Abstract
The present paper presents experimental results for the heat transfer coefficient during flow boiling of refrigerant R134a in a circular channel with internal diameter of 500 µm. The experimental database covers mass velocities ranging from 200 to 800 kg/m2 s, heat fluxes up to 100 kW/m2 and vapor qualities from 0.02 to 0.75 for a saturation temperature of 40 °C. The experimental data were parametrically analyzed and the effects of the experimental parameters (heat flux, mass velocity and vapor quality) identified. Additionally, images of two-phase flow were obtained through a high-speed camera and employed to identify the flow patterns. A flow pattern map was built and compared to prediction methods from the literature. In general, the heat transfer coefficient increased with increasing mass velocity and heat flux. The experimental data were compared against seven flow boiling predictive methods from the literature. Sun and Mishima (Int J Heat Mass Transf 52(23):5323–5329, 2009. https://doi.org/10.1016/j.ijheatmasstransfer.2009.06.041) and Kanizawa et al. (Int J Heat Mass Transf 93:566–583, 2016. https://doi.org/10.1016/j.ijheatmasstransfer.2015.09.083) methods provided the best prediction of the experimental results with only Kanizawa et al. (2016) capturing the experimental heat transfer trends.



(adapted from Aguiar and Ribatski [45])







Similar content being viewed by others
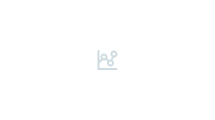
Abbreviations
- D :
-
Diameter (m)]
- f :
-
Friction factor (–)
- G :
-
Mass velocity (kg/m2 s)
- h :
-
Enthalpy (J/kg)
- I :
-
Electrical current (A)
- L :
-
Length (m)
- \(\dot{m}\) :
-
Mass flow rate (kg/s)
- Nu:
-
Nusselt number (dimensionless)
- \(\dot{q}\) :
-
Heat flux (W/m2)
- p :
-
Pressure (Pa)
- \(\dot{P}\) :
-
Electric power (W)
- Re:
-
Reynolds number (dimensionless)
- T :
-
Temperature (°C)
- V :
-
Voltage (V)
- x :
-
Vapor quality (–)
- α :
-
Heat transfer coefficient (W/m2 K)
- λ :
-
Empirical coefficient (dimensionless)
- ρ :
-
Density (kg m−3)
- σ :
-
Heat losses (dimensionless)
- \(\Delta p\) :
-
Pressure drop (Pa)
- ω :
-
Empirical coefficient (dimensionless)
- \(1\emptyset\) :
-
Single-phase
- \(2\emptyset\) :
-
Two-phase
- diff:
-
Differential (Pressure)
- f:
-
Fluid
- fric:
-
Frictional
- GO:
-
Two-phase mixture as gas
- \(i\) :
-
Discrete index position
- Int:
-
Internal
- liq:
-
Liquid
- LO:
-
Two-phase mixture as liquid
- out:
-
Outlet
- pre:
-
Preheater
- sat:
-
Saturation
- tc:
-
Thermocouple
- ts:
-
Test section
References
Sempértegui-Tapia DF, Ribatski G (2017) Two-phase frictional pressure drop in horizontal micro-scale channels: experimental data analysis and prediction method development. Int J Refrig 79:143–163. https://doi.org/10.1016/j.ijrefrig.2017.03.024
Do Nascimento FJ, Leão HLSL, Ribatski G (2013) An experimental study on flow boiling heat transfer of R134a in a micro channel-based heat sink. Exp Therm Fluid Sci 45:117–127. https://doi.org/10.1016/j.expthermflusci.2012.10.014
Cheng L, Xia G (2017) Fundamental issues, mechanisms and models of flow boiling heat transfer in microscale channels. Int J Heat Mass Transf 108:97–127. https://doi.org/10.1016/j.ijheatmasstransfer.2016.12.003
Thome JR (2006) The new frontier in heat transfer: microscale and nanoscale technologies. Heat Tranf Eng. https://doi.org/10.1080/01457630600845283
Mudawar I (2013) Recent advances in high-flux, two-phase thermal management. J Therm Sci Eng Appl 5(2):021012. https://doi.org/10.1115/1.4023599
Chauhan A, Kandlikar SG (2019) Characterization of a dual taper thermosiphon loop for CPU cooling in data centers. Appl Therm Eng 146:450–458. https://doi.org/10.1016/j.applthermaleng.2018.10.010
Sempértegui Tapia DF (2017) Análise experimental do efeito da geometria da seção transversal e do desempenho de fluidos de reduzido GWP na ebulição convectiva em canais de dimensões reduzidas. Doctoral dissertation, Universidade de São Paulo. https://doi.org/10.11606/t.18.2017.tde-02022017-152135
Agostini B, Fabbri M, Park JE, Wojtan L, Thome JR, Michel B (2007) State of the art of high heat flux cooling technologies. Heat Transf Eng 28(4):258–281. https://doi.org/10.1080/01457630601117799
Tibiriçá CB, Ribatski G (2014) Flow patterns and bubble departure fundamental characteristics during flow boiling in microscale channels. Exp Therm Fluid Sci 59:152–165. https://doi.org/10.1016/j.expthermflusci.2014.02.017
Consolini L, Ribatski G, Thome JR, Zhang W, Xu J (2007) Heat transfer in confined forced-flow boiling. Heat Transf Eng 28(10):826–833. https://doi.org/10.1080/01457630701378226
Tibirica CB, Czelusniak LE, Ribatski G (2015) Critical heat flux in a 0.38 mm microchannel and actions for suppression of flow boiling instabilities. Exp Therm Fluid Sci 67:48–56. https://doi.org/10.1016/j.expthermflusci.2015.02.020
Tibiriçá CB, Ribatski G (2010) Flow boiling heat transfer of R134a and R245fa in a 2.3 mm tube. Int J Heat Mass Transf 53(11–12):2459–2468. https://doi.org/10.1016/j.ijheatmasstransfer.2010.01.038
Lowdermilk WH, Lanzo CD, Siegel BL (1958) Investigation of boiling burnout and flow stability for water flowing in tubes. National Advisory Committee for Aeronautics
Szczukiewicz S, Borhani N, Thome JR (2013) Two-phase heat transfer and high-speed visualization of refrigerant flows in 100 × 100 μm2 silicon multi-microchannels. Int J Refrig 36(2):402–413. https://doi.org/10.1016/j.ijrefrig.2012.11.014
Consolini L (2008) Convective boiling heat transfer in a single micro-channel. Doctoral thesis. Lausanne, EPFL, Switzerland. https://doi.org/10.5075/epfl-thesis-4024
Kandlikar SG, Willistein DA, Borrelli J (2005) Experimental evaluation of pressure drop elements and fabricated nucleation sites for stabilizing flow boiling in minichannels and microchannels. In: ASME 3rd international conference on microchannels and minichannels. American Society of Mechanical Engineers Digital Collection. pp 115–124. https://doi.org/10.1115/icmm2005-75197
Tibirica CB, Ribatski G (2013) Flow boiling in micro-scale channels–synthesized literature review. Int J Refrig 36(2):301–324. https://doi.org/10.1016/j.ijrefrig.2012.11.019
Kanizawa FT, Tibiriçá CB, Ribatski G (2016) Heat transfer during convective boiling inside microchannels. Int J Heat Mass Transf 93:566–583. https://doi.org/10.1016/j.ijheatmasstransfer.2015.09.083
Tran TN, Wambsganss MW, France DM (1996) Small circular-and rectangular channel boiling with two refrigerants. Int J Multiph Flow 22(3):485–498. https://doi.org/10.1016/0301-9322(96)00002-X
Sun L, Mishima K (2009) An evaluation of prediction methods for saturated flow boiling heat transfer in mini-channels. Int J Heat Mass Transf 52(23):5323–5329. https://doi.org/10.1016/j.ijheatmasstransfer.2009.06.041
Li W, Wu Z (2010) A general correlation for evaporative heat transfer in micro/minichannels. Int J Heat Mass Transf 53(9):1778–1787. https://doi.org/10.1016/j.ijheatmasstransfer.2009.12.059
Kim SM, Mudawar I (2013) Universal approach to predicting saturated flow boiling heat transfer in mini/micro-channels–Part II. Two-phase heat transfer coefficient. Int J Heat Mass Transf 64:1239–1256. https://doi.org/10.1016/j.ijheatmasstransfer.2013.04.014
Thome JR, Dupont V, Jacobi AM (2004) Heat transfer model for evaporation in microchannels. Part I: presentation of the model. Int J Heat Mass Transf 47(14–16):3375–3385. https://doi.org/10.1016/j.ijheatmasstransfer.2004.01.006
Costa-Patry E, Thome JR (2013) Flow pattern-based flow boiling heat transfer model for microchannels. Int J Refrig 36(2):414–420. https://doi.org/10.1016/j.ijrefrig.2012.12.006
Chen JC (1966) Correlation for boiling heat transfer to saturated fluids in convective flow. Ind Eng Chem Process Des Dev 5(3):322–329
Churchill SW, Usagi R (1972) A general expression for the correlation of rates of transfer and other phenomena. AIChE J 18(6):1121–1128. https://doi.org/10.1002/aic.690180606
Stephan K, Abdelsalam M (1980) Heat-transfer correlations for natural convection boiling. Int J Heat Mass Transf 23(1):73–87. https://doi.org/10.1016/0017-9310(80)90140-4
Dittus FW, Boelter LMK (1930) University of California publications on engineering, vol 2. University of California publications in Engineering, Berkeley, p 371
Cioncolini A, Thome JR (2011) Algebraic turbulence modeling in adiabatic and evaporating annular two-phase flow. Int J Heat Fluid Flow 32(4):805–817. https://doi.org/10.1016/j.ijheatfluidflow.2011.05.006
Magnini M, Thome JR (2017) An updated three-zone heat transfer model for slug flow boiling in microchannels. Int J Multiph Flow 91:296–314. https://doi.org/10.1016/j.ijmultiphaseflow.2017.01.015
Consolini L, Thome JR (2009) Micro-channel flow boiling heat transfer of R-134a, R-236fa, and R-245fa. Microfluidics Nanofluidics 6(6):731–746. https://doi.org/10.1007/s10404-008-0348-7
Basu S, Ndao S, Michna GJ, Peles Y, Jensen MK (2011) Flow boiling of R134a in circular microtubes—Part I: study of heat transfer characteristics. J Heat Transf. https://doi.org/10.1115/1.4003159
In S, Jeong S (2009) Flow boiling heat transfer characteristics of R123 and R134a in a micro-channel. Int J Multiph Flow 35(11):987–1000. https://doi.org/10.1016/j.ijmultiphaseflow.2009.07.003
Saitoh S, Daiguji H, Hihara E (2005) Effect of tube diameter on boiling heat transfer of R-134a in horizontal small-diameter tubes. Int J Heat Mass Transf 48(23–24):4973–4984. https://doi.org/10.1016/j.ijheatmasstransfer.2005.03.035
Lazarek GM, Black SH (1982) Evaporative heat transfer, pressure drop and critical heat flux in a small vertical tube with R-113. Int J Heat Mass Transf 25(7):945–960. https://doi.org/10.1016/0017-9310(82)90070-9
Kandlikar SG, Balasubramanian P (2004) An extension of the flow boiling correlation to transition, laminar, and deep laminar flows in minichannels and microchannels. Heat Transf Eng 25(3):86–93. https://doi.org/10.1080/01457630490280425
Zhang W, Hibiki T, Mishima K (2004) Correlation for flow boiling heat transfer in mini-channels. Int J Heat Mass Transf 47(26):5749–5763. https://doi.org/10.1016/j.ijheatmasstransfer.2004.07.034
Warrier GR, Dhir VK, Momoda LA (2002) Heat transfer and pressure drop in narrow rectangular channels. Exp Therm Fluid Sci 26(1):53–64. https://doi.org/10.1016/S0894-1777(02)00107-3
Liu Z, Winterton RHS (1991) A general correlation for saturated and subcooled flow boiling in tubes and annuli, based on a nucleate pool boiling equation. Int J Heat Mass Transf 34(11):2759–2766. https://doi.org/10.1016/0017-9310(91)90234-6
Bertsch SS, Groll EA, Garimella SV (2009) A composite heat transfer correlation for saturated flow boiling in small channels. Int J Heat Mass Transf 52(7–8):2110–2118. https://doi.org/10.1016/j.ijheatmasstransfer.2008.10.022
Lee J, Mudawar I (2005) Two-phase flow in high-heat-flux micro-channel heat sink for refrigeration cooling applications: Part II—heat transfer characteristics. Int J Heat Mass Transf 48(5):941–955. https://doi.org/10.1016/j.ijheatmasstransfer.2004.09.019
Tibiriçá CB, Ribatski G, Thome JR (2012) Saturated flow boiling heat transfer and critical heat flux in small horizontal flattened tubes. Int J Heat Mass Transf 55(25–26):7873–7883. https://doi.org/10.1016/j.ijheatmasstransfer.2012.08.017
Tibiriçá CB, Rocha DM, SuethJr ILS, Bochio G, Shimizu GKK, Barbosa MC, dos Santos Ferreira S (2017) A complete set of simple and optimized correlations for microchannel flow boiling and two-phase flow applications. Appl Therm Eng 126:774–795. https://doi.org/10.1016/j.applthermaleng.2017.07.161
Ribatski G, Wojtan L, Thome JR (2006) An analysis of experimental data and prediction methods for two-phase frictional pressure drop and flow boiling heat transfer in micro-scale channels. Exp Therm Fluid Sci 31(1):1–19. https://doi.org/10.1016/j.expthermflusci.2006.01.006
Aguiar GM, Ribatski G (2019) An experimental study on flow boiling in microchannels under heating pulses and a methodology for predicting the wall temperature fluctuations. Appl Therm Eng. https://doi.org/10.1016/j.applthermaleng.2019.113851
Gnielinski V (1976) New equations for heat and mass transfer in turbulent pipe and channel flow. Int Chem Eng 16(2):359–368
Churchill SW (1977) Friction-factor equation spans all fluid-flow regimes. Chem Eng 84(24):91–92
Taylor BN, Kuyatt CE (1994) Guidelines for evaluating and expressing the uncertainty of NIST measurement results. US Department of Commerce, Technology Administration, National Institute of Standards and Technology, Gaithersburg, MD, pp 1–25
Abernethy RB, Thompson JW (1973) Handbook of uncertainty in gas turbine measurements. Arnold Engineering Development Center, Arnold Air Force Station
Ong CL, Thome JR (2011) Macro-to-microchannel transition in two-phase flow: part 2–flow boiling heat transfer and critical heat flux. Exp Therm Fluid Sci 35(6):873–886. https://doi.org/10.1016/j.expthermflusci.2010.12.003
Felcar HOM, Ribatski G, Saiz-Jabardo JM (2007) A gas–liquid flow pattern predictive method for macro-and-mini-scale round channels. In: Proceedings of the 10th UK heat transfer conference. Edinburgh, Scotland
Taitel Y, Dukler AE (1976) A model for predicting flow regime transitions in horizontal and near horizontal gas–liquid flow. AIChE J 22(1):47–55. https://doi.org/10.1002/aic.690220105
Sempértegui-Tapia DF, Ribatski G (2017) Flow boiling heat transfer of R134a and low GWP refrigerants in a horizontal micro-scale channel. Int J Heat Mass Transf 108:2417–2432. https://doi.org/10.1016/j.ijheatmasstransfer.2017.01.036
Qu W, Mudawar I (2004) Measurement and correlation of critical heat flux in two-phase micro-channel heat sinks. Int J Heat Mass Transf 47(10–11):2045–2059. https://doi.org/10.1016/j.ijheatmasstransfer.2003.12.006
Acknowledgements
The authors gratefully acknowledge grants given by FAPESP (São Paulo Research Foundation of Brazil) under Contract Number 2016/09509-1, CNPq (National Counsel of Technological and Scientific Development of Brazil) under Contract Numbers 141946/2017-2 and 305673/2017-3 and CAPES (Coordination of the Improvement in Higher Level Personnel of Brazil). The technical support given to this investigation by Mr. José Roberto Bogni and Mr. Jorge Nicolau dos Santos is also appreciated and recognized.
Author information
Authors and Affiliations
Corresponding author
Additional information
Technical Editor: Jader Barbosa Jr., PhD.
Publisher's Note
Springer Nature remains neutral with regard to jurisdictional claims in published maps and institutional affiliations.
Rights and permissions
About this article
Cite this article
dos Santos Filho, E., Aguiar, G.M. & Ribatski, G. Flow boiling heat transfer of R134a in a 500 µm ID tube. J Braz. Soc. Mech. Sci. Eng. 42, 254 (2020). https://doi.org/10.1007/s40430-020-02325-2
Received:
Accepted:
Published:
DOI: https://doi.org/10.1007/s40430-020-02325-2