Abstract
It has been reported that ball milling and adding catalyst can improve hydrogen desorption properties of MgH2. In this study, simultaneous effect of adding catalyst and ball milling on hydrogen desorption properties of MgH2 was studied. Mechanical alloying and heat treatment methods were used to synthesize MgNi4Y intermetallic as a catalyst. In this regard, pure Mg, Ni, and Y elemental powders were ball milled in different conditions and then heat treated at 1073 K (800 °C) for 4 hours. XRD and FESEM methods were used to investigate properties of the samples. It was found that, after 10 hours of ball milling and then heat treating at 1073 K (800 °C), MgNi4Y intermetallic was formed almost completely. The results of Sievert tests showed that as-received MgH2 did not release any significant amount of hydrogen at 623 K (350 °C). But, after ball milling for 10 hours, 0.8 wt pct hydrogen was released from MgH2 at 623 K (350 °C) in 40 minutes. Adding 10 wt pct catalyst via ball milling to MgH2 led to releasing 3.5 wt pct hydrogen in the same conditions. In addition, increasing ball milling time from 10 to 65 hours increased the amount of released hydrogen from 51 to 85 pct of theoretical hydrogen desorption value and improved kinetic of desorption process.
Similar content being viewed by others
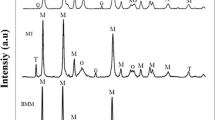
Avoid common mistakes on your manuscript.
Introduction
Considering the increasing pollution and exploitation of fossil energy reservoirs, using new energy resources is essential for future industries.[1] In this regard, hydrogen could be used as an energy source since it is a clean and renewable fuel for various applications. The major challenges in developing new hydrogen storage materials are improving energy storage density, desorption kinetics, cycle life, and ready availability at reduced cost.[2]
It is well known that Mg is the best candidate for hydrogen storage due to its high storage capacity (MgH2, up to 7.6 wt pct), light weight, and low cost. However, because of its high thermodynamic stability (ΔH = − 75 kJ/mol), high desorption temperature [more than 673 K (400 °C)], and very slow reaction kinetics, its practical uses are hindered.[3-6] Some methods like ball milling and adding catalysts such as transition metals, rare earth metals, and intermetallic compounds have been used to improve hydrogen storage properties of Mg.[7-9] It was reported that high energy milling can reduce diffusion paths because of reducing particle size and increasing specific surface area. Therefore, hydrogen can be easily absorbed and desorbed from milled metal hydrides.[10] Zaluski et al.[11] reported positive effect of rare earth metals on hydrogen storage properties of magnesium hydride. Rare earth elements are well-known oxygen getters. Their presence may reduce formation of surface oxides and hence benefit hydrogen absorption. Moreover, it was reported that MgNi4Y intermetallic had a positive effect on hydrogen desorption properties of MgH2.[12] Thus, in this research, simultaneous effect of adding catalyst and ball milling on hydrogen desorption properties of magnesium hydride was studied. In this regard, MgNi4Y intermetallic was synthesized via mechanical alloying and heat treatment, then added to MgH2 and ball milled for various times. Subsequently, hydrogen desorption of various milled mixtures was investigated.
Experiment
Table I shows purity and particle size of the raw materials used in this study.
High energy planetary ball mill (Asia Sanat Rakhsh 2400) was used to mill Mg, Ni, and Y powder mixture for 5 to 50 hours under argon atmosphere at room temperature. Hardened steel vial containing hardened steel balls of 10, 14, and 18 mm diameter with ball to powder weight ratio of 20:1 was used. Different ball sizes have been sometimes mixed to randomize motion of balls.[13] The ball milled powders were cold pressed to small pills (11 mm in diameter) under 350 MPa pressure by hydraulic pressing. Then, the pills were heat treated at 1073 K (800 °C) for 4 hours under flowing argon atmosphere inside a tube furnace. X-ray diffraction method (Philips X’pert pro diffractometer) with Cu-kα radiation and field emission scanning electron microscopy was used to evaluate structure of the milled and heat-treated powders. Clemex software was used to measure particle and agglomerate size of the milled powders. The crystallite size and lattice strain of ball milled powders, after modifying the instrumental broadening, were measured according to Williamson-Hall method[14] and crystallite size of the heat-treated powders was measured using Scherrer method.[15]
In the next step, 10 wt pct synthesized catalyst was added to MgH2 and then milled for 5 to 65 hours under argon atmosphere. Pure MgH2 was also ball milled in the same condition. Scanning electron microscopy, transmission electron microscopy (TEM), and X-ray diffraction methods were applied to characterize the milled powders. In order to measure the amount of released hydrogen, desorption test was applied via Sievert (Volumetric Chemisorption Manifold) method. In this regard, 50 mg of each sample was placed at 623 K (350 °C) under 0.1 bar of hydrogen atmosphere and pressure changes were recorded.
Results and Discussion
XRD patterns of Mg, Ni, and Y powder mixtures that were ball milled for several times and then heat treated at 1073 K (800 °C) can be seen in Figure 1. It is observed that MgNi4Y intermetallic could be formed after ball milling for 10 hours and then heat treating for 4 hours at 1073 K (800 °C). Also, other binary intermetallic compounds such as Ni5Y could be formed in this condition. Crystallite size of Ni in these samples was calculated via Scherrer method, as given in Table II. It can be seen that, after 5 hours of milling, with increasing milling time, crystallite size of Ni was increased (during heat treatment). This phenomenon was also observed in (Reference 12). It seems that, with increasing milling time, the stored energy in powder mixtures was increased. The higher stored energy in powder, the lower the temperature of energy release, and probably growth of starting crystallites would be occurred. Thus, size of the crystallites, after 4 hours of heat treating at 1073 K (800 °C), was increased with increment in milling time.
In order to investigate the effect of initial milling time on catalytic effect of intermetallic compound, the samples that were ball milled for 10 and 50 hours and then heat treated at 1073 K (800 °C) were selected as catalyst 1 and 2, respectively.
In the next step, 10 wt pct synthesized catalyst was added to MgH2 and then ball milled from 10 to 65 hours to investigate simultaneous effect of ball milling and adding catalyst on hydrogen desorption properties of MgH2.
Hydrogen desorption curves of the ball milled pure MgH2 at 623 K (350 °C) are shown in Figure 2. It could be concluded that as-received MgH2 at 623 K (350 °C) did not release any hydrogen. But, with increasing milling time, the amount of desorbed hydrogen was increased.
According to Huot et al.’s[10] report, this phenomenon can be explained by the defects introduced during ball milling, small particle size and increased specific surface area, which increased nucleation site density and reduced diffusion lengths.
Decreasing particle size and increasing specific surface area of MgH2 over milling time from 5 to 30 hours led to 25 pct increase in the amount of released hydrogen from MgH2. After 40 minutes, 30 hours milled MgH2 powder only released about 1 wt pct hydrogen at 623 K (350 °C), which was a very low amount. According to Table I, particle size of MgH2 was greater than 140 µm. After 40 hours of milling it decreased, significantly. In similar research, Pourabdoli et al.[16] reported that particle size or agglomerate size of MgH2 after 20 hours of milling reached 50 to 1000 nm and the BET specific surface area of MgH2 increased by factor of 8 in similar milling condition. It can be seen in Figure 2 that after ball milling, despite of decreasing in particle size of MgH2 and accumulation of energy in the crystallites, remarkable increase in the amount and kinetics of hydrogen desorption could not be observed.
In order to increase the amount of released hydrogen, it was necessary to add catalyst to MgH2. In this regard, catalysts 1 and 2 were added to MgH2 and ball milled for various times. Figures 3 and 4 depict desorption curves of MgH2 and 10 wt pct catalyst 1 and 2 mixtures in the same conditions, respectively. These curves express that adding catalyst and ball milling the mixture could greatly enhance the amount of released hydrogen.
Pure MgH2 that was ball milled for 10 hours only released 0.8 wt pct hydrogen at 623 K (350 °C) after 40 minutes, which was about 10 pct of the theoretical hydrogen amount of MgH2; but, by adding 10 wt pct catalyst 1 in the same condition, it can be observed that 3.5 wt pct hydrogen could be released from MgH2, which was 51 pct of the theoretical hydrogen amount of MgH2 + 10 wt pct catalyst 1 mixture. Also, after 65 hours ball milling of the MgH2 and 10 wt pct catalyst 1 mixture, 86 pct of theoretical amount of hydrogen could be released. Therefore, it could be concluded that synthesized MgNi4Y intermetallic had a positive effect on hydrogen desorption properties of MgH2. Moreover, with increasing milling time, the amount of released hydrogen could be enhanced.
Figures 5 and 6 display FESEM micrographs of MgH2 and 10 wt pct catalyst 1 and 2 mixtures, respectively, which was ball milled for various times. Also, results of calculating particle and agglomerate size of these samples are given in Table III. It was observed that, with increasing milling time, particle and agglomerate size of the samples were reduced. Therefore, it could be concluded that ball milling reduced particle size and enhanced specific surface area which led to reduction in diffusion path and subsequently easy desorption of hydrogen. TEM images of MgH2 and 10 wt pct catalyst 1 and 2 mixtures that were ball milled for 65 hours are shown in Figure 7. Average particle size of the mixtures was more than 200 nm; but, there were some nanoparticles in the mixtures which could improve hydrogen desorption properties of MgH2. It is reported that particle size of MgH2 + 10 wt pct MgNi4Y after 5 hours of ball milling was about 330 nm which was slightly lower than that of MgH2 alone (364 nm) in the same milling condition.[17] Similar results of Pourabdoli and his coworkers[16] showed that particle size of MgH2-10 wt pct 9Ni-2Mg-Y powder was almost equal to that of MgH2 after 20 hours of milling in similar milling condition. However, BET specific surface area of 20 hours milled MgH2 was less than that of for 20 hours milled MgH2-10 wt pct 9Ni-2Mg-Y. It seems that the hard and brittle nature of 9Ni-2Mg-Y catalyst was responsible for increasing the specific surface area. Also, the catalyst particles could act as hard tiny balls that mill the MgH2 particles and caused to breaking the MgH2 particles into smaller ones during ball milling process.[18]
Comparison of Figures 2, 3, and 4 revealed that adding catalyst to MgH2 and ball milling of mixture simultaneously could extremely improve hydrogen desorption properties of MgH2. This phenomenon could be related to the catalytic effect of synthesized catalyst. It can be seen in Figure 1 that decreasing particle size by ball milling had not significant effect on the hydrogen desorption process. Therefore, it could be concluded that adding synthesized catalyst to MgH2 and subsequent ball milling had minor effect on particle size of MgH2 but the catalytic effect of synthesized compound led to improve in the amount and kinetics of hydrogen desorption from MgH2.
It is obvious in Figures 3 and 4 that, with increasing milling time, the amount of released hydrogen was increased; but, after 50 hours, this amount was not significant. Thus, in order to prevent oxidation and Fe contamination, it is better to use 50 hours of milling time, instead of 65 hours.
Figures 3 and 4 also show that catalysts 1 and 2 almost had the same effect on hydrogen desorption properties of MgH2. Figure 8 depicts the results of TGA of MgH2 and catalysts milled for 65 hours. These results also demonstrated that catalysts 1 and 2 had almost the same effect on hydrogen desorption temperature and amount of released hydrogen. Therefore, catalyst 1 could be used instead of catalyst 2 because of lower milling time. It could be concluded that initial ball milling of Mg-Ni-Y mixture for 50 hours is not necessary and, in order to reduce oxidation and impurity, it is better to use 10 hours for ball milling of Mg-Ni-Y mixture.
According to the above findings, addition of 10 wt pct catalyst 1 to MgH2 and ball milling of mixture for 50 hours could be used for improving hydrogen desorption properties of MgH2.
Various researchers studied the effect of catalysts on improvement of hydrogen desorption from MgH2. Their results showed that it is possible to decrease the onset temperature of hydrogen desorption to below 523 K (250 °C) using different catalysts.[19-23] For example, Quyang et al.[24] demonstrated enhanced hydrogen storage kinetics MgH2 by adding CeH2.73-Ni catalyst. They showed that the temperature for the full hydrogenation/dehydrogenation cycle of the composites could be decreased to 505 K (232 °C). Present study also introduced another catalysts which can decrease the onset temperature of hydrogen desorption of MgH2 to below 523 K (250 °C).
Conclusions
In this study, simultaneous effect of adding catalyst and ball milling on hydrogen desorption properties of MgH2 was investigated. In this regard, MgNi4Y intermetallic that was synthesized via ball milling for 10 and 50 hours and then heat treating at 1073 K (800 °C) for 4 hours was used as catalysts 1 and 2, respectively. 10 wt pct of these catalysts was added to MgH2 and the mixtures were ball milled from 10 to 65 hours. The results showed that
-
1.
As-received MgH2 at 623 K (350 °C) did not release any significant amount of hydrogen.
-
2.
Decreasing particle size of MgH2 with increasing milling time from 5 to 30 hours led to 25 pct increase in the amount of released hydrogen from MgH2.
-
3.
Adding nanostructured MgNi4Y intermetallic to MgH2 and ball milling the mixture for various times resulted in increment in the amount of released hydrogen from 10 pct to 85 pct of the theoretical hydrogen content value.
-
4.
Catalysts 1 and 2 had almost the same effect on hydrogen desorption properties of MgH2.
-
5.
The best condition for improving hydrogen desorption properties of MgH2 was adding 10 wt pct catalyst 1 and ball milling of the sample for 50 hours. In this situation, 73 pct of the theoretical hydrogen content of MgH2 and catalyst mixture could be released.
References
M. Dornheim: Thermodynamics - Interaction Studies - Solids, Liquids and Gases, InTech, Germany, 2011, pp. 891-923
C.X. Shang, M. Bououdina, Y. Song, and Z.X. Guo: Int. J. Hydrogen Energy, 2004, vol. 29, pp.73-80
A. Zaluska, L. Zaluski, and J.O. Strom-Olsen: J. Alloys Compd., 1999, vol. 288, pp. 217-25
H. Huang, K. Huang, S. Liu, and D. Chen: Powder Technol., 2010, vol. 198, pp. 144-48
B. Sakintuna, B. Weinbergerb, F. Lamari-Darkrim, M. Hirscher, and B. Dogan: Int. J. Hydrog. Energy, 2007, vol. 32, pp. 1121–40
M. Dornheim, S. Doppiu, G. Barkhordarian, U. Boesenberg, T. Klassen, O. Gutfleisch, and R. Bormann: Scripta Mater., 2007, vol. 56, pp. 841-46
C.X. Shang and Z.X. Guo: Int. J. Hydrog. Energy, 2007, vol. 32, pp. 2920-25
H. Simchi, A. Kaflou, and A. Simchi: Powder Metall., 2011, vol. 54, pp. 480-83
W.N. Yang, C.X. Shang, and Z.X. Guo: Int. J. Hydrog. Energy, 2010, vol. 35, pp. 4534-42
J. Huot, G. Liang, S. Boily, A. Neste, and R. Schulz: J. Alloy Compd., 1999, vol. 293-295, pp. 495–500
L. Zaluski, A. Zaluska, and J.O. Ström-Olsen: J. Alloy Compd., 1997, vol. 253–254, pp. 70-79
K. Aono, S. Orimo, and H. Fujii: J. Alloy Compd. 2000, vol. 309, pp. L1-L4
L. Takacs: Prog. Mater Sci., 2002, vol. 47, pp. 355-414
G.K. Williamson and W.H. Hall: Acta Metall., 1953, vol. 1, pp. 21-31
B.D. Cullity: Elements of X-ray Diffraction, 2nd ed., Addison-Wesley, USA, 1978
M. Pourabdoli, Sh. Raygan, H. Abdizadeh, and D. Uner: Int. J. Hydrogen Energy, 2013, vol. 38, pp. 11910-19
L. Chitsazkhoyi, Sh. Raygan, and M. Pourabdoli: Int. J. Hydrogen Energy, 2013, vol. 38, pp. 6687-93
A. Ranjbar, Z.P. Guo, X.B. Yu, D. Wexler, A. Calka, C.J. Kim, and H.K. Liu: Mater. Chem. Phys., 2009, vol. 114, pp. 168-72
C. Stan, E. Andronescu, D. Predoi, and J. L. Bobet: J. Alloy Compd., 2008, vol. 461, pp. 228-34
W. Oelerich, T. Klassen, and T. Bormann: J. Alloy Compd., 2001, vol. 315, pp. 237-42
M. Song, L. Bobet, and B. Darriet: J. Alloy Compd., 2002, vol. 340, pp. 256-62
D. Kyoi, T. Sato, E. Ronnebro, N. Kitamura, A. Ueda, and M. Ito: J. Alloy Compd., 2004, vol. 372, pp. 213-17
G. Liang, J. Huot, S. Boily, and R. Schulz: J. Alloy Compd., 1998, vol. 305, pp. 239–45
L.Z. Ouyang, X.S. Yang, M. Zhu, J.W. Liu, H.W. Dong, D.L. Sun, J. Zou, and X.D. Yao: J. Phys. Chem. C, 2014, vol. 118, pp. 7808–20
Author information
Authors and Affiliations
Corresponding author
Additional information
Manuscript submitted June 8, 2014.
Rights and permissions
About this article
Cite this article
ChitsazKhoyi, L., Raygan, S. & Pourabdoli, M. Effect of Synthesized MgNi4Y Catalyst on Hydrogen Desorption Properties of Milled MgH2 . Metallurgical and Materials Transactions E 2, 27–32 (2015). https://doi.org/10.1007/s40553-014-0040-7
Published:
Issue Date:
DOI: https://doi.org/10.1007/s40553-014-0040-7