Abstract
Accurate computer simulations require the selection of suitable material models and precise prediction of their parameters. In the fields of impact engineering and plastic working, stress–strain relations that include the post-necking regime up to fracture are crucial for predicting the behavior correctly. However, obtaining suitable stress–strain relations after necking requires some form of correction and adjustment for stress and/or strain. This study applies a stepwise modeling method for post-necking characterization that only utilizes the local strain field obtained from tensile experiments to precisely measure stress–strain relations at high strain rates. The effects of the notch radius of specimens on stress–strain relations were examined to measure stress–strain relations with large strain near the stress triaxiality of 1/3. The study also discusses adequate resolution for precise stress–strain measurements. Subsequently, specimens with suitable notch radius were used to measure stress–strain relations of plate specimens of aluminum alloy 2024-T3 at high strain rates. The study also examined the effects of strain rate on the flow stress and fracture strain of aluminum alloy 2024-T3.

















Similar content being viewed by others
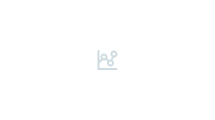
Data availability
The data generated and/or analysed in this present study are not publicly disclosed but are available from the corresponding author on reasonable request.
References
Bridgman PW (1952) Studies in large plastic flow and fracture. McGraw-Hill, New York
Tsuchida N, Inoue T, Enami K (2012) Estimations of the true stress and true strain until just before fracture by the stepwise tensile test and bridgman equation for various metals and alloys. Mater Trans 53:133–139
Zhang K, Li Z (1994) Numerical analysis of the stress–strain curve and fracture initiation for ductile material. Eng Fract Mech 49:235–241
Cabezas EE, Celentano DJ (2004) Experimental and numerical analysis of the tensile test using sheet specimens. Finite Elem Anal Des 40:555–575
Chu TC, Ranson WF, Sutton MA (1985) Applications of digital-image-correlation techniques to experimental mechanics. Exp Mech 25:232–244
Sutton MA, Orteu JJ, Schreier H (2009) Image correlation for shape, motion and deformation measurements. Basic concepts, theory and applications. Springer, New York
Wynn HP (1992) Introduction to Kiefer (1959) Optimum experimental designs, breakthroughs in statistics, foundations and basic theory. In: Kotz S, Johnson NL (eds), pp 395–399
Kiefer JC (1992), Optimum experimental designs, breakthroughs in statistics, foundations and basic theory. In: Kotz S, Johnson NL (eds), pp 400–436
Besnard G, Hild F, Roux S (2006) “Finite-element” displacement fields analysis from digital images: application to Portevin–Le Châtelier bands. Exp Mech 46:789–803
Tarigopula V, Hopperstad OS, Langseth M, Clausen AH, Hild F, Lademo O-G, Eriksson M (2008) A study of large plastic deformations in dual phase steel using digital image correlation and FE analysis. Exp Mech 48:181–196
Yazzie KE, Fei H, Jiang H, Chawla N (2012) A self-consistent approach for necking correction in tensile specimens with rectangular cross-section using a novel mirror fixture. Metall Mater Trans A 43:5058–5066
Tsuda T, Abe A, Hayashi H, Tanimura S (2014) In: Proceedings of 27th JSME Computational Mechanics Division Conference (2014), Paper number 2908 (in Japanese)
Tanimura S, Tsuda T, Abe A, Hayashi H, Jones N (2014) Comparison of rate-dependent constitutive models with experimental data. Int J Impact Eng 69:104–113
Avril S, Pierron F (2007) General framework for the identification of constitutive parameters from full-field measurements in linear elasticity. Int J Solids Struct 44:4978–5002
Avril S, Bonnet M, Bretelle AS, Grédiac M, Hild F, Ienny P, Latourte F, Lemosse D, Pagano S, Pagnacco E, Pierron F (2008) Overview of identification methods of mechanical parameters based on full-field measurements. Exp Mech 48:381–402
Grediac M, Hild F (2021) Full-field measurements and identification in solid mechanics. ISTE/Wiley London
Mathieu F, Leclerc H, Hild F, Roux S (2015) Estimation of elastoplastic parameters via weighted FEMU and integrated-DIC. Exp Mech 55:105–119
Pagnacco E, Lemosse D, Hild F, Amiot F (2005) Inverse strategy from displacement field measurement and distributed forces using FEA. In: SEM annual conference and exposition on experimental and applied mechanics, Portland, 7–9 June 2005
Pierron F, Grédiac M (2012) The virtual fields method: extracting constitutive mechanical parameters from full-field deformation measurements. Springer, New York
Ponthot J-P, Kleinermann J-P (2006) A cascade optimization methodology for automatic parameter identification and shape/process optimization in metal forming simulation. Comput Methods Appl Mech Eng 195:5472–5508
Murata M, Yoshida Y, Nishiwaki T (2018) Stress correction method for flow stress identification by tensile test using notched round bar. J Mater Process Technol 251:65–72
Shapiro A (2011) How to use LS-OPT for parameter estimation—hot stamping and quenching applications. In: 8th european LS-DYNA conference
Stander N, Witowski K, Ilg C, Haufe A, Helbig M, Koch D (2017) Application of digital image correlation to material parameter identification using LS-OPT. In: 11th European LS-DYNA conference 2017
Ilg C, Haufe A, Koch D, Stander N, Witowski K, Svedin Å, Liewald M (2018) Application of a full-field calibration concept for parameter identification of HS-steel with LS-OPT®. In: 15th international LS-DYNA users conference
Marth S, Häggblad H-Å, Oldenburg M, Östlund R (2016) Post necking characterisation for sheet metal materials using full field measurement. J Mater Process Technol 238:315–324
Papasidero J, Doquet V, Mohr D (2015) Ductile fracture of aluminum 2024–T351 under proportional and non-proportional multi-axial loading: Bao-Wierzbicki results revisited. Int J Solids Struct 69–70:459–474
Warren AS (2004) Developments and challenges for aluminum—a boeing perspective. In: Nie JF, Morton AJ, Muddle BC (eds) Proceedings of the 9th international conference on aluminium alloys, pp 24–31
Marth S, Djebien S, Kajberg J, Häggblad HÅ (2021) Stepwise modelling method for post necking characterisation of anisotropic sheet metal. Modell Simul Mater Sci Eng 29(8):085001
Metals Handbook (1990) Properties and selection: nonferrous alloys and special-purpose materials, 10 edn vol. 2,. ASM International, pp 70–71
Gruben G, Fagerholt E, Hopperstad OS, Børvik T (2011) Fracture characteristics of a cold-rolled dual-phase steel. Eur J Mech A Solids 30(3):204–218
Kajberg J, Lindkvist G (2004) Characterisation of materials subjected to large strains by inverse modelling based on in-plane displacement fields. Int J Solids Struct 41(13):3439–3459
Eman J, Sundin KG, Oldenburg M (2009) Spatially resolved observations of strain fields at necking and fracture of anisotropic hardened steel sheet material. Int J Solids Struct 46(13):2750–2756
Smith GC (1949) Age hardening of metals. Prog Met Phys 1:163–234
Kim JH, Kobayashi E, Sato T (2015) Influence of natural aging time on two-step aging behavior of Al-Mg-Si (-Cu) alloys. Mater Trans 56(11):1771–1780
Winter L, Einer F, Geisler C, Hellmig RJ, Hockauf K, Lampke T (2019) Influence of the heat-treatment prior to plastic deformation on the aging behavior and the hardness of the aluminum alloy 6056. IOP Conf Ser 480:012031
Delaunois F, Denil E, Marchal Y, Vitry V (2017) Accelerated aging and Portevin-Le Chatelier effect in AA 2024. Mater Sci Forum 879:524–529
Nishida M, Taniguchi S, Ziyi Su, Sunda M, Murata M (2023) Effects of strain rate on stress-strain curves in 2024 aluminum alloy after solution heat treatment. Mater Trans 64(2):506–513
Johnson GR, Cook WH (1983) In: Proc. 7th int. symp. on ballistics, pp 541–547
Lesuer D (1999) Lawrence Livermore National Laboratory, UCRL-ID-134691
Rusinek A, Rodríguez-Martínez JA (2009) Thermo-viscoplastic constitutive relation for aluminium alloys, modeling of negative strain rate sensitivity and viscous drag effects. Mater Des 30:4377–4390
Seidt JD, Gilat A (2013) Plastic deformation of 2024–T351 aluminum plate over a wide range of loading conditions. Int J Solids Struct 50:1781–1790
Mukai T, Higashi K, Tsuchida S, Tanimura S (1993) Influence of strain rate on tensile properties in some commercial aluminum alloys. J Jpn Inst Light Met 43(5):252–257 (in Japanese)
Mukai T, Higashi K, Tanimura S (1994) Influence of the magnesium concentration on the relationship between fracture mechanism and strain rate in high purity Al-Mg alloys. Mater Sci Eng A 176(1–2):181–189
Acknowledgements
This work was partially supported by The Light Metal Educational Foundation, Inc. The microstructure observation was carried out by UACJ Corporation. The authors would like to express their gratitude for the technical and financial support.
Author information
Authors and Affiliations
Corresponding author
Ethics declarations
Conflict of interest
The authors declare no conflicts of interest associated with this manuscript.
Additional information
Publisher's Note
Springer Nature remains neutral with regard to jurisdictional claims in published maps and institutional affiliations.
M. Nishida is a member of SEM.
Rights and permissions
Springer Nature or its licensor (e.g. a society or other partner) holds exclusive rights to this article under a publishing agreement with the author(s) or other rightsholder(s); author self-archiving of the accepted manuscript version of this article is solely governed by the terms of such publishing agreement and applicable law.
About this article
Cite this article
Djebien, S., Nohara, S., Nishida, M. et al. Strain Rate and Notch Radius Effects on Evaluating the Stress–Strain Relations Using the Stepwise Modeling Method. J. dynamic behavior mater. 10, 26–39 (2024). https://doi.org/10.1007/s40870-023-00397-4
Received:
Accepted:
Published:
Issue Date:
DOI: https://doi.org/10.1007/s40870-023-00397-4