermionic emission with concentrated solar power. Solar Energy Materials and Solar Cells 246: 111922. https://doi.org/10.1016/j.solmat.2022.111922.
Prasad, A., Verma, J., Suresh, S., et al. 2022. Recent advancements in the applicability of SnO2-based photo-catalysts for hydrogen production: Challenges and solutions. Waste Disposal Sustainable Energy 4: 179–192. https://doi.org/10.1007/s42768-022-00105-3.
Yuan, H., Riley, D.C., Shen, Z.-X., et al. 2017. Back-gated graphene anode for more efficient thermionic energy converters. Nano Energy 32: 67–72. https://doi.org/10.1016/j.nanoen.2016.12.027.
Jacobs, R., Morgan, D., and Booske, J. 2017. Work function and surface stability of tungsten-based thermionic electron emission cathodes. APL Materials 5: 116105. https://doi.org/10.1063/1.5006029.
Yuan, H., Chang, S., Bargatin, I., et al. 2015. Engineering ultra-low work function of graphene. Nano Letters 15: 6475–6480. https://doi.org/10.1021/acs.nanolett.5b01916.
Su, C.Y., Spicer, W.E., and Lindau, I. 1983. Photoelectron spectroscopic determination of the structure of (Cs, O) activated GaAs (110) surfaces. Journal of Applied Physics 54: 1413–1422. https://doi.org/10.1063/1.332166.
Liu, Z., Sun, Y., Peterson, S., et al. 2008. Photoemission study of Cs–NF3 activated GaAs(100) negative electron affinity photocathodes. Applied Physics Letters 92: 241107. https://doi.org/10.1063/1.2945276.
Koeck, F.A.M., and Nemanich, R.J. 2017. Advances in thermionic energy conversion through single-crystal n-type diamond. Frontiers of Mechanical Engineering 3: 19. https://doi.org/10.3389/fmech.2017.00019.
Liu, L., Diao, Y., and Xia, S. 2020. Exploring the n-GaN films with Cs coating surface for low work function solar cell anode. Solar Energy 211: 560–568. https://doi.org/10.1016/j.solener.2020.10.002.
Liu, L., and Diao, Y. 2021. Exploring the electronic properties of Si-doped AlN (0001) surface with Cs adsorption layer for solar cell anode applications. Materials Science in Semiconductor Processing 132: 105899. https://doi.org/10.1016/j.mssp.2021.105899.
Fisher, D.G. 1974. The effect of Cs-O activation temperature on the surface escape probability of NEA (In, Ga)As photocathodes. IEEE Transactions on Electron Devices 21: 541–542. https://doi.org/10.1109/T-ED.1974.17963.
Zhuravlev, A.G., Khoroshilov, V.S., and Alperovich, V.L. 2019. Electron emission from GaAs(Cs, O): Transition from negative to positive effective affinity. Applied Surface Science 483: 895–900. https://doi.org/10.1016/j.apsusc.2019.04.010.
Li, S., Zhang, Y., Zhang, K., et al. 2022. Comparison of activation behavior of Cs-O and Cs-NF3-adsorbed GaAs(100)-β2(2×4) surface: From DFT simulation to experiment. Journal of Colloid and Interface Science 613: 117–125. https://doi.org/10.1016/j.jcis.2022.01.013.
Zhang, J., Zhang, Y., Qian, Y., et al. 2021. First-principles investigation of Cs-NF3 co-adsorption on GaAs(100)-β2(2×4) surface. Applied Surface Science 535: 147691. https://doi.org/10.1016/j.apsusc.2020.147691.
Lv, Y., Yu, X., Tan, C., et al. 2011. Deposition temperature effects on tungsten single-crystal layer by chemical vapor transport. Journal of Crystal Growth 329: 62–66. https://doi.org/10.1016/j.jcrysgro.2011.06.040.
Kobyakov, V.P., and Kalandarishvili, A.G. 2004. Performance of thermionic energy converters with oxygen-containing emitter and collector. Technical Physics 49: 775–778. https://doi.org/10.1134/1.1767890.
Datas, A. 2016. Hybrid thermionic-photovoltaic converter. Applied Physics Letters 108: 143503. https://doi.org/10.1063/1.4945712.
Bellucci, A., Mastellone, M., Serpente, V., et al. 2020. Photovoltaic anodes for enhanced thermionic energy conversion. ACS Energy Letters 5: 1364–1370. https://doi.org/10.1021/acsenergylett.0c00022.
Datas, A., and Vaillon, R. 2019. Thermionic-enhanced near-field thermophotovoltaics. Nano Energy 61: 10–17. https://doi.org/10.1016/j.nanoen.2019.04.039.
Datas, A., and Vaillon, R. 2019. Thermionic-enhanced near-field thermophotovoltaics for medium-grade heat sources. Applied Physics Letters 114: 133501. https://doi.org/10.1063/1.5078602.
Sahu, A., Mishra, S., Jain, P., et al. 2023. Plasma cascaded solid wastes for possible adsorption of NO2 in diesel exhaust. Waste Disposal Sustainable Energy 5: 383–393. https://doi.org/10.1007/s42768-023-00158-y.
Kuang, W., Yang, H., Ying, C., et al. 2021. Cost-effective, environmentally-sustainable and scale-up synthesis of vertically oriented graphenes from waste oil and its supercapacitor applications. Waste Disposal Sustainable Energy 3: 31–39. https://doi.org/10.1007/s42768-020-00068-3.
Hackley, J., Ali, D., DiPasquale, J., et al. 2009. Graphitic carbon growth on Si(111) using solid source molecular beam epitaxy. Applied Physics Letters 95: 133114. https://doi.org/10.1063/1.3242029.
Dang, X., Dong, H., Wang, L., et al. 2015. Semiconducting graphene on silicon from first-principles calculations. ACS Nano 9: 8562–8568. https://doi.org/10.1021/acsnano.5b03722.
Xu, Y., He, K.T., Schmucker, S.W., et al. 2011. Inducing electronic changes in graphene through silicon (100) substrate modification. Nano Letters 11: 2735–2742. https://doi.org/10.1021/nl201022t.
Hu, C., Liang, T., Chen, X., et al. 2021. Graphene-anode thermionic converter demonstrating total photon reflection. Applied Physics Letters 118: 083901. https://doi.org/10.1063/5.0039113.
Qiu, H., Lin, S., Xu, H., et al. 2022. Hybrid thermionic-photovoltaic converter with graphene-on-semiconductor heterojunction anode for efficient electricity generation. iScience 25: 105051. https://doi.org/10.1016/j.isci.2022.105051.
Li, W., Peng, W., Yang, Z., et al. 2020. Performance improvements and parametric design strategies of an updated thermionic-photovoltaic converter. Physica Scripta 95: 035208. https://doi.org/10.1088/1402-4896/ab501e.
Qiu, H., Lin, S., Xu, H., et al. 2023. Experimental and theoretical study on hybrid thermionic-photovoltaic energy converters with graphene/semiconductor Schottky junction. Energy Conversion and Management 276: 116584. https://doi.org/10.1016/j.enconman.2022.116584.
Wu, Y., Lin, Y., Bol, A.A., et al. 2011. High-frequency, scaled graphene transistors on diamond-like carbon. Nature 472: 74–78. https://doi.org/10.1038/nature09979.
Roy, K., Padmanabhan, M., Goswami, S., et al. 2013. Graphene–MoS2 hybrid structures for multifunctional photoresponsive memory devices. Nature Nanotech 8: 826–830. https://doi.org/10.1038/nnano.2013.206.
Bediako, D.K., Rezaee, M., Yoo, H., et al. 2018. Heterointerface effects in the electrointercalation of van der Waals heterostructures. Nature 558: 425–429. https://doi.org/10.1038/s41586-018-0205-0.
Ma, Y., Dai, Y., Guo, M., et al. 2011. Graphene adhesion on MoS2 monolayer: An ab initio study. Nanoscale 3: 3883. https://doi.org/10.1039/c1nr10577a.
Fang, Q., Li, M., Zhao, X., et al. 2022. van der Waals graphene/MoS2 heterostructures: Tuning the electronic properties and Schottky barrier by applying a biaxial strain. Materials Advances 3: 624–631. https://doi.org/10.1039/D1MA00806D.
Shi, J., Chen, L., Yang, M., et al. 2022. Interface contact and modulated electronic properties by external vertical strains and electric fields in graphene/MoS2 heterostructure. Current Applied Physics 39: 331–338. https://doi.org/10.1016/j.cap.2022.06.002.
Clark, S.J., Segall, M.D., Pickard, C.J., et al. 2005. First principles methods using CASTEP. Zeitschrift Für Kristallographie - Crystalline Materials 220: 567–570. https://doi.org/10.1524/zkri.220.5.567.65075.
Perdew, J.P., Burke, K., and Ernzerhof, M. 1996. Generalized gradient approximation made simple. Physical Review Letters 77: 3865–3868. https://doi.org/10.1103/PhysRevLett.77.3865.
Kitchin, J.R. 2009. Correlations in coverage-dependent atomic adsorption energies on Pd(111). Physical Review B 79: 205412. https://doi.org/10.1103/PhysRevB.79.205412.
Kamaratos, M. 2001. Adsorption kinetics of the Cs–O activation layer on GaAs(100). Applied Surface Science 185: 66–71. https://doi.org/10.1016/S0169-4332(01)00584-0.
Hogan, C., Paget, D., Garreau, Y., et al. 2003. Early stages of cesium adsorption on the As-rich c (2×8) reconstruction of GaAs(001): Adsorption sites and Cs-induced chemical bonds. Physical Review B 68: 205313. https://doi.org/10.1103/PhysRevB.68.205313.
Funding
This work was supported by the National Natural Science Foundation of China (No. 52325605), the Zhejiang Provincial Natural Science Foundation (No. LR20E060001) and the Fundamental Research Funds for the Central Universities (No. 2022ZFJH004).
Author information
Authors and Affiliations
Contributions
All authors contributed to the study conception and design. Weiting Sun: Conceptualization, Methodology, Investigation, Software, Validation, Formal analysis, Visualization, Data curation, Writing-original draft, Writing-review & editing. Haoran Xu: Writing-review & editing. Hao Qiu: Writing-review & editing. Gang Xiao: Supervision, Funding acquisition, Project administration.
Corresponding author
Ethics declarations
Competing interests
The authors declare that they have no competing financial interests.
Additional information
Publisher's Note
Springer Nature remains neutral with regard to jurisdictional claims in published maps and institutional affiliations.
Supplementary Information
Below is the link to the electronic supplementary material.
Rights and permissions
Springer Nature or its licensor (e.g. a society or other partner) holds exclusive rights to this article under a publishing agreement with the author(s) or other rightsholder(s); author self-archiving of the accepted manuscript version of this article is solely governed by the terms of such publishing agreement and applicable law.
About this article
Cite this article
Sun, W., Xu, H., Qiu, H. et al. Theoretical study on the Cs/Cs-O adsorbed graphene/semiconductor heterojunction anode for thermionic converters. Waste Dispos. Sustain. Energy (2024). https://doi.org/10.1007/s42768-024-00191-5
Received:
Revised:
Accepted:
Published:
DOI: https://doi.org/10.1007/s42768-024-00191-5