Abstract
To find a chemical sensor for detection of Cyclosarin (GF) nerve agent, we studied its interaction with B24N24, AlB23N24, B16N16 and AlB15N16 nanoclusters by means of density functional theory calculations. All calculations were investigated whit the M06 method and 6-311G(d,p) basis set. It was demonstrated that the interaction of GF with AlB23N24 and AlB15N16 is more stable than that of the B24N24 and B16N16. Thermodynamic parameters indicated that the AlB23N24 and AlB15N16 interactions with the GF are exothermic and spontaneous. Despite both AlB23N24 and AlB15N16 demonstrated strong adsorption, the electronic properties calculation indicated that AlB15N16 sensitive to the nerve agent adsorption. Our results predicted AlB15N16 has good potential as a sensor for the detection of GF.
Similar content being viewed by others
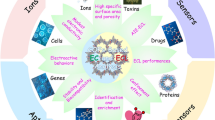
1 Introduction
Detection of nerve agents is an important chemical process for our safety and health [1]. Also, their identification is important for military and civilian defense resources [2]. Cyclosarin (GF) is a highly toxic organophosphate that belongs to the member of nerve used in the Gulf War (GW) operations [3, 4]. Various methods introduced for nerve agent detection including chromatography [5], mass spectroscopy [6], photoluminescent [7] and fluorescent sensors [8]. By the advent of nanotechnology, nanostructures have attracted an extensive attention as gas sensors because their surface/volume ratio is much higher than that of the conventional micro sensors which makes the large adsorption area available for adsorption process [9,10,11,12,13,14,15,16,17,18]. Boron nitride (BN) nanostructures have been introduced as a capable tool for the adsorption of compounds due to their high amount of bonding energy [19, 20]. Unlike the carbon nanostructures, the BN nanostructures are wide bandgap semiconductors, offer greater applications in electronic devices and sensors [21, 22]. Recently fullerene-like nanoclusters of BN nanostructures have attracted considerable attention [23,24,25,26,27].
Oku et al. [28, 29] have synthesized B16N16 and B24N24 nanoclusters, detected by laser desorption time-of flight mass spectrometry. Seifert et al. [30] indicated that the B16N16 with 4 and 6 membered B-N rings is excellent stable BN nanoclusters and Wu and Jiao [31] indicated that B24N24 nanocluster with isolated 4, 6 and 8-membered B-N rings are more stable than other structures of B24N24. Various applications and properties of B16N16 and B24N24 nanoclusters have been widely studied [32,33,34,35]. For example, Shakerzadeh et al. have shown the adsorption of phosgene over the B12N12 and B16N16 nanoclusters using density functional theory (DFT) calculations. They reported that the AlB11N12 and AlB15N16 nanoclusters could be promising sensor for phosgene detection [36]. The structure and electronic properties of B24N24 nanocluster functionalized with formaldehyde molecule was studied by Rostami et al. [37] using DFT calculations. They found B24N24 is suitable for formaldehyde detection. Soltani et al. have shown the adsorption of 5-AVA drug over the B12N12 and B16N16 nanoclusters using DFT calculations. They reported that the nanoclusters could be suitable for 5-AVA drug delivery [38]. Computational methods significantly help the experimentalist to understand different compounds behavior [39].
In this research, the interaction of GF with the B16N16 and B24N24 nanoclusters were surveyed by using theoretical calculations. Moreover, we doped Al atom instead of B atom in the B16N16 and B24N24 (AlB15N16 and AlB23N24) to find the suitable gas sensor of GF nerve agent (Fig. 1). The obtained results may provide a new insight to the gas sensor nanotechnology.
Optimized configurations, the FMO (HOMO and LUMO) and MEP plots of the GF, B24N24 and AlB23N24. (The color scheme for MEP surface is red-electron rich or partially negative charge; blue-electron deficient or partially positive charge; light blue-slightly electron deficient region; yellow-slightly electron rich region, respectively)
2 Computational method
Adsorption of GF onto the B24N24, AlB23N24, B16N16 and AlB15N16 surfaces was investigated using density functional theory (Figs. 1, 2, 3, 4, 5 and 6). All calculations were carried out using GAMESS program package [40] with the M06 method and 6-311G(d,p) basis set. The previous reports were indicated that M06 method is appropriate for predict electronic and structural properties [41, 42] and 6-311G(d,p) basis set has been known suitable for nanostructure systems [43, 44]. The adsorption energies (Ead) were calculated with the following equations:
where E(GF/B24N24), E(GF/AlB23N24), E(GF/B16N16) and E(GF/AlB15N16) are the total energies of the B24N24, AlB23N24, B16N16 and AlB15N16 interacted with GF, E(GF), E(B24N24), E(AlB23N24), E(B16N16) and E(AlB15N16) are the total energy of the lone GF, B24N24, AlB23N24, B16N16 and AlB15N16, respectively. EBSSE is the basis set superposition error (BSSE) corrected for all adsorption energy [45]. The thermodynamic parameters such as Gibbs free energy (ΔG), enthalpy (ΔH) and entropy (ΔS) (T = 298.15 K and P = 1 atm) were investigated with the M06 method and 6-311G(d,p) basis set. Furthermore, frontier molecular orbitals (FMO), molecular electrostatic potential (MEP), natural bond orbital (NBO), and density of states (DOS) analysis were computed [38].
3 Result and discussion
The optimized structure, FMO and MEP plots for GF were provided in Fig. 1. As shown in MEP plot, the negative charges (red color) are mainly localized over the carbonyl oxygen (O head), F atom (F head) and etheric oxygen (O head), which can be interacted with electrophile sites of nanocluster. The calculated P = O, P–O and P–F bond lengths were calculated 1.46, 1.59 and 1.59 Å, respectively. The FMO plot for GF is located on all atoms in the HOMO and LUMO regions.
3.1 The adsorption of GF on the B24N24 and AlB23N24
The most stable configuration of B24N24 and AlB23N24 nanoclusters was shown in Fig. 1. The calculated B–N bond length between 6–8, 6–4 and 4–8 membered rings are 1.42, 1.49 and 1.47 Å, respectively, and is completely in agreement with the previous reports [37, 46]. The calculated Al–N bond length in AlB23N24 for 6–8, 6–4 and 4–8 membered rings are 1.77, 1.83 and 1.80 Å, respectively. The MEP plots of the B24N24 and AlB23N24 indicated the electrostatic potential in the Al atom of AlB23N24 is significantly more positive (blue color) compared to the B and N atoms which makes it the most electrophilic site for GF molecule.
The reactivity of GF with nanoclusters has been studied in the various adsorption sites (Fig. 2). The adsorption energies of GF with the B24N24 in state A, B and C were calculated to be − 9.60 and − 5.57 and − 5.51 kcal mol−1, respectively (Table 1). Nejati et al. indicated the interaction of GF with BN nanosheet with weak adsorption energies of − 0.32 kcal mol−1 at the B3LYP-D method and 6-31G* basis set [47]. Equilibrium distances between GF and nanocluster in state A, B and C were obtained to be 1.62, 2.67 and 2.43 Å, respectively. These results were shown the interaction of state A (carbonyl oxygen) is stronger than that of state B (F atom) and C (etheric oxygen) since adsorption energies were indicated in state B is more negative than that of state B and C. The adsorption energy of GF/AlB23N24 was calculated − 50.63, − 23.14 and − 30.61 kcal mol−1 with equilibrium distances 1.83, 1.88 and 1.98 Å in state D, E and F, respectively. These results indicated that GF adsorption on the AlB23N24 is stronger than that on the pure one. NBO charge transfers in state D, E and F were calculated 0.183, 0.151 and 0.160 e, respectively. Positive values of charge transfers are indicated charge transfer from GF to the nanoclusters.
Furthermore, the thermodynamic parameters (Gibbs free energy (ΔG), enthalpy (ΔH) and entropy (ΔS)) were calculated and listed in Table 1. The ΔH values for GF/B24N24 in state A, B and C were calculated − 12.35, − 6.32 and − 7.54 kcal mol−1, respectively, and in GF/AlB23N24 complexes were obtained − 52.88, − 27.75 and − 33.62 kcal mol−1 in state D, E and F, respectively. The negative values of ΔH indicated that the reactions are exothermic. The ΔG values were calculated 0.70, 5.30, 5.60, − 42.54, − 12.25 and − 18.89 kcal mol−1 in state A, B, C, D, E and F, respectively. Therefore, B24N24 interaction is non-spontaneous while AlB23N24 interaction is spontaneous. The calculated ΔG values are less negative compared to the ΔH values, indicating an entropy reduction. The calculated negative values of ΔS confirm this matter. These results were indicated the interaction of the AlB23N24 is more suitable compared to the B24N24 nanocluster. The MEP plot of GF/AlB23N24 in Fig. 3, shows significant change after adsorption in the electrostatic potential. These results reinforce the fact that GF gets chemically adsorbed on the AlB23N24.
Some of the electronic properties like HOMO, LUMO and Eg were reported in Table 1. The Eg is decreased from 7.04 eV in B24N24 to 5.55 eV in AlB23N24. Salari investigated Eg of B24N24 7.11 eV at the M06/6-31G* level of theory [48]. The HOMO and LUMO values in the B24N24 and AlB23N24 nanoclusters were shifted to higher energy after adsorption (except GF/B24N24 in state B). These results indicated that the adsorption of GF on the B24N24 and AlB23N24 nanoclusters destabilizes the HOMO and LUMO levels. In GF/B24N24 complexes the Eg values were revealed that no significant change compared with pure B24N24 (− 4.62%, − 0.86% and − 0.09% in state A, B and C, respectively) but in GF/AlB23N24, Eg values were increased from 5.55 eV in pure AlB23N24 to 6.45, 6.60 and 6.44 eV in state D, E and F, respectively. The DOS plot of the pure AlB23N24 and GF/AlB23N24 in state D indicated this change (Fig. 3). The change of Eg will exponentially increase the sensitivity and electrical conductivity of the nanostructure [49,50,51]. Therefore, it is clear that the GF/AlB23N24 is more sensitive rather than B24N24. change of Eg indicated that the AlB23N24 can detect the GF.
3.2 The adsorption of GF on the B16N16 and AlB15N16
Furthermore, we examined adsorption of GF molecule on the B16N16 and AlB15N16 nanoclusters. The optimized structures, MEP and FMO plots of B16N16 and AlB15N16 are shown in Fig. 4. The B–N bond lengths of B16N16 in 6–6 and 6–4 membered rings were calculated 1.45 and 1.47 Å, respectively and Al–N bond lengths for AlB15N16 were calculated 1.80 and 1.81 Å in 6–6 and 6–4 membered rings, respectively.
After adsorption of GF on the B16N16, adsorption energies were calculated − 14.30, − 1.46 and − 16.52 kcal mol−1 in state G, H and I, respectively (Table 2). When GF is located from its etheric oxygen (state I) on the B16N16 nanocluster, it reoriented and attached from carbonyl oxygen to the B16N16 (Fig. 5). Equilibrium distances in state G, H and I were calculated 1.59, 2.53 and 1.57 Å, respectively. The adsorption energies of AlB15N16 complexes were obtained − 54.84, − 28.28 and − 35.22 kcal mol−1 in state J, K and L, respectively. These results were indicated the interaction of the AlB15N16 nanocluster is stronger than that of the B24N24, AlB23N24 and B16N16 nanoclusters. NBO charge transfer were calculated 0.190, 0.157 and 0.176 e and equilibrium distances were investigated 1.82, 1.86 and 1.93 Å in state J, K and L, respectively.
The ΔH (ΔG) values of GF/B16N16 and GF/AlB15N16 were calculated − 17.86 (− 3.94), − 2.19 (9.80), − 19.76 (− 6.12), − 57.53 (− 45.79), − 32.06 (− 20.22) and − 38.80 (− 24.63) kcal mol−1 in state G, H, I, J, K and L, respectively. The negative values of ΔH and ΔG indicated the reactions are exothermic and spontaneous. Upon adsorption of GF on AlB15N16 in state J, their MEP changes significantly. These results confirm the fact that GF gets chemically adsorbed on the AlB15N16.
The HOMO, LUMO and Eg values for B16N16 and AlB15N16 at the M06 method were shown in Table 2. The HOMO values for the B16N16 and AlB15N16 were − 8.06 and − 7.65 eV while the LUMO values were − 1.25 and − 3.06 eV, respectively. The Eg is decreased from 6.82 eV in B16N16 to 4.59 eV in AlB15N16. Soltani et al. calculated Eg of B16N16 6.37 eV at the B3LYP method [52] and Shakerzadeh et al. investigated Eg of B16N16 and AlB15N16 6.17 and 3.97 eV at the M06-2X method [36]. The HOMO and LUMO values after adsorption process were increased to higher energy and destabilizes the HOMO and LUMO levels. Eg values after adsorption in GF/B16N16 reveal that no significant change compared with pure B16N16 and indication of B16N16 nanocluster cannot detect the GF. The Eg values are increased from 4.59 eV in AlB15N16 to 5.92, 6.09 and 6.42 eV in state J, K and L, respectively. Compared to the B24N24, AlB23N24 and B16N16, the Eg represent more sensitivity in AlB15N16 (29.00% in state J, 32.71% in state K and 39.95% in state L). The DOS plot of AlB15N16 and GF/B15N16 in state J in Fig. 6 confirm this increase. The change of Eg was indicated the AlB15N16 nanocluster can detect the GF nerve agent.
4 Conclusions
In this study, the adsorption of the GF nerve agent on the B24N24, AlB23N24, B16N16 and AlB15N16 was investigated using DFT calculations. The doped atom (AlB23N24 and AlB15N16) nanoclusters facilitate the interaction of the GF compared to the pure B24N24 and B16N16 nanoclusters. Thermodynamic calculations were indicated adsorption of GF on the AlB23N24 and AlB15N16 in all states are exothermic and spontaneous. The results of Eg and DOS plots were shown that the AlB15N16 has greater sensitivity to the GF than the B24N24, AlB23N24 and B16N16. Therefore, the AlB15N16 may be used in GA sensor devices.
References
Mandal D, Mondal B, Das AK (2010) Isomerization and decomposition of a model nerve agent: a computational analysis of the reaction energetics and kinetics of dimethyl ethylphosphonate. J Phys Chem A 114(39):10717–10725
Dawson RM (1994) Review of oximes available for treatment of nerve agent poisoning. J Appl Toxicol 14(5):317–331
Heaton KJ, Palumbo CL, Proctor SP, Killiany RJ, Yurgelun-Todd DA, White RF (2007) Quantitative magnetic resonance brain imaging in US army veterans of the 1991 Gulf War potentially exposed to sarin and cyclosarin. Neurotoxicology 28(4):761–769
Kuca K, Pícha J, Jun D (2006) Reactivation potency of new group of acetylcholinesterase reactivators and their comparison with currently available oximes. Acta Medica (Hradec Kralove) 49(4):233–235
Kientz CE (1998) Chromatography and mass spectrometry of chemical warfare agents, toxins and related compounds: state of the art and future prospects. J Chromatogr A 814(1–2):1–23
Black RM, Clarke RJ, Read RW, Reid MTJ (1994) Application of gas chromatography–mass spectrometry and gas chromatography–tandem mass spectrometry to the analysis of chemical warfare samples, found to contain residues of the nerve agent sarin, sulphur mustard and their degradation products. J Chromatogr A 662(2):301–321
Kim S, Cho B, Sohn H (2012) Detection of nerve agent stimulants based on photoluminescent porous silicon interferometer. Nanoscale Res Lett 7:1–6
Dale TJ, Rebek J Jr (2006) Fluorescent sensors for organophosphorus nerve agent mimics. J Am Chem Soc 128(14):4500–4501
Cui Y, Wei Q, Park H, Lieber CM (2001) Nanowire nanosensors for highly sensitive and selective detection of biological and chemical species. Science 293(5533):1289–1292
Peyghan AA, Moradi M (2014) Influence of antisite defect upon decomposition of nitrous oxide over graphene-analogue SiC. Thin Solid Films 552:111–115
Jha S, Wang HE, Kutsay O, Jelenković EV, Chen KJ, Bello I, Kremnican V, Zapien JA (2012) Exploiting nanostructure-thin film interfaces in advanced sensor device configurations. Vacuum 86(6):757–760
Eslami M, Peyghan AA (2015) DNA nucleobase interaction with graphene like BC3 nano-sheet based on density functional theory calculations. Thin Solid Films 589:52–56
Peyghan AA, Rastegar SF, Hadipour NL (2014) DFT study of NH3 adsorption on pristine, Ni- and Si-doped graphynes. Phys Lett Sect A Gen At Solid State Phys 378(30–31):2184–2190
Dos Santos RB, Mota FDB, Rivelino R, Kakanakova-Georgieva A, Gueorguiev GK (2016) Van der Waals stacks of few-layer h-AlN with graphene: an ab initio study of structural, interaction and electronic properties. Nanotechnology 27(14):145601
Rad AS (2018) Comparison of X12Y12(X = Al, B and y = N, P) fullerene-like nanoclusters toward adsorption of dimethyl ether. J Theor Comput Chem. https://doi.org/10.1142/S0219633618500128
Salimifard M, Rad AS, Mahanpoor K (2017) Surface interaction of H2S, SO2, and SO3 on fullerene-like gallium nitride (GaN) nanostructure semiconductor. Solid State Commun 265:6–11
Rad AS, Aghaei SM, Aali E, Peyravi M (2017) Study on the electronic structure of Cr- and Ni-doped fullerenes upon adsorption of adenine: a comprehensive DFT calculation. Diam Relat Mater 77:116–121
Rad AS, Ayub K (2017) O3 and SO2 sensing concept on extended surface of B12N12 nanocages modified by Nickel decoration: a comprehensive DFT study. Solid State Sci 69:22–30
Chang CM, Jalbout AF (2010) Metal induced amino acid adsorption on nanotubes. Thin Solid Films 518(8):2070–2076
Mirzaei M, Yousefi M (2013) Modified (n, 0) BN nanotubes (n = 3–10) by acetic acids: DFT studies. Superlattices Microstruct 55:1–7
Guo G, Lin J (2005) Systematic ab initio study of the optical properties of BN nanotubes. Phys Rev B 71(16):165402
Solimannejad M, Noormohammadbeigi M (2017) Boron nitride nanotube (BNNT) as a sensor of hydroperoxyl radical (HO2): A DFT study. J Iran Chem Soc 14(2):471–476
Paine RT, Narula CK (1990) Synthetic routes to boron nitride. Chem Rev 90(1):73–91
Wu HS, Zhang FQ, Xu XH, Zhang CJ, Jiao H (2003) Geometric and energetic aspects of aluminum nitride cages. J Phys Chem A 107(1):204–209
Zhu HY, Schmalz TG, Klein DJ (1997) Alternant boron nitride cages: a theoretical study. Int J Quantum Chem 63(2):393–401
Golberg D, Bando Y, Stéphan O, Kurashima K (1998) Octahedral boron nitride fullerenes formed by electron beam irradiation. Appl Phys Lett 73(17):2441–2443
Wang Q, Sun Q, Jena P, Kawazoe Y (2009) Potential of AlN nanostructures as hydrogen storage materials. ACS Nano 3(3):621–626
Oku T, Nishiwaki A, Narita I, Gonda M (2003) Formation and structure of B24N24 clusters. Chem Phys Lett 380(5–6):620–623
Oku T, Nishiwaki A, Narita I (2004) Formation and atomic structures of BnNn (n = 24-60) clusters studied by mass spectrometry, high-resolution electron microscopy and molecular orbital calculations. Phys B Condens Matter 351(1–2):184–190
Seifert G, Fowler PW, Mitchell D, Porezag D, Frauenheim T (1997) Boron-nitrogen analogues of the fullerenes: electronic and structural properties. Chem Phys Lett 268(5–6):352–358
Wu HS, Jiao H (2004) What is the most stable B24N24 fullerene? Chem Phys Lett 386(4–6):369–372
Ma Z, Zhang Y, Li F, Chen H (2016) Comparative study of H2 adsorption on B24N24, Al24N24 and B12Al12N24 clusters. Comput Mater Sci 117:71–75
Koi N, Oku T, Suganuma KS (2005) Effects of endohedral element in B24N24 clusters on hydrogenation studied by molecular orbital calculations. Physica E 29(3–4):541–545
Rouzbehani GM, Boshra A, Seif A (2009) B24N24 nanocages: a GIAO density functional theory study of 14 N and 11B nuclear magnetic shielding and electric field gradient tensors. Monatsh Chem 140(3):255–263
Mileev MA, Kuzmin SM, Parfenyuk VI (2006) Ab initio calculations of structure and stability of small boron nitride clusters. J Struct Chem 47(6):1016–1021
Shakerzadeh E, Khodayar E, Noorizadeh S (2016) Theoretical assessment of phosgene adsorption behavior onto pristine, Al- and Ga-doped B12N12 and B16N16 nanoclusters. Comput Mater Sci 118:155–171
Rostami Z, Pashangpour M, Moradi R (2017) DFT study on the chemical sensing properties of B24N24 nanocage toward formaldehyde. J Mol Graph Model 72:129–135
Soltani A, Sousaraei A, Bezi Javan M, Eskandari M, Balakheyli H (2016) Electronic and optical properties of 5-AVA-functionalized BN nanoclusters: a DFT study. New J Chem 40(8):7018–7026
Pargolghasemi P, Saleh Hoseininezhad-Namin M, Parchehbaf Jadid A (2017) Prediction of activities of BRAF (V600E) inhibitors by SW-MLR and GA-MLR methods. Curr Comput aided Drug Design 13(3):249–261
Schmidt MW, Baldridge KK, Boatz JA, Elbert ST, Gordon MS, Jensen JH, Koseki S, Matsunaga N, Nguyen KA, Su S, Windus TL, Dupuis M, Montgomery JA Jr (1993) General atomic and molecular electronic structure system. J Comput Chem 14(11):1347–1363
Walker M, Harvey AJA, Sen A, Dessent CEH (2013) Performance of M06, M06-2X, and M06-HF density functionals for conformationally flexible anionic clusters: M06 functionals perform better than B3LYP for a model system with dispersion and ionic hydrogen-bonding interactions. J Phys Chem A 117(47):12590–12600
Rostami Z, Hosseinian A, Monfared A (2018) DFT results against experimental data for electronic properties of C60 and C70 fullerene derivatives. J Mol Graph Model 81:60–67
Kazemi M, Rad AS (2017) Sulfur mustard gas adsorption on ZnO fullerene-like nanocage: Quantum chemical calculations. Superlattices Microstruct 106:122–128
Deng WQ, Xu X, Goddard WA (2004) New alkali doped pillared carbon materials designed to achieve practical reversible hydrogen storage for transportation. Phys Rev Lett 92(16):166103-1–166103-4
Boys SF, Bernardi F (1970) The calculation of small molecular interactions by the differences of separate total energies. Some procedures with reduced errors. Mol Phys 19(4):553–566
Hazrati MK, Javanshir Z, Bagheri Z (2017) B24N24 fullerene as a carrier for 5-fluorouracil anti-cancer drug delivery: DFT studies. J Mol Graph Model 77:17–24
Nejati K, Arshadi S, Vessally E, Bekhradnia A, Hosseinian A (2017) Cyclosarin nerve agent interaction with the pristine, Stone Wales defected, and Si-doped BN nanosheets: theoretical study. Physica E 90:143–148
Salari AA (2017) Are the inorganic B24N24, Al24N24, B24P24 and Al24P24 nanoclusters synthesizable or not? A DFT study. Inorg Chim Acta 456:18–23
Hoseininezhad-Namin MS, Pargolghasemi P, Alimohammadi S, Rad AS, Taqavi L (2017) Quantum Chemical Study on the adsorption of metformin drug on the surface of pristine, Si- and Al-doped (5, 5) SWCNTs. Physica E 90:204–213
Soltani A, Javan MB, Hoseininezhad-Namin MS, Tajabor N, Lemeski ET, Pourarian F (2017) Interaction of hydrogen with Pd- and co-decorated C24 fullerenes: density functional theory study. Synth Metals 234:1–8
Safari L, Vessally E, Bekhradnia A, Hosseinian A, Edjlali L (2017) A Density functional theory study of the sensitivity of two-dimensional BN nanosheet to nerve agents cyclosarin and tabun. Thin Solid Films 623:157–163
Soltani A, Baei MT, Tazikeh Lemeski E, Pahlevani AA (2014) The study of SCN-adsorption on B12N12and B16N16nano-cages. Superlattices Microstruct 75:716–724
Author information
Authors and Affiliations
Corresponding author
Ethics declarations
Conflict of interest
The authors declare that they have no known competing financial interests or personal relationships that could have appeared to influence the work reported in this paper.
Additional information
Publisher's Note
Springer Nature remains neutral with regard to jurisdictional claims in published maps and institutional affiliations.
Rights and permissions
About this article
Cite this article
Karjabad, K.D., Mohajeri, S., Shamel, A. et al. Boron nitride nanoclusters as a sensor for Cyclosarin nerve agent: DFT and thermodynamics studies. SN Appl. Sci. 2, 574 (2020). https://doi.org/10.1007/s42452-020-2411-2
Received:
Accepted:
Published:
DOI: https://doi.org/10.1007/s42452-020-2411-2