Abstract
First results are reported using a simple, fast, and reproducible matrix-assisted ionization (MAI) sample introduction method that provides substantial improvements relative to previously published MAI methods. The sensitivity of the new MAI methods, which requires no laser, high voltage, or nebulizing gas, is comparable to those reported for MALDI-TOF and n-ESI. High resolution full acquisition mass spectra having low chemical background are acquired from low nanoliters of solution using only a few femtomoles of analyte. The limit-of-detection for angiotensin II is less than 50 amol on an Orbitrap Exactive mass spectrometer. Analysis of peptides, including a bovine serum albumin digest, and drugs, including drugs in urine without a purification step, are reported using a 1 μL zero dead volume syringe in which only the analyte solution wetting the walls of the syringe needle is used in the analysis.

Similar content being viewed by others
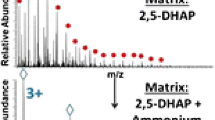
Avoid common mistakes on your manuscript.
Introduction
An important strength of mass spectrometry (MS) is the ability to characterize vanishingly small quantities of compounds residing in complex matrices. Examples include monitoring drugs and their metabolites [1, 2], and identifying proteins in biological materials [3]. Important to analyzing small quantities of analyte using MS are ionization methods to convert solid or solution phase compounds efficiently to gas-phase ions. The most commonly used ionization methods for high sensitivity analyses of either volatile or nonvolatile compounds are matrix-assisted laser desorption/ionization (MALDI) [4] and nano-electrospray ionization (n-ESI) [5–9] However, although extremely useful, vacuum MALDI requires specialized mass spectrometers and has high background chemical noise limiting detection sensitivity below mass-to-charge (m/z) of ca. 500 [10, 11]. n-ESI uses specialized ion sources with expensive consumables, is rather time consuming, and requires a high degree of operator expertise [12]. Ambient ionization approaches have been introduced over the last decade to address needs not fulfilled by traditional ionization approaches [13]. Generally, the limits of detection (LOD) for ambient ionization methods have been reported consuming nanomole to femtomole amounts of analyte [14–16]. The LOD, however, is often orders of magnitude below the amount of sample required to obtain full acquisition mass spectra in which the compound of interest is the base peak in the spectrum.
Matrix-assisted ionization (MAI) is a newly discovered ionization method for mass spectrometry [17, 18]. In MAI, sample preparation can be identical to MALDI but eliminates the need for a laser, and by using special matrix compounds reported by Trimpin and Inutan [18, 19], a heated inlet is not necessary. These matrices produce abundant ions from incorporated analyte simply by exposure to the vacuum of a mass spectrometer at the atmospheric pressure inlet aperture. A number of analytically useful MAI matrices that spontaneously produce gas-phase analyte ions without use of a laser, a high voltage source, or any external energy source have been reported [20]. The first MAI matrix discovered that does not require a heated inlet, and still one of the most useful, is 3-nitrobenzonitrile (3-NBN) [18, 19]. This matrix is employed in the studies reported herein and provides high sensitivity, especially for basic and nonvolatile compounds such as peptides and drugs [21, 22]. The charge states of ions observed using MAI are similar to electrospray ionization (ESI), allowing the use of any mass spectrometer having an atmospheric pressure inlet [20].
A number of approaches have been explored for introducing the MAI matrix:analyte sample to the inlet aperture of an atmospheric pressure mass spectrometer to initiate ionization [18, 21, 23]. Because the inlet becomes the ion source, the commercial ESI/atmospheric pressure chemical ionization (APCI) source housing is not necessary. Methods such as tapping the matrix:analyte sample applied to a glass microscope slide, filter paper, or pipette tip against the inlet aperture produce good results [17, 19]. However, these sample introduction approaches do not take advantage of the inherent sensitivity of MAI, carryover between samples is sometimes an issue, and sample introduction by hand is problematic relative to reproducibility. Herein we report reproducible sample introduction methods that nearly eliminate carryover and are significantly more sensitive than previously reported MAI methods, requiring only a few femtomoles of analyte to produce mass spectra in which the protonated molecular ions are typically the most abundant in the mass spectrum. These sample introduction methods operate on low or ultra-high resolution mass spectrometers designed for ESI or APCI.
Experimental
Materials
HPLC grade water, acetonitrile (ACN), and hydroxychloroquine sulfate, >98% purity, were purchased from Sigma Aldrich (St. Louis, MO, USA). 3-NBN, >99% purity, was purchased from Tokyo Chemical Industry (Portland, OR, USA). Angiotensin I, angiotensin II, Substance P, and bradykinin were obtained from AnaSpec Inc. (Freemont, CA, USA). Bovine serum albumin (BSA) trypsin digest was purchased from Waters (Milford, MA, USA). Lysergic acid diethylamide (LSD) was purchased from Cerilliant (Round Rock, TX, USA). A Hamilton 80100, (7001KH 1-uL SYR 25/2.75/3) 1 μL zero dead volume syringe was purchased from Hamilton (Reno, NV, USA). The 3-NBN matrix was prepared in 50:50 ACN:water at 20 mg mL–1 concentration. All chemicals were prepared in water at 100 to 300 fmol μL–1 concentrations. For the detection of LSD in urine, a standard (1000 ppm) LSD was prepared in ACN and added to urine to provide a 1-ppm solution and used without purification.
Instrumentation
The syringe-MAI experiments employed Thermo Fisher Scientific (Bremen, Germany) Exactive and Q-Exactive Orbitrap mass spectrometers. The IonMax source was removed and replaced by a MSTM (Newark, DE, USA) MAI sample introduction platform. Because the inner diameter of the inlet used in these experiments was 0.58 mm and the outer diameter of the needle from the syringe was 0.515 mm, the MSTM stage is vital in minimizing manual adjustments needed between samplings and ensuring the needle tip inserts into the inlet at the same position for each analysis. The MAI platform includes a syringe holder attached to a guide rail on a manual x, y, z stage. For the Orbitrap Exactive, the inlet temperature was set at 70 °C, and the adjustable distance at which the syringe needle inserts inside the inlet was set to 4 mm. Other parameters are capillary 60 V, tube lens 75 V, maximum trap fill time 1000 ms, skimmer 18 V, mass resolution 100,000 (50% FWHH, m/z 200), and m/z range 150–2000. All spray voltages and gases used with the ESI source were set to zero. For the Q-Exactive Orbitrap, the S-lens voltage was set to 50, the maximum trap time set to 250 ms, and the mass resolution was 70,000.
Method
Three methods for introducing matrix:analyte using a 1 μL zero dead volume syringe were studied: (Method 1) premixing the analyte and matrix in a vial before pulling the mixed solution into the syringe and expelling to air dry for ca. 30 s at the tip of the needle before being introduced to the mass spectrometer inlet; (Method 2) analyte and matrix solution are pulled into the syringe sequentially and expelled to mix and dry at the needle tip; (Method 3) analyte solution is drawn into the syringe needle and entirely dispensed back to the analyte vial followed by drawing 3-NBN matrix solution into the syringe and then expelling it to dry on the tip of the needle while the syringe is loaded onto the MSTM platform. In Method 3, the solution that is not recovered is only that which wets the wall of the zero dead volume syringe needle and was determined to be <50 nL for two tested syringes using two different methods in triplicate. In the first approach, the volume of analyte solution not recovered was estimated by weighing a microvial containing 4 μL of a water solution with 100 fmol μL–1 angiotensin II and performing 20 consecutive procedures before reweighing and dividing the weight loss by 20. The procedure involved pulling 0.5 μL of the solution into the syringe and expelling followed by an acetonitrile wash step and mild heating of the syringe needle with a heat gun to remove residual acetonitrile before the next procedure. In this method, on average, ca. 50 ng was lost per procedure. Mass spectra were obtained prior to and subsequent to the two weighing steps. In the second approach, 0.5 μL of water was pulled into and expelled from the syringe followed by touching the tip of the syringe against tissue paper to remove residual water. On average, only 17 nL of water was not recovered per procedure.
Results and Discussion
In early studies with MAI, analyte solution, usually 1 to 5 nM, was mixed with matrix solution and, typically, 1 μL, dried on a substrate, was tapped against the atmospheric pressure inlet aperture of a mass spectrometer having a heated inlet tube to obtain mass spectra [17, 24]. These sample introduction methods were also applied to revolutionary MAI matrices that do not require a heated inlet tube [18–20]. However, reproducibility of sample introduction by hand was variable, and the tapping method sometimes produced analyte carryover in subsequent acquisitions. In order to address reproducibility, a 10 μL syringe, commonly used for gas chromatography sample injection, was used to introduce a specific volume of matrix:analyte solution, after drying on the needle tip, directly into the inlet aperture of a mass spectrometer. The syringe is mechanically guided by a x, y, z-stage. Excellent reproducibility provided quantitative results similar to ESI employing internal standards [25]. Using this approach, decreasing the volume of matrix:analyte solution to a fraction of 1 μL provided nearly comparable signal-to-noise as the 1 μL injection, somewhat similar to electrospraying at low flow rates in ESI. Inserting the sample directly into the inlet had the additional advantage of practically eliminating carryover. For the studies reported here, the 10 μL syringe was replaced by a 1 μL zero dead volume syringe to better control the low volume of solution used in the analysis.
Three methods were explored for sample analysis using the 1 μL zero dead volume syringe mounted on a MSTM platform for simple and reproducible matrix:analyte introduction into the inlet aperture. Analyte ionization occurs spontaneously when the syringe needle with dry matrix:analyte is inserted into and then removed from the inlet aperture. The ion abundance lasts for a few seconds with the inlet tube of an Orbitrap Exactive at 70 °C, but the time analyte ions are formed is extended by operating the inlet at a lower temperature. Each of the methods described in the Experimental section produce exceptional results. Figure 1a shows the mass spectrum obtained by mixing equal volumes of a 3-NBN matrix solution and a 200 nM water solution of angiotensin II and pulling 200 nL of the mixed solution into the syringe before expelling to dry on the needle tip (Method 1). Because the analyte for this analysis is prepared in water and the matrix solution is 1:1 ACN:water, the expelled solution contains a high percentage of water and requires at least 30 s to dry before insertion inside the inlet tube. Even though only 200 nL of sample containing 20 fmol of analyte is required for this analysis, an extra step of mixing matrix and analyte is used. Any excess analyte in the remaining solution is contaminated with matrix making it difficult to use the sample for anything other than obtaining further mass spectral results. In practice, a minimum of about 50 fmol of sample is required using this approach.
Mass spectra obtained on an Orbitrap Exactive using 200 nM solution of angiotensin II in water and a saturated solution of 3-NBN in 1:1 ACN:water using a 1 μL dead volume syringe: (a) Method 1 using 200 nL of a 1:1 premixed matrix:analyte solution (20 fmol analyte), and (b) Method 3 using <50 nL of analyte solution (<10 fmol), both providing a S/N of ca. 2500 for the doubly charged ion
An alternative approach (Method 2) is to mix the analyte and matrix solutions inside the syringe. In this approach, a few tenths of a microliter of analyte solution is drawn into the syringe needle followed by drawing an equal volume of matrix solution. Expelling the combined solution allows it to mix and dry on the tip of the syringe needle before insertion into the inlet. Similar results as in Method 1 are obtained using the same analyte concentration when only 100 nL each of analyte (20 fmol) and matrix solution are used for the analysis (Supplementary Figure S1). The advantage of this approach is that a separate step of premixing the analyte and matrix solutions is not required, and the remaining analyte solution does not contain matrix provided it is drawn into the syringe before the matrix solution. The drying time is similar to Method 1. However, an advantage is that the volume of analyte and matrix solutions used in the analysis is operator-determined.
In Method 3, the analyte solution consumed is reduced to <50 nL and the sample drying time to the 10 to 15 s needed to mount the syringe onto the MSTM platform. In this approach, a few tenths of a microliter of the analyte solution, without mixing with matrix, is drawn into the 1 μL syringe and completely expelled back into the analyte solution followed by drawing into the same syringe a few tenths of a microliter of matrix solution and expelling to dry on the syringe needle tip. The combining of residual analyte solution with matrix solution occurs in the zero dead volume syringe needle. The drying time of the expelled solution is reduced because the percentage of water is essentially that in the matrix solution. While the volume of analyte lost in each analysis using this approach was determined to be <50 nL, there appears to be a small additional loss of the analyte. This was determined by obtaining the mass spectrum of angiotensin II in the first acquisition and again after 20 subsequent analyte removal steps from a 4 μL volume of analyte solution (see the Experimental section). While the combined ion abundances of the singly and doubly charged quasi-molecular ions of angiotensin II only decreased by ca. 35% over the 20 procedures, the background chemical noise increased substantially, which may be attributed to sample contamination from an acetonitrile wash followed by a drying step between each procedure.
Almost identical results are obtained for angiotensin II using Method 3 as was obtained for Methods 1 and 2. This simple to use approach for sample preparation and analysis is ideal for volume and concentration limited analyses. Figure 1b is the mass spectrum obtained by pulling in and fully expelling 200 nL of the same analyte solution used in Figure 1a, followed by pulling in and expelling an equal volume of 3-NBN matrix solution to dry on the syringe needle. Assuming minimal analyte loss by adsorption to the syringe needle walls, less than 10 fmol of the analyte is used in this analysis, producing a mass spectrum of angiotensin II with low chemical background. For comparison with methods reporting LOD values [21, 22], the LOD of Method 3 for angiotensin II was determined to be < 50 amol (Supplementary Figure S2). Based on these results, the sensitivity of Method 3 is similar to n-ESI, but the MAI approach is easier and faster. Improved results are obtained using Method 3 with a relatively new Q-Exactive Orbitrap mass spectrometer. The MAI mass spectrum for a 100 nM solution of angiotensin I in water is shown in the graphical abstract and Supplementary Figure S3 using 3-NBN as matrix. The amount of analyte used for the full acquisition analysis is <5 fmol. Even at this level of analyte consumed, abundant doubly and triply charge molecular ions of angiotensin I are observed with only low abundance background ions. MAI Method 3 is not limited to pure samples or simple mixtures as is shown in Figure 2 for a complex mixture of peptides in a bovine serum albumin tryptic digest (1 pmol μL–1) in which only ca. 50 nL of the solution is not recovered. The entire procedure requires less than 20 s, and the peptide molecular weights observed account for >25% of the single cleavage tryptic peptides.
Small molecules are also applicable with Method 3 as shown for different drugs. Figure 3a is of a 200 nM solution of hydroxychloroquine (HCQ) in which the 50 nL consumed contains ca. 10 fmol of HCQ. The MH+ and MH2 2+ ions, m/z 336 and m/z 168, respectively, appear well above background. As previously reported, the MAI method also works well using salty and buffered solutions [23, 25, 26]. This is demonstrated with Method 3 using a urine sample spiked with LSD to make a 1-ppm solution. Drawing 0.5 μL of the spiked urine solution into the syringe and expelling before drawing and expelling 0.5 μL of the 3-NBN matrix solution produces the mass spectrum shown in Figure 3b. Even though the urine contains a high level of salt and other compounds, the protonated molecular ion is the base peak observed at m/z 324.207 (calculated m/z 324.207). Likewise, using Method 3, a urine sample of azithromycin (MW 748) is detected as the major peak in the mass spectrum of a urine sample taken 2 d after the final dose of this medication and diluted 5× with water (Figure 3c). This drug was detectable 12 d after the final dose. The absence of sodium and potassium adducts is likely related to low ionization efficiency for metal cation adduction with 3-NBN as the matrix.
Mass spectra using the MAI syringe Method 3 with the 3-NBN matrix on the Orbitrap Exactive: (a) 200 nM solution of HCQ consuming ca.10 fmol and showing both singly and doubly charged quasi-molecular ions, (b) 1 ppm of LSD spiked in urine showing the MH+ ion as the base peak, (c) a urine sample of azithromycin 2 d after final dose diluted 5× with water showing the doubly charged ion as the base peak in the mass range 150–1125
Using the syringe method, reasonably high concentrations of analyte do not cause instrument related analyte carryover. Supplementary Figure S4a demonstrates Method 3 using a 950 μM solution of angiotensin II inserting dry matrix:analyte crystals into the inlet tube using the MSTM platform. Supplementary Figure S4b is a blank obtained directly afterwards, showing no carryover of the analyte. However, introduction of this matrix:analyte solution while still wet results in analyte carryover. To eliminate instrument-related sample carryover, similar to issues with other ionization methods, high analyte concentration solutions should be avoided. In cases where high sample concentration does cause instrument-related carryover, we have found that heating the inlet tube to ca. 400 °C and then cooling again usually eliminates the carryover even for nonvolatile analytes. This suggests that instrument carryover may be an association of matrix and analyte, and not simply analyte. Directly adding analyte to the inlet tube does not result in observation of analyte ions on introduction of matrix. Instrument contamination from the 3-NBN matrix itself is not observed, which is attributed to matrix self-cleaning through sublimation.
The most common carryover issue using the methods reported here is contamination of the syringe with analyte. This issue is sample-related with certain compounds being more susceptible to syringe contamination, possibly related to adsorption on the walls. For example, using Method 3, even a moderate concentration of HCQ (300 fmol μL–1) results in HCQ molecular ions being observed in subsequent blank acquisitions even after multiple syringe washings with, e.g., 1:1 methanol:water or methanol. It would seem that the HCQ concentration after several washings should be extremely low, making the observed carryover puzzling. That the contamination is syringe related is confirmed by using a fresh uncontaminated syringe for blank acquisitions. Fortunately, removal of the HCQ from the syringe is readily achieved by heating the syringe needle, after a single washing, using a laboratory heat gun. A heating approach applied to clean n-ESI emitters was previously reported [27].
Conclusion
New MAI sample preparation and introduction methods provide rapid and high sensitivity analysis of volatile and nonvolatile compounds. Full acquisition mass spectra in which the compounds of interest provide the base peaks are obtained consuming low nanoliters of nanomolar concentration solutions. Only a few hundred nanoliters of analyte solution is necessary for the analysis, and in one approach, all but ca. 50 nL of this solution are preserved unadulterated. For angiotensin II, the LOD on an Orbitrap Exactive is <50 amol. Using 5 fmol of the peptide angiotensin I provides a mass spectrum in which the singly, doubly, and triply charged ions are the most abundant peaks, and 50 fmol of a BSA digest allowed observation of >25% of the single cleavage tryptic peptides. Full acquisition in which the quasi-molecular ions of the drug HCQ are the base peaks in the mass spectrum requires only 10 fmol, and mass spectra of LSD and azithromycin are obtained directly from diluted urine without any sample purification steps. Data acquired at high resolution with accurate mass measurements required as little as 20 s per analysis. It is expected that the MAI approaches presented here can be further downscaled and automated.
References
Nordman, N., Sikanen, T., Moilanen, M.E., Aura, S., Kotiaho, T., Franssila, S., Kostiainen, R.: Rapid and sensitive drug metabolism studies by SU-8 microchip capillary electrophoresis-electrospray ionization mass spectrometry. J. Chromatogr. A 1218, 739–745 (2011)
Want, E.J., Cravatt, B.F., Siuzdak, G.: The expanding role of mass spectrometry in metabolite profiling and characterization. Chem. BioChem. 6, 1941–1951 (2005)
Domon, B., Aebersold, R.: Mass spectrometry and protein analysis. Science 312, 212–217 (2006)
Karas, M., Bachmann, D., Bahr, U., HillenKamp, F.: Matrix-assisted ultraviolet laser desorption of non-volatile compounds. Int. J. Mass Spectrom. Ion Process. 78, 53–68 (1987)
Wilm, M., Mann, M.: Analytical properties of the nanoelectrospray ion source. Anal. Chem. 68, 1–8 (1996)
Zhang, L.K., Gross, M.L.: Matrix-assisted laser desorption/ionization mass spectrometry methods for oligodeoxynucleotides: improvements in matrix, detection limits, quantification, and sequencing. J. Am. Soc. Mass Spectrom. 11, 854–865 (2000)
Keller, B.O., Li, L.: Detection of 25,000 molecules of Substance P by MALDI-TOF mass spectrometry and investigations into the fundamental limits of detection in MALDI. J. Am. Soc. Mass Spectrom. 12, 1055–1063 (2001)
Giorgianni, F., Cappiello, A., Beranova-Giorgianni, S., Palma, P., Trufelli, H., Desiderio, D.M.: LC-MS/MS analysis of peptides with methanol as organic modifier: improved limits of detection. Anal. Chem. 76, 7028–7038 (2004)
Walcher, W., Toll, H., Ingendoh, A., Huber, C.G.: Operational variables in high-performance liquid chromatography-electrospray ionization mass spectrometry of peptides and proteins using poly(styrene-divinylbenzene) monoliths. J. Chromatogr. A 1053, 107–117 (2004)
Krutchinsky, A.N., Chait, B.T.: On the nature of the chemical noise in MALDI mass spectra. J. Am. Soc. Mass Spectrom. 13, 129–134 (2002)
Shroff, R., Rulisek, L., Doubsky, J., Svatos, A.: Acid-base-driven matrix-assisted mass spectrometry for targeted metabolomics. Proc. Natl. Acad. Sci. U. S. A. 106, 10092–10096 (2009)
Kirby, A.E., Jebrail, M.J., Yang, H., Wheeler, A.R.: Folded emitters for nanoelectrospray ionization mass spectrometry. Rapid Commun. Mass Spectrom. 24, 3425–3431 (2010)
Venter, A., Nefliu, M., Cooks, R.G.: Ambient desorption ionization mass spectrometry. TrAC Trends Anal. Chem. 27, 284–290 (2008)
Rao, W., Celiz, A.D., Scurr, D.J., Alexander, M.R., Barrett, D.A.: Ambient DESI and LESA-MS analysis of proteins adsorbed to a biomaterial surface using in-situ surface tryptic digestion. J. Am. Soc. Mass Spectrom. 24, 1927–1936 (2013)
Santos, J.M., Vendramini, P.H., Schwab, N.V., Eberlin, M.N., de Morais, D.R.: A dopant for improved sensitivity in easy ambient sonic-spray ionization mass spectrometry. J. Mass Spectrom. 51, 53–61 (2016)
McEwen, C.N.: Atmospheric-pressure solids analysis probe (ASAP). Encycl. Anal. Chem. (2010). doi:10.1002/9780470027318.a9045
McEwen, C.N., Pagnotti, V., Inutan, E.D., Trimpin, S.: New paradigm in ionization: multiply charged ion formation from a solid matrix without a laser or voltage. Anal. Chem. 82, 9164–9168 (2010)
Trimpin, S., Inutan, E.D.: Matrix assisted ionization in vacuum, a sensitive and widely applicable ionization method for mass spectrometry. J. Am. Soc. Mass Spectrom. 24, 722–732 (2013)
Inutan, E.D., Trimpin, S.: Matrix assisted ionization vacuum (MAIV), a new ionization method for biological materials analysis using mass spectrometry. Mol. Cell. Proteomics 12, 792–796 (2013)
Trimpin, S., Lutomski, C.A., El-Baba, T.J., Woodall, D.W., Foley, C.D., Manly, C.D., Wang, B., Liu, C.W., Harless, B.M., Kumar, R., Imperial, L.F., Inutan, E.D.: Magic matrices for ionization in mass spectrometry. Int. J. Mass Spectrom. 377, 532–545 (2015)
Chubatyi, N.D., McEwen, C.N.: Improving the sensitivity of matrix-assisted ionization (MAI) mass spectrometry using ammonium salts. J. Am. Soc. Mass Spectrom. 26, 1649–1656 (2015)
El-Baba, T.J., Lutomski, C.A., Wang, B., Trimpin, S.: Characterizing synthetic polymers and additives using new ionization methods for mass spectrometry. Rapid Commun. Mass Spectrom. 28, 1175–1184 (2014)
Woodall, D.W., Wang, B., Inutan, E.D., Narayan, S.B., Trimpin, S.: High-throughput characterization of small and large molecules using only a matrix and the vacuum of a mass spectrometer. Anal. Chem. 87, 4667–4674 (2015)
Lietz, C.B., Richards, A.L., Ren, Y., Trimpin, S.: Inlet ionization: protein analyses from the solid state without the use of a voltage or a laser producing up to 67 charges on the 66 kDa BSA protein. Rapid Commun. Mass Spectrom. 25, 3453–3456 (2011)
Chakrabarty, S., DeLeeuw, J.L., Woodall, D.W., Jooss, K., Narayan, S.B., Trimpin, S.: Reproducibility and quantification of illicit drugs using matrix-assisted ionization (MAI) mass spectrometry. Anal. Chem. 87, 8301–8306 (2015)
Inutan, E.D., Wager-Miller, J., Narayan, S.B., Mackie, K., Trimpin, S.: The potential for clinical applications using a new ionization method combined with ion mobility spectrometry-mass spectrometry. Int. J. Ion Mobil. Spectrom. 16, 145–159 (2013)
Pan, N., Rao, W., Kothapalli, N.R., Liu, R., Burgett, A.W., Yang, Z.: The single-probe: a miniaturized multifunctional device for single cell mass spectrometry analysis. Anal. Chem. 86, 9376–9380 (2014)
Acknowledgments
Financial support for this work by the Richard Houghton endowment from the University of the Sciences (C.N.M.), NSF STTR Phase I grant 1417124 [MSTM], the National Institutes of Health grant number S10 OD016267-01 for purchase of the Q-Exactive (M.V.J.), and the National Institute of General Medical Sciences grant number 1 P30 GM110758-01 for operational support of the Q-Exactive (M.V.J.) are gratefully acknowledged. The authors thank Dr. Sarah Trimpin (Wayne State University) for helpful discussions during the preparation of this manuscript. All opinions expressed are those of the authors.
Author information
Authors and Affiliations
Corresponding author
Electronic Supplementary Material
Below is the link to the electronic supplementary material.
ESM 1
(DOCX 153 kb)
Rights and permissions
About this article
Cite this article
Hoang, K., Pophristic, M., Horan, A.J. et al. High Sensitivity Analysis of Nanoliter Volumes of Volatile and Nonvolatile Compounds using Matrix Assisted Ionization (MAI) Mass Spectrometry. J. Am. Soc. Mass Spectrom. 27, 1590–1596 (2016). https://doi.org/10.1007/s13361-016-1433-x
Received:
Revised:
Accepted:
Published:
Issue Date:
DOI: https://doi.org/10.1007/s13361-016-1433-x