Abstract
In this paper, the vibration and buckling behavior of a functionally graded piezoelectric porous cylindrical microshell under thermo-electro-mechanical loads are explored on the basis of modified couple stress theory and higher-order shear deformation theory. First, the model of a functionally graded piezoelectric porous cylindrical microshell composed of piezoelectric materials with gradient change in the thickness direction was described. Second, the governing equations of the microshell were derived by Hamilton’s principle and Maxwell equation. Third, the modal frequency and buckling equations of the microshell with simply supported ends were obtained on the basis of harmonic trigonometric functions. Finally, the effects of parameters on the modal frequency and buckling behavior were carried out by case studies. The results show that the modal frequency of the microshell can be adjusted by changing the porosity volume fraction, power index, applied voltage, axial load and geometric dimensions. It is also found that the vibration of the microshell is suppressed by positive voltage and axial compression but is strengthened by negative voltage and axial tension, and the material length scale parameter increases stiffness. In addition, the effects of applied voltage and axial load on the buckling behavior are larger than those of temperature. Results can be used to guide the design and application of piezoelectric devices.
Similar content being viewed by others
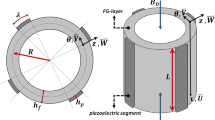
Abbreviations
- L :
-
Length of microshell
- R :
-
Mid-surface radius of microshell
- h :
-
Thickness of microshell
- x :
-
Axial coordinate
- θ :
-
Circumferential coordinate
- y :
-
Tangential coordinate
- z :
-
Radial coordinate
- T i, T o :
-
Temperatures of inner and outer surfaces
- T io :
-
Temperature difference
- P(z):
-
Material properties of microshell
- P i, P o :
-
Material properties of inner and outer surfaces
- N :
-
Power index
- α p :
-
Porosity volume fraction
- u x :
-
Axial displacement component
- u y :
-
Tangential displacement component
- u z :
-
Radial displacement component
- u :
-
Axial displacement component of any point on the mid-surface
- v :
-
Tangential displacement component of any point on mid-surface
- w :
-
Radial displacement component of any point on mid-surface
- φ x, φ y :
-
Rotation angles of mid-surface normal around x and y axes
- [C]:
-
Elastic moduli matrix
- [β]:
-
Thermal moduli matrix
- [d]:
-
Piezoelectric moduli matrix
- [ξ]:
-
Dielectric moduli matrix
- {σ}:
-
Stress vector
- {ε}:
-
Strain vector
- {E}:
-
Electric field vector
- {D}:
-
Electric displacement vector
- {p}:
-
Pyroelectric constants vector
- σ xx, σ yy :
-
Normal stresses
- T xy :
-
In-plane shear stress
- T xz, T yz :
-
Shear stresses along thickness direction
- ε xx, ε yy :
-
Normal strains
- γ xy :
-
In-plane shear strain
- γ xz, γ yz :
-
Shear strains along thickness direction
- Dx, Dy, Dz :
-
Electric displacement components
- E x, E y, E z :
-
Electric field components
- c ije :
-
Equivalent elastic moduli components
- ξ iie :
-
Equivalent dielectric moduli components
- d ije :
-
Equivalent piezoelectric moduli components
- β iie :
-
Equivalent thermal moduli components
- p ie :
-
Equivalent pyroelectric constants components
- Φ:
-
Electric potential inside the microshell
- φ :
-
Electric potential variation
- V :
-
Applied voltage
- {m}:
-
Vector of higher-order stress tensor
- {X}:
-
Vector of symmetric rotation gradient tensor
- l :
-
Material length scale parameter
- m ij :
-
Components of higher-order stress tensor
- X ij :
-
Components of symmetric rotation gradient tensor
- U :
-
Total strain energy
- U c :
-
Strain energy based on CCT
- U mc :
-
Strain energy based on MCST
- K :
-
Kinetic energy
- W :
-
Work done by external loads
- \(N_{XX}^{T}\) :
-
Thermal load
- N a :
-
Static axial load
- \(N_{XX}^{p}\) :
-
Electric load
- t :
-
Time variable
- l 0, l 1, l 2, l 3, l 4, l 6 :
-
Generalized inertia constants
- N ij, Q i :
-
Classical forces
- M ij, S ij, J i :
-
Classical moments
- Y ij :
-
Nonclassical forces
- Γ ij, P ij, T ij :
-
Nonclassical moments
- m :
-
Axial wavenumber
- n :
-
Circumferential wavenumber
- ω :
-
Modal frequency of microshell
- [G]:
-
Mass matrix
- {Δ}:
-
Displacement amplitude vector
- {X}:
-
External load vector
- [Z]:
-
Total stiffness matrix
- [Z 0]:
-
Stiffness matrix
- [Z N]:
-
Coefficient matrix of axial load
- [Z T]:
-
Coefficient matrix of temperature difference
- [Z V]:
-
Coefficient matrix of applied voltage
- N cr :
-
Static buckling load
- V cr :
-
Buckling voltage
- Ω:
-
Dimensionless frequency
- N d :
-
Dimensionless axial load
- N acr :
-
Defined buckling load
- N dcr :
-
Dimensionless buckling load
- h/R :
-
Dimensionless thickness
- L/R :
-
Dimensionless length
References
Q. L. Zhao, S. Q. Liu, J. H. Chen, G. P. He, J. J. Di, L. Zhao, T. T. Su, M. Y. Zhang and Z. L. Hou, Fast-moving piezoelectric micro-robotic fish with double caudal fins, Robotics and Autonomous Systems, 140 (2021) 103733.
J. T. Zhang, D. Qu, Z. Fang and C. Shu, Optimization of a piezoelectric wind energy harvester with a stepped beam, Journal of Mechanical Science and Technology, 34(11) (2020) 4357–4366.
F. S. Bai, L. Wang, K. D. Yang, Z. Y. He, G. Qi and J. Twiefel, Theoretical modeling and experimental investigation of a V-shaped traveling wave piezoelectric transducer for ultrasonic cavitation peening: part B, Applied Acoustics, 178 (2021) 107972.
P. P. Shi, J. Xie and S. Hao, Static response of functionally graded piezoelectric-piezomagnetic hollow cylinder/spherical shells with axial/spherical symmetry, Journal of Mechanical Science and Technology, 35(4) (2021) 1583–1596.
A. A. Jandaghian and O. Rahmani, Size-dependent free vibration analysis of functionally graded piezoelectric plate subjected to thermo-electro-mechanical loading, Journal of Intelligent Material Systems and Structures, 28(20) (2017) 3039–3053.
J. P. Su, Y. G. Qu, K. Zhang, Q. Zhang and Y. Tian, Vibration analysis of functionally graded porous piezoelectric deep curved beams resting on discrete elastic supports, Thin-Walled Structures, 164 (2021) 107838.
M. Askari, E. Brusa and C. Delprete, On the vibration analysis of coupled transverse and shear piezoelectric functionally graded porous beams with higher-order theories, The Journal of Strain Analysis for Engineering Design, 56(1) (2021) 29–49.
A. Lal, N. L. Shegokar and B. N. Singh, Finite element based nonlinear dynamic response of elastically supported piezoelectric functionally graded beam subjected to moving load in thermal environment with random system properties, Applied Mathematical Modelling, 44 (2017) 274–295.
M. F. Chen, H. L. Chen, X. L. Ma, G. Y. Jin, T. G. Ye, Y. T. Zhang and Z. G. Liu, The isogeometric free vibration and transient response of functionally graded piezoelectric curved beam with elastic restraints, Results in Physics, 11 (2018) 712–725.
F. N. Dehnavi and A. Parvizi, Electrothermomechanical behaviors of spherical vessels with different configurations of functionally graded piezoelectric coating, Journal of Intelligent Material Systems and Structures, 29(8) (2018) 1697–1710.
M. R. Barati, H. Shahverdi and A. M. Zenkour, Electromechanical vibration of smart piezoelectric FG plates with porosities according to a refined four-variable theory, Mechanics of Advanced Materials and Structures, 24(12) (2017) 987–998.
M. Komijani, Y. Kiani, S. E. Esfahani and M. R. Eslami, Vibration of thermo-electrically post-buckled rectangular functionally graded piezoelectric beams, Composite Structures, 98 (2013) 143–152.
A. A. Atai and D. Lak, Analytic investigation of effect of electric field on elasto-plastic response of a functionally graded piezoelectric hollow sphere, Journal of Mechanical Science and Technology, 30(1) (2016) 113–119.
X. T. He, Y. Z. Wang, S. J. Shi and J. Y. Sun, An electroelastic solution for functionally graded piezoelectric material beams with different moduli in tension and compression, Journal of Intelligent Material Systems and Structures, 29(8) (2018) 1649–1669.
W. Tian, T. Zhao and Z. C. Yang, Nonlinear electro-thermomechanical dynamic behaviors of a supersonic functionally graded piezoelectric plate with general boundary conditions, Composite Structures, 261 (2021) 113326.
A. M. Zenkour and Z. S. Hafed, Bending analysis of functionally graded piezoelectric plates via quasi-3D trigonometric theory, Mechanics of Advanced Materials and Structures, 27(18) (2020) 1551–1562.
C. Othmani, F. Takali, A. Njeh and M. H. Ben Ghozlen, Numerical simulation of Lamb waves propagation in a functionally graded piezoelectric plate composed of GaAs-AlAs materials using Legendre polynomial approach, Optik-International Journal for Light and Electron Optics, 142 (2017) 401–411.
J. Q. Li, Y. Xue, F. M. Li and Y. Narita, Active vibration control of functionally graded piezoelectric material plate, Composite Structures, 207 (2019) 509–518.
G. G. Sheng and X. Wang, Thermoelastic vibration and buckling analysis of functionally graded piezoelectric cylindrical shells, Applied Mathematical Modelling, 34(9) (2010) 2630–2643.
A. M. Zenkour and M. H. Aljadani, Buckling analysis of actuated functionally graded piezoelectric plates via a quasi-3D refined theory, Mechanics of Materials, 151 (2020) 103632.
A. M. Zenkour and M. H. Aljadani, Thermo-electrical buckling response of actuated functionally graded piezoelectric nano-scale plates, Results in Physics, 13 (2019) 102192.
M. Mohammad-Abadi and A. R. Daneshmehr, Size dependent buckling analysis of microbeams based on modified couple stress theory with high order theories and general boundary conditions, International Journal of Engineering Science, 74 (2014) 1–14.
M. Chen and S. J. Zheng, Size-dependent models of 0–1/0–3 polarized PLZT unimorphs and bimorphs based on a modified couple stress theory, Mechanics Research Communications, 98 (2019) 42–49.
A. Karamanli and T. P. Vo, Size-dependent behaviour of functionally graded sandwich microbeams based on the modified strain gradient theory, Composite Structures, 246 (2020) 112401.
M. E. Gurtin and A. I. Murdoch, A continuum theory of elastic material surfaces, Archive for Rational Mechanics and Analysis, 57(4) (1975) 291–323.
D. C. C. Lam, F. Yang, A. C. M. Chong, J. Wang and P. Tong, Experiments and theory in strain gradient elasticity, Journal of the Mechanics and Physics of Solids, 51(8) (2003) 1477–1508.
C. W. Lim, G. Zhang and J. N. Reddy, A higher-order nonlocal elasticity and strain gradient theory and its applications in wave propagation, Journal of the Mechanics and Physics of Solids, 78 (2015) 298–313.
A. C. Eringen, Nonlocal polar elastic continua, International Journal of Engineering Science, 10(1) (1972) 1–16.
A. C. Eringen, On differential equations of nonlocal elasticity and solutions of screw dislocation and surface waves, Journal of Applied Physics, 54(9) (1983) 4703–4710.
F. Yang, A. C. M. Chong, D. C. C. Lam and P. Tong, Couple stress based strain gradient theory for elasticity, International Journal of Solids and Structures, 39(10) (2002) 2731–2743.
Y. T. Beni, F. Mehralian and H. Razavi, Free vibration analysis of size-dependent shear deformable functionally graded cylindrical shell on the basis of modified couple stress theory, Composite Structures, 120 (2015) 65–78.
R. Ansari, M. F. Shojaei, V. Mohammadi, R. Gholami and M. A. Darabi, Size-dependent vibrations of post-buckled functionally graded Mindlin rectangular microplates, Latin American Journal of Solids and Structures, 11(13) (2014) 2351–2378.
S. Sahmani, R. Ansari, R. Gholami and A. Darvizeh, Dynamic stability analysis of functionally graded higher-order shear deformable microshells based on the modified couple stress elasticity theory, Composites Part B, 51 (2013) 44–53.
M. L. Dehsaraji, M. Arefi and A. Loghman, Size dependent free vibration analysis of functionally graded piezoelectric micro/nano shell based on modified couple stress theory with considering thickness stretching effect, Defence Technology, 17(1) (2021) 119–134.
S. Zeng, B. L. Wang and K. F. Wang, Nonlinear vibration of piezoelectric sandwich nanoplate with a functionally graded porous core with consideration of flexoelectric effect, Composite Structures, 207 (2019) 340–351.
M. Askari, A. R. Saidi and A. S. Rezaei, On natural frequencies of Levy-type thick porous-cellular plates surrounded by piezoelectric layers, Composite Structures, 179 (2017) 340–354.
M. Askari, E. Brusa and C. Delprete, Electromechanical vibration characteristics of porous bimorph and unimorph doubly curved panels, Actuators, 9(1) (2020) 7.
M. Askari, A. R. Saidi and A. S. Rezaei, An investigation over the effect of piezoelectricity and porosity distribution on natural frequencies of porous smart plates, Journal of Sandwich Structures and Materials, 22(7) (2020) 2091–2124.
M. R. Barati and A. M. Zenkour, Electro-thermoelastic vibration of plates made of porous functionally graded piezoelectric materials under various boundary conditions, Journal of Vibration and Control, 24(10) (2018) 1910–1926.
Y. Q. Wang, Electro-mechanical vibration analysis of functionally graded piezoelectric porous plates in the translation state, Acta Astronautica, 143 (2018) 263–271.
L. B. Nguyen, C. H. Thai, A. M. Zenkour and H. Nguyen-Xuan, An isogeometric Bézier finite element method for vibration analysis of functionally graded piezoelectric material porous plates, International Journal of Mechanical Sciences, 157–158 (2019) 165–183.
A. M. Zenkour and M. H. Aljadani, Porosity effect on thermal buckling behavior of actuated functionally graded piezoelectric nanoplates, European Journal of Mechanics/A Solids, 78 (2019) 103835.
E. Sadeghi Rad, A. R. Saidi, A. S. Rezaei and M. Askari, Shear deformation theories for elastic buckling of fluid-infiltrated porous plates: an analytical approach, Composite Structures, 254 (2020) 112829.
Y. F. Liu and Y. Q. Wang, Thermo-electro-mechanical vibrations of porous functionally graded piezoelectric nanoshells, Nanomaterials, 9(2) (2019) 301.
M. Ghadiri and H. SafarPour, Free vibration analysis of size-dependent functionally graded porous cylindrical microshells in thermal environment, Journal of Thermal Stresses, 40(1) (2017) 55–71.
N. Zhang, X. Zhao, S. J. Zheng and D. J. Chen, Size-dependent static bending and free vibration analysis of porous functionally graded piezoelectric nanobeams, Smart Materials and Structures, 29(4) (2020) 045025.
Y. Q. Wang, C. Ye and J. W. Zu, Identifying the temperature effect on the vibrations of functionally graded cylindrical shells with porosities, Applied Mathematics and Mechanics, 39(11) (2018) 1587–1604.
J. N. Reddy, A simple higher-order theory for lamnated composite plates, Journal of Applied Mechanics, 51(4) (1984) 745–752.
D. P. Zhang, Y. J. Lei and Z. B. Shen, Thermo-electromechanical vibration analysis of piezoelectric nanoplates resting on viscoelastic foundation with various boundary conditions, International Journal of Mechanical Sciences, 131–132 (2017) 1001–1015.
S. Zeng, B. L. Wang and K. F. Wang, Analyses of natural frequency and electromechanical behavior of flexoelectric cylindrical nanoshells under modified couple stress theory, Journal of Vibration and Control, 25(3) (2019) 559–570.
C. W. Lim, Y. F. Ma, S. Kitipornchai, C. M. Wang and R. K. K. Yuen, Buckling of vertical cylindrical shells under combined end pressure and body force, Journal of Engineering Mechanics, 129(8) (2003) 876–884.
X. Q. Fang and C. S. Zhu, Size-dependent nonlinear vibration of nonhomogeneous shell embedded with a piezoelectric layer based on surface/interface theory, Composite Structures, 160 (2017) 1191–1197.
A. Alibeigloo and M. Shaban, Free vibration analysis of carbon nanotubes by using three-dimensional theory of elasticity, Acta Mechanica, 224(7) (2013) 1415–1427.
H. Zeighampour and Y. T. Beni, A shear deformable cylindrical shell model based on couple stress theory, Archive of Applied Mechanics, 85(4) (2015) 539–553.
Y. W. Wang, K. Xie, T. R. Fu and W. Zhang, A unified modified couple stress model for size-dependent free vibrations of FG cylindrical microshells based on high-order shear deformation theory, The European Physical Journal Plus, 135(1) (2020) 1–19.
Acknowledgments
This research is supported by the National Natural Science Foundation of China (Grant No. 51965042).
Author information
Authors and Affiliations
Corresponding author
Additional information
Wenguang Liu received his Ph.D. degree from Nanjing University of Aeronautics and Astronautics, China. He is currently working as an Associate Professor in Nanchang Hangkong University, China.
Zhipeng Lyu is currently studying as a graduating student in Nanchang Hangkong University, China. His research interests include multi-field coupling dynamics of piezoelectric structures.
Rights and permissions
About this article
Cite this article
Lyu, Z., Liu, W., Liu, C. et al. Thermo-electro-mechanical vibration and buckling analysis of a functionally graded piezoelectric porous cylindrical microshell. J Mech Sci Technol 35, 4655–4672 (2021). https://doi.org/10.1007/s12206-021-0933-1
Received:
Revised:
Accepted:
Published:
Issue Date:
DOI: https://doi.org/10.1007/s12206-021-0933-1