Abstract
Fluorosis can induce neurotoxicity. Sodium butyrate (SB), a histone deacetylase inhibitor, has important research potential in correcting glucose metabolism disorders and is widely used in a variety of neurological diseases and metabolic diseases, but it is not yet known whether it plays a role in combating fluoride-induced neurotoxicity. This study aims to evaluate the effect of SB on fluoride neurotoxicity and the possible associated mechanisms. The results of HE staining and Morris water maze showed that, in mice exposed to 100 mg/L fluoride for 3 months, the hippocampal cells arranged in loosely with large cell gaps and diminished in number. One thousand milligram per kilogram per day SB treatment improved fluoride-induced neuronal cell damage and spatial learning memory impairment. Western blot results showed that the abundance of malate dehydrogenase 2 (MDH2) and pyruvate dehydrogenase (PDH) in the hippocampus of fluorosis mice was increased, the abundance of pyruvate kinase M (PKM), lactate dehydrogenase (LDH), hexokinase (HK), phosphatidylinositol 3-kinase (PI3K), phosphorylated Akt (P-AKT), and hypoxia-inducible factor 1α (HIF-1α) was inhibited, and the content of lactate and ATP was decreased. SB treatment reversed the decreased glycolysis in the hippocampus of fluorosis mice. These results suggested that SB could ameliorate fluorosis-induced neurotoxicity, which might be linked with its function in regulating glycolysis as well as inhibition of the PI3K/AKT/HIF-1α pathway.
Graphical Abstract
Sodium butyrate ameliorates fluorosis-induced neurotoxicity by regulating hippocampal glycolysis in vivo (created with MedPeer (www.medpeer.cn))









Similar content being viewed by others
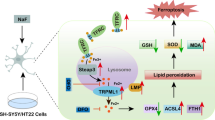
Data Availability
The datasets generated during and/or analyzed during the current study are available from the corresponding author on reasonable request.
References
Zheng D, Liu Y, Luo L, Shahid MZ, Hou D (2020) Spatial variation and health risk assessment of fluoride in drinking water in the Chongqing urban areas, China. Environ Geochem Health 42:2925–2941. https://doi.org/10.1007/s10653-020-00532-3
Dall Agnol MA, Battiston C, Tenuta LMA, Cury JA (2022) Fluoride formed on enamel by fluoride varnish or gel application: a randomized controlled clinical trial. Caries Res 56:73–80. https://doi.org/10.1159/000521454
Li X, Yang J, Liang C, Yang W, Zhu Q, Luo H, Liu X, Wang J, Zhang J (2022) Potential protective effect of riboflavin against pathological changes in the main organs of male mice induced by fluoride exposure. Biol Trace Elem Res 200:1262–1273. https://doi.org/10.1007/s12011-021-02746-7
Lopes GO, Martins Ferreira MK, Davis L, Bittencourt LO, Bragança Aragão WA, Dionizio A, Rabelo Buzalaf MA, Crespo-Lopez ME, Maia CSF, Lima RR (2020) Effects of fluoride long-term exposure over the cerebellum: global proteomic profile, oxidative biochemistry, cell density, and motor behavior evaluation. Int J Mol Sci 21. https://doi.org/10.3390/ijms21197297
Łukomska A, Baranowska-Bosiacka I, Dec K, Pilutin A, Tarnowski M, Jakubczyk K, Żwierełło W, Skórka-Majewicz M, Chlubek D, Gutowska I (2020) Changes in gene and protein expression of metalloproteinase-2 and -9 and their inhibitors TIMP2 and TIMP3 in different parts of fluoride-exposed rat brain. Int J Mol Sci 22. https://doi.org/10.3390/ijms22010391
Saeed M, Malik RN, Kamal A (2020) Fluorosis and cognitive development among children (6-14 years of age) in the endemic areas of the world: a review and critical analysis. Environ Sci Pollut Res Int 27:2566–2579. https://doi.org/10.1007/s11356-019-06938-6
Ran LY, Xiang J, Zeng XX, Tang JL, Dong YT, Zhang F, Yu WF, Qi XL, Xiao Y, Zou J, Deng J, Guan ZZ (2021) Integrated transcriptomic and proteomic analysis indicated that neurotoxicity of rats with chronic fluorosis may be in mechanism involved in the changed cholinergic pathway and oxidative stress. Journal of trace elements in medicine and biology : organ of the Society for Minerals and Trace Elements (GMS) 64:126688. https://doi.org/10.1016/j.jtemb.2020.126688
Nadei OV, Khvorova IA, Agalakova NI (2020) Cognitive decline of rats with chronic fluorosis is associated with alterations in hippocampal calpain signaling. Biol Trace Elem Res 197:495–506. https://doi.org/10.1007/s12011-019-01993-z
Jiang P, Li G, Zhou X, Wang C, Qiao Y, Liao D, Shi D (2019) Chronic fluoride exposure induces neuronal apoptosis and impairs neurogenesis and synaptic plasticity: role of GSK-3β/β-catenin pathway. Chemosphere 214:430–435. https://doi.org/10.1016/j.chemosphere.2018.09.095
Beard E, Lengacher S, Dias S, Magistretti PJ, Finsterwald C (2021) Astrocytes as key regulators of brain energy metabolism: new therapeutic perspectives. Front Physiol 12:825816. https://doi.org/10.3389/fphys.2021.825816
Yamagata K (2022) Lactate supply from astrocytes to neurons and its role in ischemic stroke-induced neurodegeneration. Neuroscience 481:219–231. https://doi.org/10.1016/j.neuroscience.2021.11.035
Pan SM, Pan Y, Tang YL, Zuo N, Zhang YX, Jia KK, Kong LD (2022) Thioredoxin interacting protein drives astrocytic glucose hypometabolism in corticosterone-induced depressive state. J Neurochem 161:84–100. https://doi.org/10.1111/jnc.15489
Schurr A, Passarella S (2022) Aerobic glycolysis: a DeOxymoron of (Neuro)Biology. Metabolites 12. https://doi.org/10.3390/metabo12010072
Zheng J, Xie Y, Ren L, Qi L, Wu L, Pan X, Zhou J, Chen Z, Liu L (2021) GLP-1 improves the supportive ability of astrocytes to neurons by promoting aerobic glycolysis in Alzheimer’s disease. Mol Metabol 47:101180. https://doi.org/10.1016/j.molmet.2021.101180
Cunnane SC, Trushina E, Morland C, Prigione A, Casadesus G, Andrews ZB, Beal MF, Bergersen LH, Brinton RD, De La Monte S, Eckert A, Harvey J, Jeggo R, Jhamandas JH, Kann O, La Cour CM, Martin WF, Mithieux G, Moreira PI et al (2020) Brain energy rescue: an emerging therapeutic concept for neurodegenerative disorders of ageing. Nat Rev Drug Discov 19:609–633. https://doi.org/10.1038/s41573-020-0072-x
Xing G, Ren M, Watson WD, O’neill JT, Verma A (2009) Traumatic brain injury-induced expression and phosphorylation of pyruvate dehydrogenase: a mechanism of dysregulated glucose metabolism. Neurosci Lett 454:38-42. https://doi.org/10.1016/j.neulet.2009.01.047
Lazzarino G, Amorini AM, Signoretti S, Musumeci G, Lazzarino G, Caruso G, Pastore FS, Di Pietro V, Tavazzi B, Belli A (2019) Pyruvate dehydrogenase and tricarboxylic acid cycle enzymes are sensitive targets of traumatic brain injury induced metabolic derangement. Int J Mol Sci 20. https://doi.org/10.3390/ijms20225774
Solano Fonseca R, Metang P, Egge N, Liu Y, Zuurbier KR, Sivaprakasam K, Shirazi S, Chuah A, Arneaud SL, Konopka G, Qian D, Douglas PM (2021) Glycolytic preconditioning in astrocytes mitigates trauma-induced neurodegeneration. eLife 10. https://doi.org/10.7554/eLife.69438
Wang C, Zheng D, Weng F, Jin Y, He L (2022) Sodium butyrate ameliorates the cognitive impairment of Alzheimer’s disease by regulating the metabolism of astrocytes. Psychopharmacology 239:215–227. https://doi.org/10.1007/s00213-021-06025-0
Stilling RM, Van De Wouw M, Clarke G, Stanton C, Dinan TG, Cryan JF (2016) The neuropharmacology of butyrate: the bread and butter of the microbiota-gut-brain axis? Neurochem Int 99:110–132. https://doi.org/10.1016/j.neuint.2016.06.011
Campos-Perez W, Martinez-Lopez E (2021) Effects of short chain fatty acids on metabolic and inflammatory processes in human health. Biochimica et biophysica acta. Mol Cell Biol Lipids 1866:158900. https://doi.org/10.1016/j.bbalip.2021.158900
Angoa-Pérez M, Kuhn DM (2021) Evidence for modulation of substance use disorders by the gut microbiome: hidden in plain sight. Pharmacol Rev 73:571–596. https://doi.org/10.1124/pharmrev.120.000144
Tran SM, Mohajeri MH (2021) The role of gut bacterial metabolites in brain development, aging and disease. Nutrients 13. https://doi.org/10.3390/nu13030732
Jaworska J, Zalewska T, Sypecka J, Ziemka-Nalecz M (2019) Effect of the HDAC inhibitor, sodium butyrate, on neurogenesis in a rat model of neonatal hypoxia-ischemia: potential mechanism of action. Mol Neurobiol 56:6341–6370. https://doi.org/10.1007/s12035-019-1518-1
Jiang Y, Li K, Li X, Xu L, Yang Z (2021) Sodium butyrate ameliorates the impairment of synaptic plasticity by inhibiting the neuroinflammation in 5XFAD mice. Chem Biol Interact 341:109452. https://doi.org/10.1016/j.cbi.2021.109452
Wang H, Song W, Wu Q, Gao X, Li J, Tan C, Zhou H, Zhu J, He Y, Yin J (2021) Fecal transplantation from db/db mice treated with sodium butyrate attenuates ischemic stroke injury. Microbiol Spectr 9:e0004221. https://doi.org/10.1128/Spectrum.00042-21
Nitulescu GM, Van De Venter M, Nitulescu G, Ungurianu A, Juzenas P, Peng Q, Olaru OT, Grădinaru D, Tsatsakis A, Tsoukalas D, Spandidos DA, Margina D (2018) The Akt pathway in oncology therapy and beyond (Review). Int J Oncol 53:2319–2331. https://doi.org/10.3892/ijo.2018.4597
Sun LT, Zhang LY, Shan FY, Shen MH, Ruan SM (2021) Jiedu Sangen decoction inhibits chemoresistance to 5-fluorouracil of colorectal cancer cells by suppressing glycolysis via PI3K/AKT/HIF-1α signaling pathway. Chin J Nat Med 19:143–152. https://doi.org/10.1016/s1875-5364(21)60015-8
Duan F, Mei C, Yang L, Zheng J, Lu H, Xia Y, Hsu S, Liang H, Hong L (2020) Vitamin K2 promotes PI3K/AKT/HIF-1α-mediated glycolysis that leads to AMPK-dependent autophagic cell death in bladder cancer cells. Sci Rep 10:7714. https://doi.org/10.1038/s41598-020-64880-x
Wang P, Cong M, Liu T, Li Y, Liu L, Sun S, Sun L, Zhu Z, Ma H, You H, Zhang H, Jia J (2020) FoxA2 inhibits the proliferation of hepatic progenitor cells by reducing PI3K/Akt/HK2-mediated glycolysis. J Cell Physiol 235:9524–9537. https://doi.org/10.1002/jcp.29759
Niu R, Chen H, Manthari RK, Sun Z, Wang J, Zhang J, Wang J (2018) Effects of fluoride on synapse morphology and myelin damage in mouse hippocampus. Chemosphere 194:628–633. https://doi.org/10.1016/j.chemosphere.2017.12.027
Xin J, Zeng D, Wang H, Sun N, Khalique A, Zhao Y, Wu L, Pan K, Jing B, Ni X (2020) Lactobacillus johnsonii BS15 improves intestinal environment against fluoride-induced memory impairment in mice-a study based on the gut-brain axis hypothesis. PeerJ 8:e10125. https://doi.org/10.7717/peerj.10125
Wan G, Yu G, Xu C, Wang S, Zhang Z, Zhang L, Ye P, Bian C, Zhao X, Song L, Shi Y, Liu Z (2001) Determination for fluoride in serum - ion selective electrode method. Standards Press of China, Beijing
Yu G, Bian J, Wang W, Ji X, Zhu L, Yang X, Ji X (2015) Determination for fluoride in urine - Ion selective electrode method. Standards Press of China, Beijing
Qiao L, Liu X, He Y, Zhang J, Huang H, Bian W, Chilufya MM, Zhao Y, Han J (2021) Progress of signaling pathways, stress pathways and epigenetics in the pathogenesis of skeletal fluorosis. Int J Mol Sci 22. https://doi.org/10.3390/ijms222111932
Guth S, Hüser S, Roth A, Degen G, Diel P, Edlund K, Eisenbrand G, Engel KH, Epe B, Grune T, Heinz V, Henle T, Humpf HU, Jäger H, Joost HG, Kulling SE, Lampen A, Mally A, Marchan R et al (2020) Toxicity of fluoride: critical evaluation of evidence for human developmental neurotoxicity in epidemiological studies, animal experiments and in vitro analyses. Arch Toxicol 94:1375–1415. https://doi.org/10.1007/s00204-020-02725-2
Grandjean P (2019) Developmental fluoride neurotoxicity: an updated review. Environ Health 18:110. https://doi.org/10.1186/s12940-019-0551-x
Ning H, Li C, Yin Z, Hu D, Ge Y, Chen L (2021) Fluoride exposure decreased neurite formation on cerebral cortical neurons of SD rats in vitro. Environ Sci Pollut Res Int 28:50975–50982. https://doi.org/10.1007/s11356-021-13950-2
Kitahara M, Inoue T, Mani H, Takamatsu Y, Ikegami R, Tohyama H, Maejima H (2021) Exercise and pharmacological inhibition of histone deacetylase improves cognitive function accompanied by an increase of gene expressions crucial for neuronal plasticity in the hippocampus. Neurosci Lett 749:135749. https://doi.org/10.1016/j.neulet.2021.135749
Sun J, Yuan B, Wu Y, Gong Y, Guo W, Fu S, Luan Y, Wang W (2020) Sodium butyrate protects N2a cells against Aβ toxicity in vitro. Mediators Inflamm 2020:7605160. https://doi.org/10.1155/2020/7605160
Zeng XX, Deng J, Xiang J, Dong YT, Cao K, Liu XH, Chen D, Ran LY, Yang Y, Guan ZZ (2020) Protections against toxicity in the brains of rat with chronic fluorosis and primary neurons exposed to fluoride by resveratrol involves nicotinic acetylcholine receptors. J Trace Elem Med Biol 60:126475. https://doi.org/10.1016/j.jtemb.2020.126475
Sacks D, Baxter B, Campbell BCV, Carpenter JS, Cognard C, Dippel D, Eesa M, Fischer U, Hausegger K, Hirsch JA, Shazam Hussain M, Jansen O, Jayaraman MV, Khalessi AA, Kluck BW, Lavine S, Meyers PM, Ramee S, Rüfenacht DA et al (2018) Multisociety consensus quality improvement revised consensus statement for endovascular therapy of acute ischemic stroke. Int J Stroke 13:612–632. https://doi.org/10.1177/1747493018778713
Fernando W, Martins IJ, Morici M, Bharadwaj P, Rainey-Smith SR, Lim WLF, Martins RN (2020) Sodium butyrate reduces brain amyloid-β levels and improves cognitive memory performance in an Alzheimer’s disease transgenic mouse model at an early disease stage. J Alzheimers Dis 74:91–99. https://doi.org/10.3233/jad-190120
Badejogbin C, Areola DE, Olaniyi KS, Adeyanju OA, Adeosun IO (2019) Sodium butyrate recovers high-fat diet-fed female Wistar rats from glucose dysmetabolism and uric acid-associated cardiac tissue damage. Naunyn Schmiedebergs Arch Pharmacol 392:1411–1419. https://doi.org/10.1007/s00210-019-01679-2
Amoêdo ND, Rodrigues MF, Pezzuto P, Galina A, Da Costa RM, De Almeida FC, El-Bacha T, Rumjanek FD (2011) Energy metabolism in H460 lung cancer cells: effects of histone deacetylase inhibitors. PLoS One 6:e22264. https://doi.org/10.1371/journal.pone.0022264
Zhou T, Xu H, Cheng X, He Y, Ren Q, Li D, Xie Y, Gao C, Zhang Y, Sun X, Xu Y, Huang W (2022) Sodium butyrate attenuates diabetic kidney disease partially via histone butyrylation modification. Mediators Inflamm 2022:7643322. https://doi.org/10.1155/2022/7643322
Yellen G (2018) Fueling thought: management of glycolysis and oxidative phosphorylation in neuronal metabolism. J Cell Biol 217:2235–2246. https://doi.org/10.1083/jcb.201803152
Bell SM, Burgess T, Lee J, Blackburn DJ, Allen SP, Mortiboys H (2020) Peripheral glycolysis in neurodegenerative diseases. Int J Mol Sci 21. https://doi.org/10.3390/ijms21238924
Alberini CM, Cruz E, Descalzi G, Bessières B, Gao V (2018) Astrocyte glycogen and lactate: new insights into learning and memory mechanisms. Glia 66:1244–1262. https://doi.org/10.1002/glia.23250
Gray LR, Tompkins SC, Taylor EB (2014) Regulation of pyruvate metabolism and human disease. Cell Mol Life Sci 71:2577–2604. https://doi.org/10.1007/s00018-013-1539-2
Harris RA, Tindale L, Lone A, Singh O, Macauley SL, Stanley M, Holtzman DM, Bartha R, Cumming RC (2016) Aerobic glycolysis in the frontal cortex correlates with memory performance in wild-type mice but not the APP/PS1 mouse model of cerebral amyloidosis. J Neurosci 36:1871–1878. https://doi.org/10.1523/jneurosci.3131-15.2016
Yang SQ, Tian Q, Li D, He SQ, Hu M, Liu SY, Zou W, Chen YJ, Zhang P, Tang XQ (2020) Leptin mediates protection of hydrogen sulfide against 6-hydroxydopamine-induced Parkinson’s disease: Involving enhancement in Warburg effect. Neurochem Int 135:104692. https://doi.org/10.1016/j.neuint.2020.104692
Chin YR, Toker A (2009) Function of Akt/PKB signaling to cell motility, invasion and the tumor stroma in cancer. Cell Signal 21:470–476. https://doi.org/10.1016/j.cellsig.2008.11.015
Robey RB, Hay N (2009) Is Akt the “Warburg kinase”?-Akt-energy metabolism interactions and oncogenesis. Semin Cancer Biol 19:25–31. https://doi.org/10.1016/j.semcancer.2008.11.010
Kim JW, Tchernyshyov I, Semenza GL, Dang CV (2006) HIF-1-mediated expression of pyruvate dehydrogenase kinase: a metabolic switch required for cellular adaptation to hypoxia. Cell Metab 3:177–185. https://doi.org/10.1016/j.cmet.2006.02.002
Marín-Hernández A, Gallardo-Pérez JC, Ralph SJ, Rodríguez-Enríquez S, Moreno-Sánchez R (2009) HIF-1alpha modulates energy metabolism in cancer cells by inducing over-expression of specific glycolytic isoforms. Mini Rev Med Chem 9:1084–1101. https://doi.org/10.2174/138955709788922610
Sun R, Meng X, Pu Y, Sun F, Man Z, Zhang J, Yin L, Pu Y (2019) Overexpression of HIF-1a could partially protect K562 cells from 1,4-benzoquinone induced toxicity by inhibiting ROS, apoptosis and enhancing glycolysis. Toxicol In Vitro 55:18–23. https://doi.org/10.1016/j.tiv.2018.11.005
Zhou Z, Xu N, Matei N, Mcbride DW, Ding Y, Liang H, Tang J, Zhang JH (2021) Sodium butyrate attenuated neuronal apoptosis via GPR41/Gβγ/PI3K/Akt pathway after MCAO in rats. J Cereb Blood Flow Metab 41:267–281. https://doi.org/10.1177/0271678x20910533
Funding
This work was supported by grants from the National Natural Science Foundation of China (NSFC) (no. 81803200andno. 81803180), Liaoning Province Key Research and Development Program Guidance Plan (no. 2019JH8/10300047), Shenyang Science and Technology Plan Project (no. 21-108-9-11), Shenyang Middleyounger Scientific and Technological Innovation Support Plan (no. RC200238), Project of Education Department of Liaoning Province (no. SYYX202008), Youth Foundation of Liaoning Education Department (no. LJKQZ2021173), Science and Technology Innovation Fund for Postgraduates of Shenyang Medical College (no. Y20210503), and Natural Science Foundation of Liaoning Province (2022-NLTS-14-06).
Author information
Authors and Affiliations
Contributions
Y. L.: writing—original draft preparation; N. Y. and Z. W.: conceptualization, methodology, and funding acquisition; X. D. and J. L.: writing—review and editing and supervision; Y. D. and Y. W.: provided experimental method guidance; Y. Y and X. J.: data curation; Q. L.: generated and validated the mouse model.
Corresponding authors
Ethics declarations
Ethics Approval
Animal use has been approved by the Animal Use and Care Committee at Shenyang Medical College (protocol number: SYYXY2021031502).
Consent for Publication
All authors have read and agreed to the published version of the manuscript.
Conflict of Interest
The authors declare no competing interests.
Additional information
Publisher’s Note
Springer Nature remains neutral with regard to jurisdictional claims in published maps and institutional affiliations.
Yangjie Li and Zhengdong Wang have contributed to the work equally and should be regarded as co-first authors.
Rights and permissions
Springer Nature or its licensor (e.g. a society or other partner) holds exclusive rights to this article under a publishing agreement with the author(s) or other rightsholder(s); author self-archiving of the accepted manuscript version of this article is solely governed by the terms of such publishing agreement and applicable law.
About this article
Cite this article
Li, Y., Wang, Z., Li, J. et al. Sodium Butyrate Ameliorates Fluorosis-Induced Neurotoxicity by Regulating Hippocampal Glycolysis In Vivo. Biol Trace Elem Res 201, 5230–5241 (2023). https://doi.org/10.1007/s12011-023-03583-6
Received:
Accepted:
Published:
Issue Date:
DOI: https://doi.org/10.1007/s12011-023-03583-6