Abstract
The surface waters of Tianjin municipality exhibit severe nitrogen loading and salinization; however, the extent to which the current levels of nitrogen enrichment and salinization have impacted belowground biomass and root morphology in reed marshes is poorly documented. This study was conducted at typical saline reed marshes (Dahuangpu, Qilihai, and Beidagang) in the Tianjin municipality. The relationships between belowground biomass, belowground-aboveground biomass (B-A) ratio, root morphology and the varying sediment nitrogen and salinity levels were investigated. Results showed: 1) Higher salinity levels related to a substantial decrease in the belowground biomass, B-A ratio, and root length density; 2) Higher nitrogen stress levels related to decreased belowground biomass, specific root length and root length density, and B-A ratio at Qilihai site with the highest nitrogen loading; 3) specific root length, root length density, and B-A ratio were significantly correlated to the aboveground plant nitrogen concentration; and 4) neither salinity nor nitrogen stress significantly altered aboveground biomass. The results show that aboveground biomass invariance masks significant belowground productivity changes in response to salinization and nitrogen loading in Reed Marshes. Impact of salinization and nitrogen loading on belowground productivity in wetlands should be considered to fine-tune critical carbon-dependent components of global change models.







Similar content being viewed by others
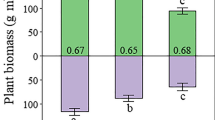
References
Asaeda T, Manatunge J, Fujino T, Sovira D (2003) Effects of salinity and cutting on the development of Phragmites australis. Wetlands Ecology and Management 11:127–140
Barter M, Li Z, Xu J (2001) Shorebird numbers on the Tianjin municipality coast in may 2000. The Stilt 39:2–9
Bastlová D, Čížková H, Bastl M, Květ J (2004) Growth of Lythrum salicaria and Phragmites australis plants originating from a wide geographical area: response to nutrient and water supply. Global Ecology and Biogeography 13:259–271. doi:10.1111/j.1466-822X.2004.00089.x
Berendse F, Möller F (2009) Effects of competition on root-shoot allocation in Plantago lanceolata L.: adaptive plasticity or ontogenetic drift? Plant Ecology 201:567–573. doi:10.1007/978-90-481-2798-6_16
Bernstein N, Meiri A, Zilberstaine M (2004) Root growth of avocado is more sensitive to salinity than shoot growth. Journal of the American Society Horticultural Science 129:188–192
Bertness MD, Ewanchuk PJ, Silliman BR (2002) Anthropogenic modification of New England salt marsh landscapes. Proceedings of the National Academy of Sciences 99:1395–1398. doi:10.1073/pnas.022447299
Chen Q, Liu D, Ma C, Wang Z (2016) Productivity and N and P nutrition of the Phragmites australis community in typical wetland in Tianjin and their relationships with environmental factors. Journal of ecology and rural enviroment 32:60–67
Cheng Y, Han Y, Wang Q, Wang Z (2004) Seasonal dynamics of fine root biomass, root length density, specific root length and soil resource availability in a Larix gmelinii plantation. Acta Phytoecological Sinica 1:310–317. doi:10.1007/ s11515-006-0039-2
Colmer T (2003) Long-distance transport of gases in plants: a perspective on internal aeration and radial oxygen loss from roots. Plant, Cell & Environment 26:17–36. doi:10.1046/j.1365-3040.2003.00846.x
Darby FA, Turner RE (2008a) Below-and aboveground biomass of Spartina alterniflora: response to nutrient addition in a Louisiana salt marsh. Estuaries and Coasts 31:326–334. doi:10.1007/s12237-008-9037-8
Darby FA, Turner RE (2008b) Effects of eutrophication on salt marsh root and rhizome biomass accumulation. Marine Ecology Progress Series 363:63–70. doi:10.3354/meps07423
Davey E, Wigand C, Johnson R, Sundberg K, Morris J, Roman CT (2011) Use of computed tomography imaging for quantifying coarse roots, rhizomes, peat, and particle densities in marsh soils. Ecological Applications 21:2156–2171. doi:10.1890/10–2037.1
Ding X, Tian C, Zhang S, Song J, Zhang F, Mi G, Feng G (2010) Effects of NO3−-N on the growth and salinity tolerance of Tamarix Laxa Willd. Plant and Soil 331:57–67. doi:10.1007/s11104-009-0231-7
Engloner AI (2009) Structure, growth dynamics and biomass of reed (Phragmites australis)–a review. Flora-Morphology, Distribution, Functional Ecology of Plants 204:331–346. doi:10.1016/j.flora.2008.05.001
Feng XP, Wang YD, Chen Q, Guo CC, Wang ZZ (2014) Research on the spatial evolvement regulations of soil salt of the coastal natural wetlands in Tianjin. Journal of Tianjin Normal University (Natural Science Edition) 34:41–48
Galvan-Ampudia CS, Testerink C (2011) Salt stress signals shape the plant root. Current Opinion in Plant Biology 14:296–302. doi:10.1016/j.pbi.2011.03.019
Gao Y, Giese M, Lin S, Sattelmacher B, Zhao Y, Brueck H (2008) Belowground net primary productivity and biomass allocation of a grassland in Inner Mongolia is affected by grazing intensity. Plant and Soil 307:41–50. doi:10.1007/s11104-008-9579-3
Guo W, Wang R, Zhou S, Zhang S, Zhang Z (2003) Genetic diversity and clonal structure of Phragmites australis in the Yellow River delta of China. Biochemical Systematics and Ecology 31:1093–1109. doi:10.1016/S0305-1978 (03) 00032-2
Güsewell S, Koerselman W (2002) Variation in nitrogen and phosphorus concentrations of wetland plants. Perspectives in Plant Ecology, Evolution and Systematics 5:37–61. doi:10.1078/1433-8319-0000022
Hilbert DW (1990) Optimization of plant root: shoot ratios and internal nitrogen concentration. Annals of Botany 66:91–99
Janousek CN, Mayo C (2013) Plant responses to increased inundation and salt exposure: interactive effects on tidal marsh productivity. Plant Ecology 214:917–928. doi:10.1007/s11258-013-0218-6
Jiang TT, Pan JF, Pu XM, Wang B, Pan JJ (2015) Current status of coastal wetlands in China: degradation, restoration, and future management. Estuarine, Coastal and Shelf Science 164:265–275. doi:10.1016/j.ecss.2015.07.046
Kirwan ML, Guntenspergen GR (2012) Feedbacks between inundation, root production, and shoot growth in a rapidly submerging brackish marsh. Journal of Ecology 100:764–770. doi:10.1111/j.1365-2745.2012.01957.x
Koerselman W, Meuleman AF (1996) The vegetation N: P ratio: a new tool to detect the nature of nutrient limitation. Journal of applied ecology:1441-1450 doi:10.2307/2404783
Langley JA, McKee KL, Cahoon DR, Cherry JA, Megonigal JP (2009) Elevated CO2 stimulates marsh elevation gain, counterbalancing sea-level rise. Proceedings of the National Academy of Sciences 106:6182–6186
Langley JA, Mozdzer TJ, Shepard KA, Hagerty SB, Megonigal JP (2013) Tidal marsh plant responses to elevated CO2, nitrogen fertilization, and sea level rise. Global Change Biology 19:1495–1503. doi:10.1111/gcb.12147
Li F, Xie Y, Qin Y (2009) Adaptive strategies of wetland plants in salt stress environment. Chinese Journal of Ecology 28:314–321
Li J, Liu CQ, Yue F, Zhu ZZ, Zhang GP, Xiang M, Li Y (2010) Hydrochemical evidence of surface water salinization process in the Tianjin coastal plain, China. Environmental Chemisity 29:285–289
Li L, Han W, Thevs N, Jia X, Ji C, Jin D, He P, Schmitt AO, Cirella GT, Zerbe S (2014) A comparison of the functional traits of common reed (Phragmites australis) in northern China: aquatic vs. Terrestrial Ecotypes Plos One 9:e89063. doi:10.1371/journal.pone.0089063 eCollection 2014
Lissner J, Schierup HH (1997) Effects of salinity on the growth of Phragmites australis. Aquatic Botany 55:247–260. doi:10.1016/S0304-3770(96)01085-6
López-Bucio J, Cruz-Ramirez A, Herrera-Estrella L (2003) The role of nutrient availability in regulating root architecture. Current Opinion in Plant Biology 6:280–287
Lovelli S, Perniola M, Tommaso TD, Bochicchio R, Amato M (2012) Specific root length and diameter of hydroponically-grown tomato plants under salinity. Journal of Agronomy 11:101. doi:10.3923/ja.2012.101.106
Lynch JP (2007) Roots of the second green revolution. Australian Journal of Botany 55:493–512
Mauchamp A, Mésleard F (2001) Salt tolerance in Phragmites australis populations from coastal Mediterranean marshes. Aquatic Botany 70:39–52. doi:10.1016/S0304-3770(00)00140-6
Meyerson L, Saltonstall K, Windham L, Kiviat E, Findlay S (2000) A comparison of Phragmites australisin freshwater and brackish marsh environments in North America. Wetlands Ecology and Management 8:89–103
Moore GE, Burdick DM, Peter CR, Keirstead DR (2012) Belowground biomass of Phragmites australis in coastal marshes. Northeastern Naturalist 19:611–626
Olsthoorn A, Keltjens W, Van Baren B, Hopman M (1991) Influence of ammonium on fine root development and rhizosphere pH of Douglas-fir seedlings in sand. Plant and Soil 133:75–81. doi:10.1007/BF00011901
Ostonen I, Püttsepp Ü, Biel C, Alberton O, Bakker MR, Lõhmus K, Majdi H, Metcalfe D, Olsthoorn AF, Pronk A (2007) Specific root length as an indicator of environmental change. Plant Biosystems 141:426–442. doi:10.1080/11263500701626069
Palomo L, Niell FX (2009) Primary production and nutrient budgets of Sarcocornia perennis ssp. alpini (lag.) Castroviejo in the salt marsh of the Palmones River estuary (southern Spain). Aquatic Botany 91:130–136. doi:10.1016/j.aquabot. 2009.04.002
Poorter H, Nagel O (2000) The role of biomass allocation in the growth response of plants to different levels of light, CO2, nutrients and water: a quantitative review. Functional Plant Biology 27:1191–1191. doi:10.1071/PP99173
Rickey M, Anderson RC (2004) Effects of nitrogen addition on the invasive grass Phragmites australis and a native competitor Spartina pectinata. Journal of Applied Ecology 41:888–896
Roman CT, Daiber FC (1984) Aboveground and belowground primary production dynamics of two Delaware Bay tidal marshes. Bulletin of the Torrey Botanical Club:34–41. doi:10.2307/2996208
Rubinigg M, Posthumus F, Ferschke M, Elzenga JTM, Stulen I (2003) Effects of NaCl salinity on 15N-nitrate fluxes and specific root length in the halophyte Plantago maritima L. Plant and Soil 250:201–213. doi:10.1023/A:1022849928781
Saltonstall K, Court Stevenson J (2007) The effect of nutrients on seedling growth of native and introduced Phragmites australis. Aquatic Botany 86:331–336. doi:10.1016/j.aquabot.2006.12.003
Tennant D (1975) A test of a modified line intersect method of estimating root length. The Journal of Ecology 63:995–1001
Tripathee R, Schäfer K (2015) Above-and belowground biomass allocation in four dominant salt marsh species of the eastern United States. Wetlands 35:21–30. doi:10.1007/s13157-014-0589-z
Turner RE, Swenson EM, Milan CS, Lee JM, Oswald TA (2004) Below-ground biomass in healthy and impaired salt marshes. Ecological Research 19:29–35
Turner RE, Howes BL, Teal JM, Milan S, Swenson EM, Tonerb DDG (2009) Salt marshes and eutrophication: an unsustainable outcome. Limnology and Oceanography 54:1634–1642. doi:10.4319/lo.2009.54.5.1634
Van Zandt PA, Tobler MA, Mouton E, Hasenstein KH, Mopper S (2003) Positive and negative consequences of salinity stress for the growth and reproduction of the clonal plant, Iris hexagona. Journal of Ecology 91:837–846. doi:10.1046/j.1365-2745.2003.00806.x
Votrubová O, Pecháčková A (1996) Effect of nitrogen over-supply on root structure of common reed. Folia Geobotanica 31:119–125
Wang Q, Wang C, Zhao B, Ma Z, Luo Y, Chen J, Li B (2006) Effects of growing conditions on the growth of and interactions between salt marsh plants: implications for invasibility of habitats. Biological Invasions 8:1547–1560. doi:10.1007/s10530-005-5846-x
Xue D, Boeckx P, Wang Z (2014) Nitrate sources and dynamics in the salinized rivers and estuaries - a δ15N-and δ18O-NO3 − isotope approach. Biogeosciences Discussions 11:4563–4589. doi:10.5194/bgd-11-4563-2014
Yi L, Wang Z (2011) Root system characters in growth and distribution among three littoral halophytes. Acta Ecologica Sinica 31:1195–1202
Yue F, Liu X, Li J, Zhu Z, Wang Z (2010) Using nitrogen isotopic approach to identify nitrate sources in waters of Tianjin, China. Bulletin of Environmental Contamination and Toxicology 85:562–567. doi:10.1007/s00128-010-0156-0
Acknowledgements
This work was supported by the Tianjin Municipal Science and Technology Foundation (15JCQNJC08100, 15JCYBJC49200 and 14JCQNJC08800), and the Innovation Team Training plan of the Tianjin Education Committee (TD12-5037). We would like to thank Mr. Changcheng Guo and Yuntao Shang for their help with field work of the experiment. We thank Elaine Stebler, Associate Editor and two anonymous reviewers for helpful comments on previous drafts of this manuscript. We thank Michael Wine for technical editing.
Author information
Authors and Affiliations
Corresponding author
Electronic supplementary material
ESM 1
(DOC 579 kb)
Rights and permissions
About this article
Cite this article
Chen, Q., Wang, Y., Zou, C.B. et al. Aboveground Biomass Invariance Masks Significant Belowground Productivity Changes in Response to Salinization and Nitrogen Loading in Reed Marshes. Wetlands 37, 985–995 (2017). https://doi.org/10.1007/s13157-017-0932-2
Received:
Accepted:
Published:
Issue Date:
DOI: https://doi.org/10.1007/s13157-017-0932-2