Abstract
Innovation in healthcare promises unparalleled potential in optimizing the production, distribution, and use of the health workforce and infrastructure, allocating system resources more efficiently, and streamline care pathways and supply chains. A recent innovation contributing to this is robot-assisted surgeries (RAS). RAS causes less damage to the patient's body, less pain and discomfort, shorter hospital stays, quicker recovery times, smaller scars, and less risk of complications. However, introducing a robot in traditional surgeries is not straightforward and brings about new risks that conventional medical instruments did not pose before. For instance, since robots are sophisticated machines capable of acting autonomously, the surgical procedure's outcome is no longer limited to the surgeon but may also extend to the robot manufacturer and the hospital. This article explores the influence of automation on stakeholder responsibility in surgery robotization. To this end, we map how the role of different stakeholders in highly autonomous robotic surgeries is transforming, explore some of the challenges that robot manufacturers and hospital management will increasingly face as surgical procedures become more and more automated, and bring forward potential solutions to ascertain clarity in the role of stakeholders before, during, and after robot-enabled surgeries (i.e. a Robot Impact Assessment (ROBIA), a Robo-Terms framework inspired by the international trade system 'Incoterms', and a standardized adverse event reporting mechanism). In particular, we argue that with progressive robot autonomy, performance, oversight, and support will increasingly be shared between the human surgeon, the support staff, and the robot (and, by extent, the robot manufacturer), blurring the lines of who is responsible if something goes wrong. Understanding the exact role of humans in highly autonomous robotic surgeries is essential to map liability and bring certainty concerning the ascription of responsibility. We conclude that the full benefits the use of robotic innovations and solutions in surgery could bring to healthcare providers and receivers cannot be realized until there is more clarity on the division of responsibilities channeling robot autonomy and human performance, support, and oversight; a transformation on the education and training of medical staff, and betterment on the complex interplay between manufacturers, healthcare providers, and patients.
Similar content being viewed by others
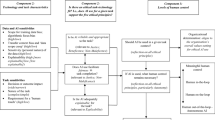
1 Introduction
The twentieth century is often characterized by technology mediated healthcare delivery, from innovations in imaging technologies to organ transplantation, heart surgery and cardiac care, to the advent of randomized control trials and surgical anesthetic and antisepsis. The market forces that are identified as driving such innovations are technological opportunity, growth in demand and growth in costs [22]. Robotics and artificial intelligence (AI) are some of the latest technological breakthroughs expected to qualitatively improve safety of care while simultaneously restraining expenditure given their success in the industrial sector [16, 75]. As a result of their reduced cost and their increased roles and capacities (COMEST 2017), healthcare robots are likely to be deployed to this end at an unprecedented rate [81] to perform medical interventions [69], support impaired patients [88], provide therapy to children [79] and keep the elderly company [8]. Among all these technological innovations, in this article we focus on surgery robots which are service robots that support surgeons during surgical procedures [7].
Since the mid-1980s, when the first robot-assisted surgical (RAS) procedures took place, surgical robotics has evolved into a highly dynamic and rapidly growing field of business and research, enjoying increased clinical attention worldwide [26], Bergeles and Yang 2013, [57]. Initially introduced for a limited type of surgical procedures, advances in ergonomics, computing power, hardware dexterity, safety, and surgery ease have immensely improved robot-assisted surgical procedures. These robots have been used for minimally invasive surgical (MIS) operations, i.e., for operations that involve the insertion of a narrow laparoscopic device into the human body instead of having to open the patient to that end [85]. The difference here is that instead of the surgeon manually operating instruments, the surgeons are supported by high-tech robotic systems' power and precision [34]. MIS is thus an example of a technology-enabled service [11] which has merged traditional surgery with innovative technologies enabling new, more efficient, and safer surgery delivery. This type of surgery causes less damage to the patient's body and is generally associated with less pain and discomfort, shorter hospital stays, quicker recovery times, smaller scars, and less risk of complications following the surgery [52]. Moreover, since robots are devoid of shortcomings such as fatigue or momentary lapses of attention, they can perform repeated and tedious surgeries, enabling at the same time, the performance of surgical procedures that were previously considered impossible. For instance, RAS could contribute to “optimize the production, distribution, and use of the health workforce and infrastructure, allocate system resources more efficiently and streamline care pathways and supply chains” in low- and middle-income countries (Reddy et al. 2016).
Despite the many clear benefits of promoting constant innovation in the field of healthcare robotics, its application in the real world presents multiple gaps that can cause harm in a way that humans cannot necessarily correct or oversee [3]. For instance, safety issues such as injury or death may arise if robot surgeons power down mid-operation or operate unintendedly [1, 27]. Moreover, as robots' perception, decision-making power, and capacity to perform a task autonomously increase, the human role and its associated responsibilities will necessarily change, and other issues relating to cybersecurity and privacy will become more significant [95]. Security vulnerabilities may allow unauthorized users to remotely access and control robots, potentially harming patients (FDA 2020), continuing to blur the discernment of who is responsible if something goes wrong [36]. Given the strong human–machine interplay and the involvement of a wide variety of stakeholders in robotic surgery, determining which stakeholder is liable for particular harm is incredibly challenging for risks that arise from the composition of, and interactions between, components managed by different entities, rather than from a single entity failing to act competently [37]. Each of these aspects challenges the innovation management capability, i.e., the ways in which innovation is organized and managed [39] by the different stakeholders to assure that the introduction of such innovation is truly improving healthcare delivery.
This article explores the influence of automation on stakeholder responsibility in surgery innovation. Our main research question is: how does automation affect stakeholder responsibility in surgery robotization? We explore how the role of different stakeholders in the highly complex robotic surgeries’ ecosystem is transforming due to the increasing levels of robot automation, and investigate some of the challenges that robot manufacturers and hospital management will likely face as surgical procedures become increasingly automated. We identify some gaps in the existing innovation management approaches and regulations when it comes to the application of robots in the surgical environment and the identification of the roles and responsibilities of stakeholders involved. In this respect, we put forward a six-layered model for levels of autonomy for surgery robots as a (disregarded) model for modulating the human–robot interaction (HRI) between the surgeon (user) and the robot (manufacturer). We zoom in on the relationship between the social and legal implications for HRI in light of these autonomy levels. One of the implications we highlight is the increasing complexity in allocating responsibility in a highly complex context featuring humans and robots performing a joint task. Although there are independent regulations for surgical robots and the multiple stakeholders involved in the surgery robot ecosystem, there is a lack of framework(s) directed towards the robotic surgery ecosystem as a whole. Consequently, the stakeholders’ liability for the risks arising from the interplay between surgeons and robots (and robot developers) throughout the surgical process remains unclear. The progressive autonomy levels further confuse this panorama. This article aims to review these gaps and present specific recommendations that can be added as an additional layer to existing frameworks to simplify the complexities in the robotic surgery ecosystem, especially to robot developers (manufacturers) and robot users (surgeons and hospitals).
This article is structured as follows. Section 2 provides an overview of the current state of the art of robot surgeries' innovation and the surgical procedures for which they are deployed. We map the ecosystem where healthcare robots are inserted, including doctors, medical professionals, patients, caregivers (CGs), healthcare providers, and technology providers, to better understand their roles and responsibilities. Regarding the latter, we categorize robots based on their autonomy level, which is of fundamental importance because the more autonomous a system is, the less human control exists. Section 3 identifies some of the risks arising from the use of highly sophisticated robots in surgery. Section 4 discusses different challenges that increasingly blur the understanding of who is responsible for what in highly sophisticated robotic surgery ecosystems. This is because understanding the exact role of humans in highly autonomous robotic surgeries is essential to map liability and bring certainty concerning the ascription of responsibility. In this sense, amongst all identified stakeholders, hospital management will increasingly have to pay attention to healthcare automation, education, and training schemes for medical staff, and the terms that establish the division of responsibility between the different actors involved. In Sect. 5, we propose potential solutions to ascertain clarity in the role of stakeholders before, during, and after robot-enabled surgeries. In this sense, we introduce the need to develop a framework for robot operators' skill development and a compliance tool named Robot Impact Assessment (ROBIA) to assist developers in complying with that framework. To help ease the allocation of responsibility, we introduce the concept of Robo-Terms, inspired by the international trade system 'Incoterms,' which establishes the responsibility among complex interaction chains. To ease responsibility management, we also put forward the creation of a standardized adverse event reporting mechanism that could support evidence-based regulations. In Sect. 6, we conclude that the full benefits of robotic innovations and solutions to deliver surgeries cannot be realized until there is better clarity on the division of responsibilities channeling robot autonomy and human performance, support, and oversight; a transformation in the education and training of medical staff, and a clarification of the complex interplay between manufacturers, healthcare providers, and patients.
2 Surgical Robots and Stakeholder Ecosystem
The healthcare service ecosystem is the network of stakeholders, processes, and materials necessary for the treatment of an ailment by way of medical intervention on a patient (de Vries 2016). The European project 'RoboLaw' identified an extensive list of robotics stakeholders in general used in society (Palmerini et al. 2014). The list includes producers and employers of robots, insurance companies, trade-unions, user associations, professional users, and policymakers (PMs). To a certain extent, these stakeholders can also be identified in the field of healthcare robots. However, the field of healthcare calls for a more specific approach because of the many parties involved and the healthcare setting's particular nature.
The healthcare robot ecosystem includes doctors, medical professionals, patients, family members, CGs, healthcare providers, or even technology providers. All these stakeholders have similar goals, although they perceive healthcare from different viewpoints (Deijer 2004), namely: providing (medical) care and independence, preserving patients' dignity, and empowering those with special needs (Simshaw et al. 2016). Their relationship with robots is also different. A common and practical approach is to divide the stakeholders in healthcare robotics into primary, secondary, and tertiary stakeholders [75]. The primary stakeholders are those who will use healthcare robots on a regular or even daily basis, including direct robot users (DRUs), clinicians (CL), and CGs. The secondary stakeholders are involved in the use and development of healthcare robots, but will not directly use them themselves. This group includes robot makers (RMs), the environmental service workers (ESWs), and health administrators (HAs). The tertiary stakeholders are those who are interested in the use and deployment of healthcare robots in society, including PMs, insurance providers (ICs), and advocacy groups (AGs), that will unlikely use the robots directly in their capacity [75].
The European Foresight Monitoring Network, EFMN (2008) defined healthcare robots as ‘systems able to perform coordinated mechatronic actions (force or movement exertions) based on processing information acquired through sensor technology, to support the functioning of impaired individuals, medical interventions, care and rehabilitation of patients and also individuals in prevention programs.’ Over the years, the Policy Department for Economic, Scientific, and Quality of Life Policies of the European Parliament identified robotic surgery, care, and socially assistive robots, rehabilitation systems, and training for healthcare workers as ‘the most interesting applications of healthcare robots’ (Dolic et al. 2019). The robotic surgery ecosystem thus forms a smaller ecosystem within the complex healthcare robots’ ecosystem, and it comprises the surgeon, the nurses and other staff members that help the doctor during the surgical procedure, and the patient as the DRUs. The hospital administration also plays a role, as they are usually the ones looking for reliable measurements of processes cost, quality, and efficiency.
Although the central role that robots play within the surgery robot ecosystem affects and is influenced by the roles and responsibilities of all other involved stakeholders [95], robot autonomy should not necessarily exempt other stakeholders' responsibilities [90] or replace them completely [53]. Robots operate as a part of complex ecosystems comprising several components, including cloud services, that may entail multiple processes over which different persons, natural or legal, exercise control and might be held liable [37, 82]. Moreover, the surgeon and the support staff will still be integral to the surgical environments for many functions, such as selecting the process parameters or positioning the patient, stressing further the essential role humans still have in robot-mediated surgeries.
2.1 Surgical Robots’ State of the Art
Surgical robots are service robots supporting surgeons during surgical procedures, now called robot-assisted surgery (RAS). Robots used in surgery are not always surgical robots [12]. Medical devices within the definition of robots exist in current surgeries like robotic shape actuated operating tables or robotized microscopes. However, these two are usually not considered surgical robots. A robotic surgical instrument is an invasive device with an applied part, intended to be manipulated by robotically-assisted surgical equipment (RASE) to perform surgery tasks (IEC 80601-2-77:2019).
Generally, robotic surgical systems operate within three different function-areas of medical practice, namely: (1) acquisition and analysis of information, (2) division of surgical trajectories or plan of actions, and (3) execution of the surgery [64]. In this article, we focus on the performance of the surgery only. Furthermore, surgical robots are used in different medical areas, usually on a spectrum that ranges from surgical robots that are more generic in nature, such as the da Vinci System®, to highly specific surgical robots, such as the PRECEYES Surgical System (R2D2). Depending on their capability and level of autonomy, surgical robots may be used for surgical procedures, ranging from less complicated surgeries on rigid body parts to more complex surgeries on soft tissues [72]. Table 1 provides some examples of surgical procedures currently performed with the help of a robot [34].
The technology incorporated in the surgical robot and its embodiment plays an essential role in the performance of the surgical procedure. The main characteristics of surgical robots include robotic arm(s) used to mimic and extend human movement, cutting instruments, cameras, and X-ray systems. They usually also have surgeon consoles and probes, and mobile compartments and tools. In practice, robotic platforms for surgical procedures involve an interplay between the sophisticated automated platform on one side and the surgeon, along with his/her team on the other [1]. The outcome and implications of such shared task performance essentially depends on how they are attuned to one another. This will inevitably change as surgical procedures become increasingly automated and the robotics introduced into the surgical environment are characterised by increasing levels of autonomy [38].
2.2 The Influence of Automation on Stakeholder Roles
Current surgical robots used to assist a surgeon performing (specific functions of) surgical procedures have different degrees of autonomy that vary from no autonomy to full autonomy, passing by being under the control of or in cooperation with a trained practitioner. While the Society of Automotive Engineers (SAE) has established automation levels for automobiles by the standard SAE J3016,Footnote 1 there are no universal standards that define the levels of autonomy in surgical robots. Nevertheless, within this context, Yang et al. [95] have proposed a six-layered model for medical robotics autonomy levels. Although a step forward into the investigation of the different levels of automation outside the automotive industry, the model proposed by Yang et al. [95] requires more detailing on how it applies to specific types of medical robots, including surgery robots, rehabilitation robots, and socially assistive robots. Indeed, medical robots' embodiment and capabilities differ vastly across surgical, physically/socially assistive, or serviceable contexts, and the involved HRI is also distinctive [38]. Socially assistive robots, for instance, interact with users socially, performing a task for the user, but physical contact with the user is minimal. In contrast, physically assistive robots (e.g., lower-limb exoskeletons) work towards a seamless integration with the user's movement, and surgical robots are collaborative robots that extend the surgeon's abilities. Therefore, building upon the model presented by Yang et al. [95], in Fig. 1, we introduce a similar six-layered model tailored explicitly to robotic surgical devices and the different actors' roles throughout the surgical procedure [29, 76, 89, 95].
Autonomy levels and the role of humans in robot surgeries as per Fosch-Villaronga et al. [38]
Over the last two decades, healthcare industry trends have suggested a strong affinity towards increased autonomy levels among stakeholders, and currently, surgical robots with level 4 autonomy are already in the development stage. Although commercially available robots with level 5 autonomy seem distant, some researchers already conceptualize them. Only performed on pigs so far (Greenemeier 2020), however, current robot surgeons cannot yet perform an entire surgery completely independent from the beginning to the end on humans [80]. The efforts to transit from the presently available level 3 robots towards level 4 robots indeed suggests that, in principle, the deployment of surgical robots with fully autonomous capabilities equivalent to level 5 is the ulterior motive of researchers and engineers working in this field [96].
The term 'autonomy' refers to the quality or state of being self-governing (Merriam-Webster). As such, the term 'robot autonomy' refers to a robot's capability to execute specific tasks based on current state and sensing without human intervention (ISO 8373:2012). In practice, it relates to the robot's ability to perform particular functions of the surgical procedure independently, with humans still needed for other parts of the process, including supervision. As indicated in Fig. 1, for surgery robots this means that although there is a gradual decrease in the active role of the human surgeon in favor of increasingly autonomous robots [95], there is no complete elimination of humans from the surgical procedure. The role of the medical support staff, for instance, will remain integral and crucial to the surgical environments for a multitude of functions, such as selecting the process parameters or positioning the patient. At worst, and as illustrated in Fig. 1, the human surgeon's role changes from active performance to oversight. Likewise, the surgical robot's role varies from oversight to active performance as the surgical robot's autonomy increases. This nuance is essential to avoid ascribing or extending responsibility to the surgical robot, which the literature has repeatedly highlighted as a legit course of action in complex robotic ecosystems. Understanding the exact surgeon's role in RAS is thus essential to understand better who is responsible if something goes wrong.
Based on the robots' capability and the surgeon's role in performing the desired task, surgical robots can generally be classified into three categories [7]:
-
The shared-controlled approach, placed within the scope of levels 0 and 1, refers to a surgical environment wherein one or more robotic devices work in tandem with the surgeon [68]. Here, the surgeon primarily carries out the procedure using a robot that offers steady-hand manipulations of the instrument. Typically, the workspace splits into several segments allowing the system to behave differently based on different localization according to safe, close, boundary, or forbidden classifications (Boyarez et al. 2019). An example is when a surgeon moves a cutting tool in a tissue direction that should not be damaged. Following the shared-controlled approach, the robot will apply the haptic force feedback that will grow stronger as the cutting tool comes closer to the fragile tissue, signaling the surgeon extra caution ought to be taken. The Yomi dental robot by Neocis is an example of a shared-controlled surgical robot.
-
The tele-controlled approach, also called master–slave, remote-controlled or telesurgical, corresponds to autonomy level 2. A human surgeon operates the robot from a (close) distance with no pre-programmed or autonomous elements. The surgeon (master) tele-controls the robotic arms (slave element), to which the surgical instruments are attached using a console (master controller). Such robotic platforms are usually configured with an optical system and computer-aided motion stabilization with a plurality of sensors for providing haptic feedback to the surgeon. Typical examples of teleoperated robots are the da Vinci and the ZEUS platforms.
-
The supervisory-controlled approach is the most automated and corresponds to three and four autonomy levels. This approach entails robotic systems configured to perform certain functions of the surgical procedure autonomously, with the surgeon being in a supervisory role throughout these moments of the process. In other words, the surgeon remains indispensable in devising a surgical strategy and overseeing the execution of the robot [68]. These robotic platforms generally comprise multiple robotic arms equipped with different surgical tools and are often powered by AI [e.g., artificial neural networks (ANN)] and fuzzy logic [2]. Examples of supervisory-controlled surgical robots are the PROBOT17 and ROBODOC18 platforms.
The difference between these approaches primarily revolves around the robot's autonomy level, the degree of assistance provided by robotic systems during the execution of surgical procedures, and the human surgeon's control exercised [34]. Research has pointed out that while the majority of RAS deploys surgical robots that follow the teleoperated and shared-controlled approach, supervisory-controlled and fully autonomous surgical robotic devices have not yet found their way into RAS [34]. This may be because human surgeons have shown to be considerably better than robots at weighing their experience to understand a particular context and make complex surgical judgments [34]. In the end, surgery is not only about enhanced dexterity but also about context understanding. In this sense, like cruise control and park assistance have made their way into cars progressively before realizing fully autonomous driving, fully autonomous surgical devices will probably enter clinical practice (Svoboda 2019), but only in the distant future. Similar to the automation in the automotive industry, the insertion of increasingly autonomous surgical robots into the healthcare domain will inevitably call into existence far-reaching risks and implications in terms of responsibility allocation if something goes wrong.
3 Robot-Enabled Surgery Convergence Risks
In medical practice, there exist no standard parameters for determining the success of surgical procedures. Surgery is generally considered unsuccessful if there is a deviation in the actual outcome from the intended outcome (Postachini and Cinotti 1999). Traditionally, the surgery's success was primarily dependent on the experience and the technical skill of the surgeon and his or her team. However, with a rise in the use of automated biomedical devices in surgeries, the medical equipment's performance has also become a vital factor in the surgery's overall outcome. Some studies have concluded that nearly 24% of all surgical failures can be attributed to failures of the equipment or the technology used in the surgical procedure [91]. In the case of RAS, studies have revealed that of the total recorded malfunctions that caused injury to a patient, over 20.9% of the reported malfunctions were due to the robotic arm and instruments' mechanical failure [27, 28].
While RAS benefits abound, introducing a robot to the doctor-to-patient relationship changes how the surgery is performed. RAS extends the abilities of the doctor, but it also presents new challenges for team fluency, which may be measured by quantitative metrics such as task execution time and the amount of concurrent motion [59]. A revision of 14 years of data from the Food and Drug Administration (FDA) shows that surgical robots can cause injury or death if they spontaneously power down mid-operation due to system errors or imaging problems [1]. Broken or burnt robot pieces can fall into the patient, electric sparks may burn human tissue, and instruments may operate unintendedly, all of which may cause harm, including death [1]. Additionally, security vulnerabilities may allow unauthorized users to remotely access, control, and issue commands to robots, potentially harming patients (FDA 2019). Meta-analytic research has shown how a robot’s performance attributes are primary drivers and critical for trust calibration in human robot interaction [48]. To prevent or, at least, reduce such preventable incidents in the future, and thereby establish trust amongst robot users, advanced techniques in the design and operation of robotic surgical systems and enhanced mechanisms for adverse event reporting ought to be adopted [1].
The following sections identify the potential convergence risks arising from the use of robots in surgical procedures.Footnote 2 For clarity, the risks have been classified based on their source of origin, arising from the surgical robot malfunctions or the negligence or lack of skill of the healthcare provider (i.e., medical malpractices hereafter).
3.1 Robotic Malfunctions
A surgical robot, just like any other mechanical or electrical machine, is said to have undergone a malfunction if it fails to deliver the quality of performance or match the specific performance parameters claimed to be capable of achieving or intended to accomplish in the given operating conditions (FDA 2019). Alemzadeh et al. [1] report that, in injuries in robotic surgeries from the year 2000 to 2013, nearly 33.7% of the patient deaths were caused due to the inherent risks of the ailment and the nature of the surgery that is a high-risk surgery or a low-risk surgery [1]. The remaining percentage of patient injuries was attributed to malfunctions and the surgeon and his team's errors during the surgery. Further analysis of these statistics revealed that while 7.1% of the problems were due to 'operator errors' and 6.3% were due to 'improper positioning of the patient,' nearly 62% of the injuries involved device malfunctions. This study concluded that, despite the widespread adoption of surgical robots in the healthcare industry, technical breakdowns and robotic malfunctions continue to happen during surgical procedures and cause patient harm.
Based on the nature of the reported cases, the potential malfunctions in surgical robots primarily fall in one or more of the sub-categories listed in Table 2 [1, 27].
Although the incorporation of surgical robots in healthcare has enhanced the doctor's capabilities, it has also increased the risks of patients suffering harm due to a failure or breakdown of the robotic platform that was not possible with conventional medical instruments (FDA 2020b [86]. Accordingly, a RAS's success depends not only on the doctor's diligence and expertise and his or her team but also on the quality and performance of the surgical robot developed by the robot manufacturer [63]. Therefore, the role of the robot manufacturer is central to the robotic surgery ecosystem, meaning that when mechanical breakdown or malfunction harms patients, the robot manufacturer can be held liable under the products liability framework of the respective jurisdiction.
3.2 Medical Malpractices in Robotic Surgery
A medical procedure comprises multiple stages. It begins with the diagnosis of an ailment and ends with the discontinuation of the post-operative treatment. Patient harm caused by negligence can occur in any of these stages. In the surgical environment, the occurrence of a robot's breakdown usually adjudicates robot manufacturers' liability. In cases where the patient suffered an injury, and there was no reported breakdown of the robot, almost intrinsically, the onus of liability shifts to surgeons on the grounds of negligence. Surgical errors, which may vary from wrong steps taken by the surgeon or his team, or wrong dosage given to the patient, to surgical materials, referred to as retained surgical bodies, being left behind in the patient's body, can cause the most severe of harms to patients [97]. Understanding the different steps in a medical procedure is instrumental in mapping the risks arising from doctors' actions even when robots also played a role. Depending on the nature and the stage of occurrence, an event of medical malpractice can generally be identified in one of the categories listed in Table 3 [55].
RAS has substantially reduced the perioperative complications present in conventional surgeries. However, there has been an increase in the iatrogenic complications related to positioning, trocar placement, gas insufflation, and surgical techniques in RAS [87]. Besides, incorrect usage of surgical robot equipment is also a significant factor behind patient injuries.
Moreover, apart from using the robot correctly, healthcare providers thus have an additional duty of care towards the patient by informing him/her of the risks associated with the procedure and seeking consent. The informed consent doctrine mandates the disclosure of the risk information of the specific ailment to the patient by the surgeon and seeking consent before the surgical procedure takes place. However, the dynamics change substantially in the RAS ecosystem [60]. Specifically, practitioners may be held liable for negligence in obtaining informed consent if they fail to disclose information relating to the risks associated with using the robot or withholding information of their skill at operating the surgical robot [60], p. 513).
Within the RAS ecosystem, the products liability framework covers incidents such as robotic equipment pieces falling into the patient's body under product failure and holds the robot manufacturer liable. Surgical errors originating from the surgical robot's incorrect usage, especially in master–slave systems, can be categorized as medical malpractice. The surgeon or his/her team members can be held liable for harm originating from such surgical errors.
4 Robot-Enabled Surgery Convergence Liability Management Challenges
The complex surgery robotics ecosystem is characterised by the multiple players it covers, and chains of responsibility may be extensive, complex, and often opaque—even to the parties involved [84]. Robot providers may depend on other providers and sub-providers, as a result of which it may be challenging to understand where faults or errors originated, determine causality, and attribute responsibility to a party—something that very often ends in parties trying to shift the blame on one another [37, 66].
As Calo [10] explains, if the vacuum cleaner named ‘Roomba’ harms someone when vacuuming the floor, the manufacturer that produced it (iRobot in this example) will probably be held liable because they have built the hardware and wrote the software. If, however, a Roomba is modified or is used for other purposes, then iRobot might seek to avoid liability. The case of surgery robots is different and more complex. A fundamental reason for this complexity resides in the human–machine interplay in robotic surgeries, inasmuch as the robot does not operate fully autonomously but in interaction with other humans, involved at different levels in different moments of the surgical procedure. The following subsections identify some of the core issues that are the root cause of the dilemmas concerning the identification of the liable entity in a complicated task.
4.1 Increased Automation Continues to Blur the Role of Stakeholders in Robot-Enabled Surgeries
Surgical errors, which may vary from wrong steps taken by the surgeon or his team, or wrong dosage given to the patient to surgical materials or bodies left behind in the patient's body, can cause the most severe types of harm to patients (Zejnullahu 2017). From a legal standpoint, the determination of manufacturers’ and medical practitioners' liability is more straightforward if the risks can be categorized under device malfunctions or medical malpractices, as litigation in such cases primarily relies on a fault-based system.
However, robotic surgery's technically complex nature involves an interplay between the surgical robot and the medical practitioner, which often determines the surgery outcome. In this sense, while an action that caused patient harm may have been performed by the robot autonomously, the doctor may be responsible for selecting the task to be executed by the robot. In an alternate example, the harm may originate from the doctor's action, but the robot may have chosen the surgical trajectory for the doctor. When moving from surgeon-2-patient procedures to surgeon-2-robot-2-patient, determining whether there was medical negligence or a product malfunction becomes increasingly challenging [66].
In cases where an AI-driven robotic platform is employed to acquire and analyze the patient's information, a misdiagnosis may mean the wrongful interpretation of the doctor's diagnostic results. It can result in a wrong line of treatment for the patient, causing harm. Given the current robotic ecosystem, where the doctor is responsible for making decisions regarding the treatment line, the doctor is generally at fault in misdiagnosis cases. While medication errors are mostly attributed to healthcare professionals, they can also be caused if patients withhold vital information regarding their medical history. In highly automated environments, however, it could also be possible that a machine causes medication error.
In the case of surgical robots, the product liability framework covers incidents such as pieces of the robotic equipment falling into the patient's body under product failure and holds the robot manufacturer liable. However, surgical errors originating from the surgical robot's incorrect usage, especially in master–slave systems, can be categorized as medical malpractice. The surgeon or his or her team members can be held liable for harm originating from such surgical errors. In this sense, the progressive autonomous levels of surgical robots blur such lines even further, making it ever more complex to determine which stakeholder in the surgery robot ecosystem is responsible if harm occurs. Such autonomy levels will force policy and standard makers to have clear guidance for each of the levels 0 through 5, as each party may be interested in being the least responsible for the tasks others do.
Apart from providing treatment prudently, healthcare providers also have the duty of conducting a risk assessment associated with the ailment and the proposed treatment for the patient (see below). After informing the patient about the risks involved and before beginning the treatment, it is legally necessary for the healthcare provider to seek the patient's consent to proceed with the proposed treatment line. In cases where the treatment is provided omitting the critical step of informing about the risks and potential complications and failing to seek consent, doctors and hospitals can be liable for malpractice even if there were no adverse impacts on the patient.
4.2 Autonomous Machines Do Not Imply a Decrease in Surgical Procedures’ Human Oversight
In the context of surgery robots, Yang et al. [95] state the more autonomous medical robots are, the less is human oversight. However, the phrasing oversight may give the impression that RAS is gearing towards humanless surgeries, whereas this is not happening (see Fig. 1). If medical robots operate increasingly autonomously it does not mean that humans are entirely out of the loop in surgical procedures. Even with the most autonomous robots used today, surgeries still require humans for several actions during the operation. Some of the actions refer to (but are not limited to) providing patient pre-operative care and maintaining them in a stable condition before the surgery. Also, selecting the robot's surgical trajectory and task to execute, the right process parameters of the surgical procedure, and positioning the patient in the correct way relative to the robot:
-
Selection of a surgical strategy for the robot. Even the most sophisticated surgical robots employed in healthcare delivery today are level 3 stereotactic robots and require surgeon approval before acting autonomously. Based on dynamic situation analysis capabilities, stereotactic robots devise multiple strategies for the surgeon to choose. Once the surgeon selects a strategy, the robot executes it. Therefore, a significant factor in the robot's intervention is the strategy that the surgeon chose for the robot.
-
Ergonomic positioning of the patient. In every surgical procedure, the patient must be placed in a specific position on the operation table. The placement of the patient must be such that maximum exposure to surgical sight is possible. Since the robot cannot understand the context or see the patient during surgery, the patient's correct placement determines the robotic intervention's success, turning the surgeon and support members' role even more critical.
-
Configuration of the robot. Inputting the patient parameters correctly, uploading the required images of the surgical area, and configuring the robot for the patient's surgery is crucial in robot surgeries [18]. This may also include placing sensors, tags, and other equipment accurately on the patient's body as these determine the performance of the robot. Since the surgeon and his/her team configure these parameters, their actions can directly affect the surgery outcome.
No matter how efficient or safe a robot is, if humans do not perform these functions adequately, the robot's action can injure the patient [83]. Thus, humans play an essential role in determining the robot's course of operation. It is true, however, that an unintended harmful behavior may occur when, despite having a well-defined function, a robot behaves in a way that differs from the designer's intent [70]. Still, this does not necessarily mean that we have technology in place without human responsibility [9, 33, 53]. Indeed, some long term studies report that out of all equipment related failures reported in surgical procedures, nearly 43% can be attributed to incorrect configuration and settings [1]. Stressing this point may help dissipate some of the concerns raised in favor of the so-called responsibility gap. The responsibility gap refers to the idea that, if a robot learns as it operates, and the robot itself can, in the course of its operation, change the rules by which it acts, then there is no reason why humans should be held responsible for the autonomous behaviors of such a robot [45, 65].
In light of these factors, a major dilemma for the courts is to decide whether the injury suffered by the patient was due to an error by the robot for which the robot manufacturer should be liable, or the harm was an indirect consequence of an error by the humans involved in the procedure for which the surgeon and his/her team should be held liable. While humans maintain an essential role in determining the robot's course of operation, so too does the level of adequacy of training of the relevant humans to do so correctly and diligently form an important element in allowing the courts to decide the aforementioned dilemma.
4.3 The Inadequacy of Robot Operators’ Skill Development
The rate of technology innovation is not proportional to the rate of robot operators’ skill development. With a rise in autonomy and algorithmic dependency, manufacturers are incorporating fault tolerance principles into the design of robots to mitigate risks [17, 21]. However, the incorrect use of medical equipment continues to be a major reason for medical errors [15, 46, 92]. User errors occur mainly when the surgeon or a member of his/her team operates the surgical robot incorrectly. While sometimes, these errors may be a consequence of negligence, they can also be caused inadvertently due to either psychological factors such as stress, loss of focus, or training related factors such as insufficient or incorrect training.
The regulation of the safety standards for medical devices is an integral aspect of every country's healthcare system, with almost every country having a national legislative framework for medical devices. There is a risk-based classification system for medical devices and surgical robots in both the European and US systems and are presently regulated under the same. However, it is challenging to place surgical robots under the existing risk-based frameworks because of the way robotic technology has evolved over the years from being technically advanced medical equipment to sophisticated machines. As a result, they cannot be placed under the same frameworks that regulate conventional medical equipment.
In the EU, surgical robots are classified as “Class IIb medical devices” under the Medical Devices Directive (MDD) (Directive 93/42/EEC). At present, the surgeons and support members' training is a responsibility shared between the manufacturer of the robot and hospitals employing the surgical robots and is usually limited to teaching the doctors how to use a specific robotic platform that they will be using [41]. Although the existing MDD is set to be replaced by a new EU Medical Device Regulation (2017), which will come into effect in 2021, the new regulation does not classify surgical robots under a separate class of medical devices. In practice, this implies that the regulation of the state-of-the-art surgical robots is done in the same way as scalpels and screws (The Regulatory Review 2020). In addition, the EU does not recognize separate training standards or licenses on surgeons and robot operators for the use of surgical robots [61].
In this respect, while RAS is rapidly evolving, there is no agreement on a specific learning curve, leading to disparities in the training frameworks adopted by the stakeholders [27, 28]. Subsequently, it is uncertain what certification is deemed adequate for surgeons. In conventional education models, doctors and practitioners are exposed to using basic medical equipment in their study years, however, the surgical robots' concept is relatively recent. As a result, surgeons and practitioners must acquire the necessary skills to use surgical robots through external training. Basic training modules generally comprise patient side training, including correct patient positioning, port, sensor placement, robot docking, and console side training, including lab simulation and supervised operation control (Sridahr et al. 2017). Presently, there are no standardized training modules for the use of surgical robots [43]. It implies that practitioners' dexterity using surgical robots depends on the quality of the robot manufacturer's training and may vary from one region to another. From a legal standpoint, in the case of harm caused to a patient due to incorrect usage of the surgical robot or improper positioning of the patient, a major dilemma is “who is at fault?” Prima facie, while a claim may be brought up against the surgeon or the practitioner for incorrect usage of the surgical robot, a counterclaim resides in the insufficiency of training provided by the robot manufacturer. In the absence of a license/accreditation framework, it is challenging for courts to decipher what an optimum level of training is and whether the robot manufacturer was at fault for not providing a required training level to the practitioners or the surgeon.
Another major problem is that the external training programs currently available are limited to familiarizing the practitioner with the surgical robot's correct usage. In instances such as the robot's stalling, where no direct harm to the patient is caused, the medical practitioner must immediately take over the surgery and complete it. In such cases, a dilemma arises if injuries occurred to the patient when the surgeon took over the surgery to perform it manually. Although harm could be directly attributed to the robot, the surgeon's inexperience and the subsequent time lost in taking over the surgery could be the determining factors for the harm.
5 Potential Solutions to Establish Clarity in the Role of Stakeholders Before, During, and After Robot-Enabled Surgeries
Healthcare robots operate as a part of a complex system comprising several cyber-physical elements, involving multiple intricate processes and close interaction with humans. Different persons, natural or legal, exercise control over these processes at various levels and could be held responsible [84]. However, concluding who is liable for a particular incident is incredibly difficult for issues arising from complex ecosystems, including healthcare robotics [37]. Still, in light of patient harm or an emergency, it is essential to think about what needs to be done and who has to do it (Sanvik 2020). In this section, we introduce a risk-based instrument to anticipate risks related to robot technology that can inform innovation management and also a theoretical approach to identify what is the best course of action in case harm occurs.
5.1 Establishing a Framework for Robot Operators’ Skill Development
Not having a clear framework that establishes a common minimum baseline for robotic surgeons creates incongruity in procedural safety. Given that even in the most advanced surgical robots currently in use, surgeons and their teams still perform multiple functions, an optimal training framework on the use of surgery robots must be established. Such a training framework entails technical training, whereby trainee surgeons may be taught: in the case of master–slave systems, how to use the robot, and in the case of semi-autonomous robots, how to supervise the performance of the robot, like experienced surgeons would oversee the performance of their trainees. To strike a balance between the theoretical and practical aspects of robotic surgery, training programs must include:
-
Dry lab training on machines such as, but not limited to, virtual reality (VR) simulators that can simulate real-time situations and challenges and be used for practice; and
-
Wet lab training through real human/animal robotic surgeries teaching the “reaction of tissues” when the robotic instrument operates, such as dissection, excision, or suturing.
Correct positioning of the patient relative to the surgical robot is one of the critical factors determining robotic surgery's success. In this sense, there should be a mandatory standard module for surgeons that focuses on the non-technical yet crucial aspects of patient positioning, robot docking, and port placement. Adequately trained professionals will ensure patient safety and eliminate the liability dilemma originating from patient harm due to the robot's action. However, this may be indirectly linked to incorrect positioning or placement of ports by the other stakeholders, i.e., the surgeon and his/her team members involved in the procedure.
In this respect, a sound basis for such a framework demands a clear and elaborate overview of the most significant categories currently established within the field of surgical robots, their intended purpose, use, and main characteristics [34]. Establishing and regulating surgical robots' training curriculums (following the previous section's sub-classifications) will streamline professionals' knowledge development cycle. Such a framework will improve surgeons and their team members' proficiency levels and help regulators and patients differentiate the formally qualified doctors from others. In case of harm, it will be easier to hold doctors liable for performing surgery without the required qualification and the hospitals responsible for employing under-qualified doctors and support staff.
5.2 Robot Impact Assessment
Even with standard training modules in place, as proposed in the previous section, it is likely that surgeons will have inadequate technical knowledge to map surgical robots' technical risks fully. Therefore, a more standardized model to convey to patients the medical risks and the technical risks associated with robotic surgery is needed. A critical instrument to assess the challenges and impacts that certain technologies have are technology assessments such as the Algorithmic Impact Assessment, Robot Impact Assessment (ROBIA), or the Assessment for Trustworthy AI (Reisman et al. 2018; Fosch-Villaronga 2015; HLEG AI 2019). We do not consider the Health Technology Assessment (HTA) because HTA assesses the need to introduce a new device in the clinical practice at the national health service's expenses when surgery robots are already in use.
A standardized procedure such as the ROBIA could help roboticists identify, analyze, and mitigate the legal risks associated with robot technology [33]. Such a ROBIA should include at least the following steps:
A standardized procedure could be developed and governed by Standards Development Organizations (SDO) or independently by firms that have a first mover or dominant position in the market and that could change common practice over time [94]. Mapping the surgical robot’s risks based on key parameters would further qualify surgeons and hospitals as ‘learned intermediaries.’ This enables them to adequately inform the patients and create a correct perception before seeking informed consent [27, 28]. This will provide patients with the necessary knowledge to conduct an assessment based on the negative consequences of damage caused by a possible problem and provide informed consent. This will reduce the burden of liability on the healthcare provider and will also keep the robotic manufacturer in the picture.
5.3 Managing Liability: Establishing a Clear Transition of Responsibility (Robo-terms)
There is a need for a framework tailored to address the liability challenges and strike a balance between the various stakeholders' opposing interests. Healthcare is not the only field where autonomous robotic technology has found its application and regulatory approaches can be borrowed from other fields where robotic technology has successfully been implemented. For example, the automobile industry's regulatory model is based on established levels of autonomy of the vehicles and defines the driver's role when the vehicle is in self-driving mode (J3016B: Taxonomy of definitions, SAE). Another model is that of the aviation industry, which imposes compliance requirements and operation protocols on airline operators. Airline operators generate profit by providing the service to passengers through sophisticated aircraft purchased from aircraft manufacturers and pilots employed to operate these aircraft [67]. The latest developments are in unmanned autonomous aircraft [19]. As hospitals' vested interests, just like interests of airline operators in aviation, are an integral aspect of robotic surgeries, they are in a position of control over the processes and procedures followed in robotic surgery.
Accordingly, aligned with the presupposed duty of care expected by patients, hospitals' role in ensuring infrastructural and procedural compliance with standards, protocols, and the latest practices are pivotal. For example, the contribution of the robot manufacturer in ensuring safety may be limited to manufacturing a surgical robot per the ISO standards on the basic safety and essential performance of surgical robots (ISO IEC 80601-2-77:2019). However, robotic surgery safety also depends on factors like the patient's condition, the surgeon's knowledge and expertise in medicine, and his/her skill at operating the robot. Therefore, the support staff's training involved in the robotic surgery and the quality of risk assessment done before the surgery are equally important. A hospital can significantly contribute to robotic surgeries' safety by employing well-trained surgeons and support staff, and having standard procedures in place for risk assessment, pre-operative care, and informed consent.
A best practice model defined by the framework for International Commercial Terms (Incoterms®) for product sales defines the rules for international traders' responsibility and could have applicability in highly-automated sectors. We propose a similar idea called Robo-terms to simplify the allocation of responsibility in highly automated environments (Fosch-Villaronga 2015). This system could ease the understanding of who is responsible if something goes wrong in each of the different robot usage stages. This is similar to the robot's self-explicative system that Johnson [53] mentioned and is in line with the Medical Device Regulation Eudamed Information system, which clearly identifies the different legal obligations that various stakeholders involved in the manufacturing, sale, distribution, and use of medical devices are obliged to comply with depending on their role (European Commission 2019). The Robo-terms employ a more distributive or responsibility-based approach that has not been fully considered yet. Although clearer legislation on the responsibilities in case of failed or harmful robotic systems could help allocate responsibility, Robo-term could be regarded as a starting point in this respect.
In line with this consideration, and although we acknowledge that more work is needed in this area, we propose the following Roboterms that, subject to the stakeholders' acceptance, would determine their roles and responsibilities at the different stages in the robot surgery ecosystem (see Fig. 2). These considerations could be taken into account for the policy formulation process [44, 62].
5.4 Standardized Adverse Event Reporting Mechanism Could Ease Responsibility Management
Over the years, as the number of robotic surgeries has increased, robot failures and adverse events have also increased (Friedman 2013). In the case of damage resulting from robot malfunction, an aggrieved party may sue a robot provider for compensation for a failure to take reasonable steps to avoid foreseeable risks. To establish causation, a claimant may need access to information regarding the specific incident and probably more general information about how the robotics ecosystem works. Although the incident reporting mechanisms in other domains, such as aviation, have proven to be effective in analysing and investigating the adverse event, its mere translation into healthcare may be difficult as there might be an inherent hesitancy in healthcare providers when it comes to reporting adverse events, to avoid liability [6]. Additionally, surgeons' experience in handling intraoperative complications and instrument malfunctions has also substantially improved over the last few years. As a result, events involving instrument malfunction but not involving patient harm, including robot complications, have been underreported [4], Cooper 2015). Reporting is a cumbersome task and may make little sense if, in the end, the patient was not harmed. Nevertheless, underreporting cases prevents building knowledge on the wrongs and the faults that could be useful for allocating responsibility and differentiating device failure from medical malpractice. Accordingly, there is a need to establish a standardized adverse event reporting mechanism that captures not only adverse events but also “near misses” or incidents where patient harm could have occurred in order to supplement the limited data available [5]. Such a mechanism will ease responsibility management as well as expose areas of improvement in product development for manufacturers, skill development for hospital management, and healthcare policymaking for policy makers.
Reporting databases exist in the US and Europe, although the latter does not have a centralized reporting system. MAUDE is the American Manufacturer and User Facility Device Experience, a reporting adverse event system in RAS. MAUDE's main problem is that it does not specify what constitutes an adverse event. Furthermore, the reporting is voluntary. As a result, the existing knowledge of robot malfunctions available to the public may be a mere fraction of the total robotic malfunctions. In the absence of standard parameters to determine what qualifies as an adverse event, healthcare providers usually consider patient harm as one of the determining factors.
Reporting robotic malfunctions in RAS, irrespective of whether the patient suffered, could help identify the frequency of surgical robots' malfunctions and RAS' complications. Building knowledge from particular cases may be complicated initially, but could offer a useful picture of how to improve RAS procedures [33]. Altogether, this could help narrow down the grey areas in allocating responsibility and constitute a lessons-learned repository. Over time, and from the knowledge generated from this repository, a robot surgeon framework could emerge to ensure safer RAS procedures [35].
6 Conclusions
This article has explored the influence of automation on stakeholder responsibility in surgery innovation. Robotic surgeries involve a complex human–machine interplay, and it is often difficult to understand who is responsible, and for what, when something goes wrong. In some situations, the robot's actions may appear to have inflicted harm on a patient. However, the root cause could be the patient's inaccurate positioning with respect to the robot, for which the healthcare professionals involved in the surgery are responsible. In other situations, the harm may be caused by the doctor's action during the surgery, but the root cause could be a wrong surgical trajectory selected by the surgical robot. However, even with the most advanced surgical robots currently in use, surgeons and their teams still perform multiple functions.
Moving slightly away from discourses that support the idea that the more autonomous medical robots are, the less human oversight is [95], we developed an analysis of the different roles that various stakeholders play in highly automated surgeries. We argue that humans continue to participate actively in highly automated surgical procedures in performance, oversight, or support [38]. While innovation surgery promises unparalleled potential for healthcare providers and patients alike, we conclude that the full benefits that robotic innovations and solutions could bring to healthcare providers and receivers cannot be realized until there is more clarity on each stakeholder’s role and responsibility in the surgical ecosystem, starting from the development stage of the robot to its installation and use in real healthcare settings. Of course, all activities within an organization may involve risk [ISO 31000:2009(en Risk management—Principles and guidelines]. Nevertheless, by channeling robot autonomy and human performance, support, and oversight; a transformation of the education and training of medical staff and creating a repository of adverse incidents that will help improve future development and research, the complex interplay between manufacturers, healthcare providers, and patients can be simplified. Obviously, as surgery robots continue to develop and become more sophisticated, the surgery robot ecosystem continues to evolve, and a new equilibrium will have to be achieved.
Since humans play an essential role in surgery automation, establishing more clarity concerning the complexity surrounding the roles and relationships in RAS procedures is critical. To examine these relationships and improve clarity regarding the division of roles and risks, we brought forward two proposals for further discussion within the community: the ROBIA and the Robo-Terms. An important implication in understanding the exact role of humans in highly autonomous robotic surgeries is the ability to map liability and bring legal certainty concerning the ascription of responsibility in case something goes wrong. A ROBIA can help anticipate and mitigate the surgery robots' problems with a compulsory adverse event reporting mechanism that can constitute a lessons-learned repository. We also stress the importance of having clear guidelines on the transmission of responsibility and strengthening the ecosystem's training to avoid unforeseen scenarios and reduce patient harm. In addition, the Roboterms framework highlights a liability attribution framework based on standard yardsticks for identifying which stakeholder is liable for which aspects of the robotic surgery. Such a framework will nudge the stakeholders to comply with the specific standards and procedures for which they are responsible.
These factors that take elements from management, context, institutional approach, and legal issues underlie the need for a multi-stakeholder positive transformation in the healthcare ecosystem critical to the successful implementation of surgery innovation. In the long run, bringing more certainty regarding the role and responsibility of each stakeholder will prove valuable for making long-term asset-specific investments in healthcare innovation and, most importantly, ensure patient safety in an increasingly robotized healthcare.
Notes
See the taxonomy and definitions for terms related to on-road motor vehicle automated driving systems. SAE International, available at http://standards.sae.org/j3016_201609/.
According to the Oxford's Learner's Dictionary, convergence means (1) to move toward a place from different directions and meet, (2) to move toward each other and meet at a point, or (3) to become very similar or the same. Here, convergence risks thus should be understood as the (potential) risks associated with increased collaboration between surgical robots and the other stakeholders involved in the surgery robot ecosystem, in particular surgeons and support staff.
References
Alemzadeh H, Raman J, Leveson N, Kalbarczyk Z, Iyer RK (2016) Adverse events in robotic surgery: a retrospective study of 14 years of FDA data. PLoS ONE 11(4):e0151470. https://doi.org/10.1371/journal.pone.0151470
Amato F, López A, Peña-Méndez E, Vaňhara P, Hampl A, Havel J (2013) Artificial neural networks in medical diagnosis. J Appl Biomed 11(2):47–58
Amodei D, Olah C, Steinhardt J, Christiano P, Schulman J, Mané D (2016) Concrete problems in AI safety. arXiv preprint arXiv:1606.06565
Andonian S, Okeke Z, Okeke DA, Rastinehad A, Vanderbrink BA, Richstone L, Lee BR (2008) Device failures and patient injuries associated with robot-assisted laparoscopic surgeries: a review of the FDA database. J Urol 179(4S):344–344
Barach P, Small SD (2000) Reporting and preventing medical mishaps: lessons from non-medical near miss reporting systems. BMJ 320(7237):759–763
Billings CE (1998) Some hopes and concerns regarding medical event-reporting systems: lessons from the NASA Aviation Safety Reporting System. Arch Pathol Lab Med 122(3):214
Boyraz P, Dobrev I, Fischer G, Popovic MB (2019) Robotic surgery. In: Popovic MB (ed) Biomechatronics. Academic, London, pp 431–450
Broekens J, Heerink M, Rosendal H (2009) Assistive social robots in elderly care: a review. Gerontechnology 8(2):94–103
Bryson JJ, Diamantis ME, Grant TD (2017) Of, for, and by the people: the legal lacuna of synthetic persons. Artif Intell Law 25(3):273–291
Calo R (2014) The need to be open: U.S. laws are killing the future of robotics. Mashable. https://mashable.com/2014/01/01/us-law-robotics-future/?europe=true. Accessed 1 Jan 2014
Chang YC, Miles I, Hung SC (2014) Introduction to special issue: managing technology-service convergence in Service Economy 3.0. Technovation 34(9):499–504
Chinzei K (2019) Safety of surgical robots and IEC 80601-2-77: the first international standard for surgical robots. Acta Polytech Hung 16(8):171–184
CoE, Council Directive 93/42/EEC of 14 June 1993 concerning ... (n.d.). Rule 5-6, 9. https://eur-lex.europa.eu/LexUriServ/LexUriServ.do?uri=CONSLEG:1993L0042:20071011:en:PDF
Cooper MA, Ibrahim A, Lyu H, Makary MA (2015) Underreporting of robotic surgery complications. J Healthc Qual 37(2):133–138
Courdier S, Garbin O, Hummel M, Thoma V, Ball E, Favre R, Wattiez A (2009) Equipment failure: causes and consequences in endoscopic gynecologic surgery. J Minim Invasive Gynecol 16(1):28–33
Cresswell K, Cunningham-Burley S, Sheikh A (2018) Health care robotics: qualitative exploration of key challenges and future directions. J Med Internet Res 20(7):e10410
Crestani D, Godary-Dejean K, Lapierre L (2015) Enhancing fault tolerance of autonomous mobile robots. Robot Auton Syst 68:140–155
Crouch H (2020) Legal considerations when it comes to robotics in surgery. Digit Health. https://www.digitalhealth.net/2020/01/legal-considerations-robotics-surgery/. Accessed 26 Oct 2020
Custers BHM (2016) Flying to new destinations: the future of drones. In: Custers BHM (ed) The future of drone use. Springer, Heidelberg
De Vries CR, Rosenberg JS (2016) Global surgical ecosystems: a need for systems strengthening. Ann Glob Health 82(4):605–613
Doran M, Sterritt R, Wilkie G (2020) Autonomic architecture for fault handling in mobile robots. Innov Syst Softw Eng 16(3):263–288
Drejer I (2004) Service innovation. Organizational responses to technological opportunities and market imperatives; Joe Tidd and Frank M. Hull (Eds); Imperial College Press, London, September 2003; 437 pages, Book Review. Technovation 11(24):922–923
European Commission (2019) Draft Functional specifications for the European Database on Medical Devices (Eudamed)-First release (High(1)) to be audited. Directorate-General for Internal Market, Industry, Entrepreneurship and SMEs, Brussels. https://ec.europa.eu/health/md_eudamed/overview_en. Accessed 30 Nov 2020
European Parliament (2017) Resolution with Recommendations to the Commission on Civil Law Rules on Robotics (2015/2103(INL)). http://www.europarl.europa.eu/doceo/document/TA-8-2017-0051_EN.html. Accessed 07 Oct 2020
European Parliament (2019) Resolution on a comprehensive European industrial policy on artificial intelligence and robotics (2018/2088(INI)). https://www.europarl.europa.eu/doceo/document/TA-8-2019-0081_EN.html. Accessed 07 Oct 2020
Faust RA (2007) Robotics in surgery: history, current and future applications. Nova Publishers, New York
Ferrarese A, Pozzi G, Borghi F, Marano A, Delbon P, Amato B et al (2016) Malfunctions of robotic system in surgery: role and responsibility of surgeon in legal point of view. Open Med 11(1):286–291
Ferrarese A, Pozzi G, Borghi F, Pellegrino L, Di Lorenzo P, Amato B et al (2016) Informed consent in robotic surgery: quality of information and patient perception. Open Med 11(1):279–285
Ficuciello F, Tamburrini G, Arezzo A, Villani L, Siciliano B (2019) Autonomy in surgical robots and its meaningful human control, Paladyn. J Behav Robot 10(1):30–43. https://doi.org/10.1515/pjbr-2019-0002
Food and Drug Administration, FDA (2019) Code of Federal Regulations. Title 21. https://www.accessdata.fda.gov/scripts/cdrh/cfdocs/cfcfr/CFRSearch.cfm?CFRPart=803&showFR=1. Accessed 12 Oct 2020
Food and Drug Administration, FDA (2020a) Cybersecurity safety communications. https://www.fda.gov/medical-devices/digital-health/cybersecurity. Accessed 7 Oct 2020
Food and Drug Administration, FDA (2020b) Reports. https://www.accessdata.fda.gov/scripts/cdrh/cfdocs/cfmaude/search.cfm. Accessed 7 Oct 2020
Fosch-Villaronga E (2019) Responsibility, liability, and accountability. In: Fosch-Villaronga E (ed) Robots, healthcare, and the law: regulating automation in personal care. Routledge, London, pp 147–171
Fosch-Villaronga E, Drukarch H (2021) On healthcare robots. Concepts, definitions, and considerations for healthcare robot governance, pp 1–87. ArXiv pre-print, https://arxiv.org/abs/2106.03468
Fosch-Villaronga E, Golia A Jr (2019) Robots, standards and the law. Rivalries between private standards and public policymaking for robot governance. Comput Law Secur Rev 35(2):129–144
Fosch-Villaronga E, Mahler T (2021) Cybersecurity, safety and robots: strengthening the link between cybersecurity and safety in the context of care robots. Comput Law Secur Rev 41:105528
Fosch-Villaronga E, Millard C (2019) Cloud robotics law and regulation: challenges in the governance of complex and dynamic cyber–physical ecosystems. Robot Auton Syst 119:77–91
Fosch-Villaronga E, Khanna P, Drukarch H, Custers BH (2021) A human in the loop in surgery automation. Nat Mach Intell 3(5):368–369
Francis D, Bessant J (2005) Targeting innovation and implications for capability development. Technovation 25(3):171–183
Friedman DCW, Lendvay TS, Hannaford B (2013) Instrument failures for the da Vinci surgical system: a Food and Drug Administration MAUDE database study. Surg Endosc 27:1503–1508
Grillone G, Jalisi S (2014) Robotic surgery of the head and neck. Springer, Berlin, pp 30–31
Greenmeier L (2020) Robot surgeon successfully sews pig intestine. Sci Am. https://www.scientificamerican.com/article/robot-surgeon-successfully-sews-pig-intestine/
Guzzo TJ, Gonzalgo ML (2009) Robotic surgical training of the urologic oncologist. Urol Oncol Semin Orig Investig 27(2):214–217. https://doi.org/10.1016/j.urolonc.2008.09.019
Hailey D, McDonald I (1996) The assessment of diagnostic imaging technologies: a policy perspective. Health Policy 36(2):185–197
Hellström T (2013) On the moral responsibility of military robots. Ethics Inf Technol 15(2):99–107
Hempel S, Maggard-Gibbons M, Nguyen DK, Dawes AJ, Miake-Lye I, Beroes JM et al (2015) Wrong-site surgery, retained surgical items, and surgical fires: a systematic review of surgical never events. JAMA Surg 150(8):796–805
High Level Expert Group on AI, HLEG AI (2019) Ethics guidelines for trustworthy AI. https://ec.europa.eu/digital-single-market/en/news/ethics-guidelines-trustworthy-ai. Accessed 7 Oct 2020
Hancock PA, Billings DR, Schaefer KE, Chen JY, De Visser EJ, Parasuraman R (2011) A meta-analysis of factors affecting trust in human–robot interaction. Hum Factors 53(5):517–527
ICC-International Chamber of Commerce (2020) Incoterms® 2020. ICC-International Chamber of Commerce. https://iccwbo.org/resources-for-business/incoterms-rules/incoterms-2020/. Accessed 6 July 2020
ISO 31000:2009(en) Risk management—principles and guidelines. https://www.iso.org/obp/ui/es/#iso:std:iso:31000:ed-1:v1:en
ISO (2019) IEC 80601-2-77:2019; medical electrical equipment—Part 2-77: particular requirements for the basic safety and essential performance of robotically assisted surgical equipment. https://www.iso.org/standard/68473.html
Jaffray B (2005) Minimally invasive surgery. Arch Dis Child 90(5):537–542
Johnson DG (2015) Technology with no human responsibility? J Bus Ethics 127(4):707–715
Khodabandehloo K (1996) Analyses of robot systems using fault and event trees: case studies. Reliab Eng Syst Saf 53(3):247–264
Justia (2019) Common types of medical malpractice. https://www.justia.com/injury/medical-malpractice/common-types-of-medical-malpractice/. Accessed 12 Oct 2020
Korb W, Kornfeld M, Birkfellner W, Boesecke R, Figl M, Fuerst M et al (2005) Risk analysis and safety assessment in surgical robotics: a case study on a biopsy robot. Minim Invasive Ther Allied Technol 14(1):23–31
Lane T (2018) A short history of robotic surgery. Ann R Coll Surg Engl 100(6 Suppl):5–7
Lanfranco AR, Castellanos AE, Desai JP, Meyers WC (2004) Robotic surgery: a current perspective. Ann Surg 239(1):14–21. https://doi.org/10.1097/01.sla.0000103020.19595.7d
Lasota PA, Fong T, Shah JA (2017) A survey of methods for safe human–robot interaction. Now Publishers, Norwell, p 285
Lee YL, Kilic GS, Phelps JY (2011) Medicolegal review of liability risks for gynecologists stemming from lack of training in robot-assisted surgery. J Minim Invasive Gynecol 18(4):512–515
Leenes R, Palmerini E, Koops B-J, Bertolini A, Salvini P, Lucivero F (2017) Regulatory challenges of robotics: some guidelines for addressing legal and ethical issues. Law Innov Technol 9(1):1–9. https://doi.org/10.1080/17579961.2017.1304921
Liao SH (2005) Technology management methodologies and applications: a literature review from 1995 to 2003. Technovation 25(4):381–393
Lucas SM, Pattison EA, Sundaram CP (2011) Global robotic experience and the type of surgical system impact the types of robotic malfunctions and their clinical consequences: an FDA MAUDE review. BJU Int 109(8):1222–1227
Manzey D, Strauss G, Trantakis C, Lueth T, Roettger S, Bahner-Heyne JE et al (2009) Automation in surgery: a systematic approach. Surg Technol Int 18:37–45
Matthias A (2004) The responsibility gap: ascribing responsibility for the actions of learning automata. Ethics Inf Technol 6(3):175–183
McLean T (2007) The complexity of litigation associated with robotic surgery and cybersurgery. Int J Med Robot Comput Assist Surg 3(1):23–29
Mauritz A (2003) Chapters 2–5. In: Liability of the operators and owners of aircraft for damage inflicted to persons and property on the surface. Shaker Publishers, Maastricht
Mohammad S (2013) Robotic surgery. J Oral Biol Craniofac Res 3(1):2
Nouaille L, Laribi MA, Nelson CA, Zeghloul S, Poisson G (2017) Review of kinematics for minimally invasive surgery and tele-echography robots. J Med Devices 11(4):040802
Pistono F, Yampolskiy RV (2016) Unethical research: how to create a malevolent artificial intelligence. arXiv preprint arXiv:1605.02817.
Postacchini F, Cinotti G (1999) Surgical failures. In: Postacchini F (ed) Lumbar disc herniation. Springer, Vienna, pp 557–574
Prabu AJ, Narmadha J, Jeyaprakash K (2014) Artificial intelligence robotically assisted brain surgery. Artif Intell 4(05)
Reddy CL, Mitra S, Meara JG, Atun R, Afshar S (2019) Artificial Intelligence and its role in surgical care in low-income and middle-income countries. Lancet Digit Health 1(8):e384–e438
Regulation (EU) 2017/745 of the European Parliament and of the Council of 5 April 2017 on Medical Devices, amending Directive 2001/83/EC, Regulation (EC) No 178/2002 and Regulation (EC) No 1223/2009 and Repealing Council Directives 90/385/EEC and 93/42/EEC (text with EEA relevance)
Riek LD (2017) Healthcare robotics. Commun ACM 60(11):68–78
Rosenberg LB (1993), Virtual fixtures: perceptual tools for telerobotic manipulation. In: Proceedings of IEEE virtual reality annual international symposium, Seattle, WA, USA, pp 76–82
SAE (2020) J3016B: taxonomy and definitions for terms related to driving automation systems for on-road motor vehicles. SAE International. https://www.sae.org/standards/content/j3016_201806/. Accessed 12 Oct 2020
Sandvik KB (2020) “Smittestopp”: if you want your freedom back, download now. Big Data Soc 7(2):2053951720939985
Scassellati B, Admoni H, Matarić M (2012) Robots for use in autism research. Annu Rev Biomed Eng 14:275–294
Shademan A, Decker RS, Opfermann JD, Leonard S, Krieger A, Kim PC (2016) Supervised autonomous robotic soft tissue surgery. Sci Transl Med 8(337):337ra64. https://doi.org/10.1126/scitranslmed.aad9398
Simshaw D, Terry N, Hauser K, Cummings ML (2015) Regulating healthcare robots: Maximizing opportunities while minimizing risks. Richmond J Law Technol 22:1
Singh J, Walden I, Crowcroft J, Bacon J (2016) Responsibility and machine learning: part of a process. https://papers.ssrn.com/sol3/papers.cfm?abstract_id=2860048. Accessed 6 Nov 2020
Shah SB, Hariharan U, Bhargava AK, Rawal SK, Chawdhary AA (2017) Robotic surgery and patient positioning: ergonomics, clinical pearls and review of literature. Trends Anaesth Crit Care 14:21–29
Singh J, Millard C, Reed C, Cobbe J, Crowcroft J (2018) Accountability in the IoT: systems, law, and ways forward. Computer 51(7):54–65
Sridhar AN, Briggs TP, Kelly JD, Nathan S (2017) Training in robotic surgery—an overview. Curr Urol Rep 18(8):58
Tapper A, Leale D, Megahan G, Nacker K, Killinger K, Hafron J (2019) Robotic instrument failure—a critical analysis of cause and quality improvement strategies. Urology 2019(131):125–129. https://doi.org/10.1016/j.urology.2019.02.052(Epub31May
Tourinho-Barbosa RR, Tobias-Machado M, Castro-Alfaro A, Ogaya-Pinies G, Cathelineau X, Sanchez-Salas R (2018) Complications in robotic urological surgeries and how to avoid them: a systematic review. Arab J Urol 16(3):285–292
Tucker MR, Olivier J, Pagel A, Bleuler H, Bouri M, Lambercy O et al (2015) Control strategies for active lower extremity prosthetics and orthotics: a review. J Neuroeng Rehabil 12(1):1–30
Varma TRK, Eldridge P (2006) Use of the NeuroMate stereotactic robot in a frameless mode for functional neurosurgery. Int J Med Robot Comput Assist Surg 2(2):107–113
Vedder AH, Custers BHM (2009) Whose responsibility is it anyway? Dealing with the consequences of new technologies. In: Sollie P, Düwell M (eds) Evaluating new technologies: methodological problems for the ethical assessment of technology developments. Springer, New York, pp 21–34
Weerakkody RA, Cheshire NJ, Riga C, Lear R, Hamady MS, Moorthy K et al (2013) Surgical technology and operating-room safety failures: a systematic review of quantitative studies. BMJ Qual Saf 22(9):710–718
Ward JR, Clarkson PJ (2004) An analysis of medical device-related errors: prevalence and possible solutions. J Med Eng Technol 28(1):2–6
World Commission on the Ethics of Scientific Knowledge and Technology (COMEST) (2017) Report of COMEST on Robotics Ethics. SHS/YES/COMEST-10/17/2 REV
Xie Z, Hall J, McCarthy IP, Skitmore M, Shen L (2016) Standardization efforts: the relationship between knowledge dimensions, search processes and innovation outcomes. Technovation 48:69–78
Yang GZ, Cambias J, Cleary K, Daimler E, Drake J, Dupont PE, Hata N, Kazanzides P, Martel S, Patel RV, Santos VJ, Taylor RH (2017) Medical robotics—regulatory, ethical, and legal considerations for increasing levels of autonomy. Sci Robot 2(4):eaam8638
Yip M, Das N (2017) Robot autonomy for surgery. http://arxiv.org/abs/1707.03080. Accessed 12 Oct 2020
Zejnullahu VA, Bicaj BX, Zejnullahu VA, Hamza AR (2017) Retained surgical foreign bodies after surgery. Open Access Maced J Med Sci 5(1):97
Acknowledgements
Part of this project was funded by the LEaDing Fellows Marie Curie COFUND Fellowship, a project that has received funding from the European Union’s Horizon 2020 Research and Innovation Programme under the Marie Skłodowska-Curie Grant Agreement No. 707404.
Author information
Authors and Affiliations
Corresponding author
Additional information
Publisher's Note
Springer Nature remains neutral with regard to jurisdictional claims in published maps and institutional affiliations.
Rights and permissions
Open Access This article is licensed under a Creative Commons Attribution 4.0 International License, which permits use, sharing, adaptation, distribution and reproduction in any medium or format, as long as you give appropriate credit to the original author(s) and the source, provide a link to the Creative Commons licence, and indicate if changes were made. The images or other third party material in this article are included in the article's Creative Commons licence, unless indicated otherwise in a credit line to the material. If material is not included in the article's Creative Commons licence and your intended use is not permitted by statutory regulation or exceeds the permitted use, you will need to obtain permission directly from the copyright holder. To view a copy of this licence, visit http://creativecommons.org/licenses/by/4.0/.
About this article
Cite this article
Fosch-Villaronga, E., Khanna, P., Drukarch, H. et al. The Role of Humans in Surgery Automation. Int J of Soc Robotics 15, 563–580 (2023). https://doi.org/10.1007/s12369-022-00875-0
Accepted:
Published:
Issue Date:
DOI: https://doi.org/10.1007/s12369-022-00875-0