Abstract
Previously, we found short-term increases in ambient particulate matter (PM) air pollutant concentrations were associated with increased serum fibrinogen levels in patients with cardiac disease. We now studied whether high blood levels of omega-3 (ω-3) fatty acids blunted this fibrinogen response to increased PM concentrations in these same patients. Plasma fibrinogen and ω-3 fatty acid levels (% of total identified fatty acids) were measured in blood samples collected from 135 patients treated at the University of Rochester Medical Center for myocardial infarction or stable ischemic heart disease requiring cardiac catheterization. Using ambient measurements of ultrafine, accumulation mode, and fine particles (PM2.5), Delta-C, and black carbon (BC), we regressed serum fibrinogen levels against pollutant concentrations over the previous 1–96 h, using interaction terms to estimate these associations separately for those with HIGH (> 5.12%) and LOWMED serum levels of ω-3 fatty acid (≤ 5.12%). Each 5.6 μg/m3 increase in PM2.5 concentration in the previous hour was associated with a 3.1% increase in fibrinogen (95% CI = 1.5%, 4.7%) in those subjects with LOWMED total ω-3 fatty acid levels, but only a 0.9% increase (95% CI = − 1.5%, 3.2%) in patients with HIGH total ω-3 fatty acid levels. This same pattern was observed with fish oil-derived docosahexaenoic and eicosapentaenoic acids but not alpha-linolenic (from plant oil or seeds). A similar finding was observed with BC in the prior 24 h, but not other PM. Thus, increased blood levels of fish-based ω-3 fatty acids attenuated increases in fibrinogen associated with short-term increases in ambient PM.
Similar content being viewed by others
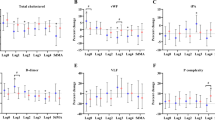
Avoid common mistakes on your manuscript.
Introduction
Particulate air pollution (PM) has repeatedly been associated with an increased risk of acute cardiovascular events like myocardial infarction (Brook et al. 2010). Other studies have reported associations between short-term increases in ambient air pollutants and adverse changes in plasma biomarkers of inflammation and coagulation (Rich et al. 2012a, b; Ruckerl et al. 2007), which are potential mediators of any pollutant effect on these acute cardiovascular events. Specifically, the acute phase reactant fibrinogen has been associated with increases in PM concentrations at lag times of 24–72 h following exposure (Chuang et al. 2007; Rich et al. 2012b) with our prior study showing an association at lag times as short as 1 h (Croft et al. 2017). Biologically, fibrinogen has been observed to increase within 6 h after an inflammatory stimulus with a peak response typically observed at 96 h (Colley et al. 1983).
Previous studies have examined whether intake of omega 3 (ω-3) fatty acids can reduce the risk of acute cardiovascular events and overall risk of cardiovascular disease (Harris et al. 2008). ω-3 fatty acids include eicosapentaenoic acid (EPA) and docosahexaenoic acid (DHA), which are obtained from marine sources such as fish, and alpha-linolenic acid (ALA), a plant-derived fatty acid present in the oils of seeds, nuts, and beans (Harris et al. 2008). Docosapentaenoic acid (DPA) is an ω-3 fatty acid intermediary in the metabolism of EPA to DHA and is generally found in lower concentrations than either of these other fatty acids in foods, with the exception of human milk (Byelashov et al. 2015). Adequate DHA, EPA, and ALA blood concentrations have all been shown to be protective for cardiovascular disease (Chaddha and Eagle 2015; Mozaffarian and Wu 2011) including acute coronary syndrome (ACS) events (de Oliveira Otto et al. 2013). While the cardioprotective effects of ω-3 fatty acids remain an active area of research, the American Heart Association currently states that supplementation with DHA and EPA is reasonable for patients with recent MI or heart failure with reduced ejection fraction (Siscovick et al. 2017).
The cardioprotective effects of ω-3 fatty acids are thought to involve a multifactorial mechanism including anti-inflammatory (Chapkin et al. 2009) and anti-platelet effects (Abdolahi et al. 2014; Block et al. 2013; Block et al. 2015). Previous studies have shown that fish oil supplements (omega-3 fatty acids) blunted ambient air pollutant effects on measures of autonomic dysfunction (i.e., heart rate variability) (Romieu et al. 2005). Also, Tong et al. (2015) observed attenuation of increases in fibrinogen associated with PM in subjects taking fish or olive oil supplements. Our study examines whether specific components of ω-3 fatty acids (whether fish or plant based) can blunt acute ambient air pollutant impacts on fibrinogen in cardiac patients.
Previously, in a panel of patients treated for acute coronary syndrome or stable coronary artery disease in our cardiac catheterization laboratory, we found that increased ambient PM concentrations in the previous 1–48 h were associated with approximately 1–3% increases in plasma fibrinogen levels (Croft et al. 2017). Using these same fibrinogen, pollutant, clinical data, and ω-3 fatty acids measured in these same plasma samples, we hypothesized that in those subjects with lower ω-3 fatty acids levels, we would see increases in fibrinogen associated with acute increases in PM concentrations (1–96 h lags), but little to no increase in fibrinogen associated with increased PM in those subjects with high ω-3 fatty acids levels. We also hypothesized that the fish-based ω-3 fatty acids (EPA and DHA) would modify these same PM/fibrinogen associations, but not the plant-based ω-3 fatty acid (ALA).
Methods
Study population
The study population has been described previously (Croft et al. 2017). Briefly, we included adult (≥ 18 years of age) patients treated at the University of Rochester Medical Center (URMC) Cardiac Catheterization Laboratory, during the winters (November 1st to April 30th) of 2011, 2012, or 2013, for either ST-elevation myocardial infarction (STEMI; n = 25), non-ST elevation myocardial infarction (NSTEMI; n = 32), or stable ischemic heart disease requiring cardiac catheterization (SIHD) (n = 78). After informed consent was received, we atraumatically collected a 30-mL blood sample at the time of cardiac catheterization from each patient.
Biomarkers
Plasma concentrations of fibrinogen (μg/ml) were measured using an enzyme-linked immunoassay (ELISA). The plasma phospholipid fatty acid composition of ω-3 fatty acids (EPA, DHA, ALA, and DPA) was analyzed by gas chromatography (GC) with flame ionization detection, which has similar testing characteristics as the standard thin layer chromatography method (Harris et al. 2007). Plasma concentrations of ω-3 fatty acids were reported as a percent of total identified fatty acids. All study activities were approved by the University of Rochester Research Subjects Review Board.
Air pollution and weather data
Full details of our ambient air pollutant measurement methods have been described previously (Croft et al. 2017). Briefly, hourly pollutant measurements were made at the New York State Department of Environmental Conservation (NYSDEC) site in Rochester, NY, and averaged to hourly concentrations. Particulate matter (PM2.5) was measured continuously using a tapered element oscillating microbalance (TEOM; model 1400ab, ThermoFisher, Franklin, MA). Black carbon (BC) (marker of traffic pollution) was measured using a two-wavelength (370 and 880 nm) aethalometer (Magee Scientific, Inc., Berkeley, CA) with BC measured using the 880-nm wavelength and Delta-C (a marker of wood smoke) calculated as the difference between the BC measured at 370 and 880 nm (Wang et al. 2012). AMP (100–500 nm diameter) and UFP (10–100 nm diameter) were both measured using a 3071 Electrostatic Classifier with a 3010 Condensation Particle Counter (TSI Inc., St. Paul, MN) functioning as a scanning mobility particle sizer (SMPS). Ambient temperature and relative humidity were continuously measured at the same NYSDEC site.
Statistical analysis
For our primary analysis, all pollutant specific analyses described below used those lag times associated with the largest change in fibrinogen in our previous analyses (i.e., BC in the previous 24 h, Delta-C and AMP in the previous 12 h, PM2.5 in the previous 1 h, and UFP in the previous 48 h) (Croft et al. 2017). First, we separated all subjects into tertiles (LOW, MEDIUM, HIGH) based on their ω-3 fatty acids level. Second, using linear regression, we regressed fibrinogen against the mean PM2.5 concentration in the previous 1 h, including indicator variables for ω-3 tertile, interaction terms between ω-3 tertiles and PM2.5 (PM2.5*LOW, PM2.5*MEDIUM), and indicator variables for age (< 50, 50–59, 60–69, 70–79, > 79 years old), year (2011, 2012, or 2013), weekday (weekday versus weekend), hour of the day (04:00–11:59 versus 12:00–03:59), dyslipidemia, prior MI, and smoking (current versus not-current), as well as natural splines (3 degrees of freedom) of temperature (degrees Fahrenheit, prior 24 h), and relative humidity (%, prior 24 h). Except for the indicator variables for ω-3 tertile and the interaction terms, this was the same model used in our previous analyses (Croft et al. 2017). Third, we re-ran the models described above, but with only two ω-3 groups per analysis (i.e., LOW and MEDIUM tertiles combined [LOWMED] versus the HIGH tertile). As a secondary objective, we separately re-ran the same models to examine effect modification by each specific ω-3 fatty acid (DHA, EPA, ALA, and DPA). Finally as a sensitivity analysis, we re-ran the same model for each lagged PM2.5, BC, UFP, AMP, and Delta-C concentration (lag hours 0, 0–11, 0–23, 0–47, 0–71, and 0–95). All analyses were performed using SAS version 9.4 (SAS Institute Inc., Cary, NC) with statistical significance defined as p < 0.05.
Results
The 135 patients in the study were primarily Caucasian (96%), male (73%), over 60 years of age (63%), and overweight or obese (83%), with cardiovascular comorbidities including hypertension (76%) and dyslipidemia (67%) (Table 1). The distributions of pollutant concentrations used in the analysis are shown in Table 2, the distribution of ω-3 fatty acids in Table 3, and the distribution of fibrinogen within ω-3 fatty acids tertiles in Table S1.
After adjusting for covariates, for each 0.29 μg/m3 increase in black carbon concentration lagged 24 h was associated with a 2.6% increase in fibrinogen levels (95% CI = 0.7%, 4.4%) in the LOW total ω-3 tertile and a 2.8% increase (95% CI = 1.3, 4.2) in the MEDIUM ω-3 tertile. However, there was essentially no change in fibrinogen in the HIGH ω-3 tertile (0.2% increase; 95% CI = − 1.7%, 2.1%) (Table 4). Since effect estimates appeared similar for the LOW and MEDIUM tertiles, but different for the HIGH tertile for the main pollutants PM2.5 and BC, we combined the LOW and MEDIUM into one group (LOWMED), with all further analyses based on two groupings (HIGH and LOWMED).
Using these two groups, each 5.6 μg/m3 increase in PM2.5 concentration in the prior 1 h in subjects in the LOWMED ω-3 fatty acid group was associated with a 3.1% increase in fibrinogen levels (95% CI = 1.5%, 4.7%), but only a 0.9% increase (95% CI = − 1.5%, 3.2) in those with HIGH ω-3 fatty acid levels (Table 5). Similarly, each 0.29 μg/m3 increase in black carbon concentration lagged 24 h was associated with a 2.7% increase in fibrinogen levels (95% CI = 1.5%, 3.8%) in the LOWMED ω-3 group. However, there was essentially no change in fibrinogen in the HIGH ω-3 group (0.2% increase; 95% CI = − 1.7%, 2.1%). However, there did not appear to be effect modification of the associations between fibrinogen and AMP, UFP, or Delta-C, as there was little difference in effect estimates between the HIGH and LOWMED ω-3 groups (Table 5).
Next, we examined whether specific ω-3 fatty acid subgroups (i.e., EPA, DHA, DPA, and ALA) each individually modified the fibrinogen/PM associations. Each 5.6 μg/m3 increase in PM2.5 concentration in the previous hour was associated with a 3.96% increase in fibrinogen (95% CI = 2.25%, 5.67%) within the LOWMED DHA group, but essentially no change in the HIGH DHA group (0.15%; 95% CI = − 1.89%, 2.2%) (Table 6 and Fig. 1a). This same pattern of effect modification of fibrinogen was also observed for BC (Table 6 and Fig. 1b). For both PM2.5 and BC, we observed a similar pattern of effect modification of the fibrinogen/pollutant association by EPA and DHA, but not ALA or DPA (Table 6, Fig. 1a, b). Last, we evaluated whether the same effects were noted for all lag times. We observed similar patterns of effect modification of the fibrinogen/PM2.5 1 h association and the fibrinogen/black carbon 24 h association for other PM2.5 and BC averaging/lag times (Table S2 and S3).
Discussion
In patients with active coronary disease (i.e., those experiencing a myocardial infarction or those treated for stable ischemic heart disease in our cardiac catheterization laboratory), we previously found that increases in fibrinogen were associated with increased concentrations of PM air pollution (PM2.5, BC, UFP, and AMP) in the previous 1 to 48 h (Croft et al. 2017).
However, as hypothesized, we observed that increased fibrinogen was associated with increased PM2.5 and black carbon concentrations only in those with LOW/MEDIUM ω-3 fatty acid levels, but there was no effect of either pollutant on fibrinogen when serum ω-3 fatty acid levels were HIGH. Further, this same pattern of effect modification by ω-3 fatty acids was seen across multiple lag times (i.e., lag hours 0, 0–11, 0–23, 0–47, 0–71, and 0–95) for both PM2.5 and BC. Consistent with our hypothesis, this same pattern was observed for two ω-3 fatty acids found in fish oil (EPA and DHA), but not an ω-3 fatty acid derived from plants (ALA).
Previous studies have reported that fish oil ω-3 fatty acid supplements blunted or lessened changes in markers of autonomic dysfunction associated with short-term increases in ambient PM concentrations, including successive normal RR intervals differing by more than 50 ms (pNN50), the root mean square of the sum of the square of differences between adjacent intervals (r-MSSD), and the standard deviation of normal to normal intervals (SDNN) (Romieu et al. 2005; Tong et al. 2012). A recent study reported an attenuation of fibrinogen levels after exposure to concentrated ambient air pollution particles in subjects who underwent 4 months of fish oil supplementation prior to exposure (Tong et al. 2015). Our finding that elevated plasma levels of fibrinogen associated with acutely increased PM concentrations were attenuated by high levels of total ω-3 fatty acids and specifically fish oil-derived EPA and DHA is consistent with these studies. Furthermore, our study results are strengthened by our finding of consistent effect modification by EPA and DHA for multiple pollutants (PM2.5 and BC) at all lag times studied (1–96 h) (Tables S2 and S3). These findings provide additional evidence for fish oil’s mitigation of adverse changes in fibrinogen with an important strength being the measurement of the actual blood levels of specific ω-3 fatty acid components.
Other studies not focused on air pollution have examined whether ω-3 fatty acid supplements reduced plasma fibrinogen concentrations. Lee et al. (2006) reported no change in overall fibrinogen level following 3 months’ treatment with 1 g/day of OMACOR® ω-3 fatty acids, and no change in fibrinogen binding following supplementation with OMACOR® (850–882 mg EPA and DHA ω-3 fatty acids) for 6 weeks (Mackay et al. 2012). Similar to our study, Mackay et al. (2012) also used plasma levels of ω-3 fatty acids, while Lee et al. (2006) relied on supplementation history. Our measuring of blood plasma levels of fatty acids is preferable to relying on dietary or supplement information when studying cardiovascular disease outcomes (Burr et al. 1989; Mozaffarian and Wu 2011), as the high variability in absorption makes extrapolating blood levels from survey-based methods less reliable (Superko et al. 2013).
Blunting or lessening of PM/fibrinogen associations by EPA and DHA may be related to their anti-inflammatory properties (Chapkin et al. 2009). The general mechanism of EPA and DHA’s anti-inflammatory effect likely involves the attenuation of cyclooxygenase (COX) activity, which normally promotes thromboxane production, perpetuating inflammation and thrombosis (Im 2016). More specifically, EPA and DHA produce resolvins which act via inhibition of the interleukin IL-1β while DHA produces two additional anti-inflammatory specialized pro-resolving mediators, protectins, and maresins (Calder 2015). Protectins inhibit tumor necrosis factor alpha (TNF-α) and interleukin-1-beta (IL-1β) production (Calder 2015) and maresins inhibit leukotriene A4 hydrolase (LTA4H) (Serhan et al. 2015). Though ALA is associated with reductions in some of the similar anti-inflammatory cells as DHA and EPA (i.e., IL-6), the plant-based ALA and fish oil-based DHA and EPA are suspected to act by independent mechanistic pathways (Anderson and Ma 2009). Also, though our study did not show effect modification of DPA on the adverse changes in fibrinogen, there is a growing literature on DPA’s (intermediary ω-3 fatty acid between EPA and DHA) cardiovascular benefit, thought to be through anti-inflammatory pathways and beneficial lipid and platelet effects (Byelashov et al. 2015). Thus, it is possible that our finding of effect modification of PM-mediated inflammation by DHA and EPA, but not DPA or the predominantly plant-based ALA, is due to modification of an inflammatory pathway unique to EPA and DHA. Elucidating the respective mechanisms of fish and plant-based ω-3 fatty acids on PM-mediated inflammation is an important area of future research.
It is unclear why ω-3 fatty acids modified associations between fibrinogen and PM2.5 and BC, but not fibrinogen associations with UFP, AMP, and Delta-C. Whether a unique inflammatory pathway in response to PM2.5 and BC is particularly susceptible to effect modification by omega-3 fatty acids has not been studied. Future studies using larger populations and more spatially resolved pollutant concentrations (e.g., land use regression estimated concentrations at each subject’s residence) or even personal measurements may provide a clearer understanding of this issue.
Although our plasma measurements of ω-3 fatty acids at the same time as our fibrinogen biomarker measurements and matched pollution data were strengths of this study, there were also several limitations. First, our limited sample size (n = 135) likely resulted in reduced statistical power. However, inference on effect modification of the PM/fibrinogen association by ω-3 fatty acids was primarily made by considering whether there were similar patterns of effect modification across multiple pollutants (e.g., PM2.5 and BC), multiple lag times (lag hours 0, 0–11, 0–23, 0–47, 0–71, 0–96), and multiple ω-3 fatty acid subgroups (e.g., EPA, DHA, ALA), and not on whether one individual test was statistically significant. Second, we used ambient air pollution data from a central monitoring station for each subject without the ability to account for the distance of their home or work from the monitoring site, likely resulting in non-differential exposure misclassification and underestimates of effects. Third, we were unable to control for differences in socioeconomic status or medication history/usage between subjects, potentially resulting in residual confounding of our comparisons of the fibrinogen/PM associations between subjects in the HIGH and LOWMED ω-3 groups. Fourth, though our use of laboratory measurement of plasma ω-3 levels (and specifically EPA and DHA) provides an accurate assessment of ω-3 levels, we did not have data on regular dietary fish intake or fish oil supplementation history. Without documentation of each subject’s regular ω-3 intake, we cannot determine whether the observed beneficial fish oil-derived ω-3 effect was due to fish oil supplementation, dietary fish intake, or both (Mohebi-Nejad and Bikdeli 2014).
Conclusions
Increased circulating ω-3 fatty acid concentrations appeared to blunt the adverse changes in fibrinogen associated with increased PM2.5 and black carbon concentrations, but not other pollutants. Furthermore, this protective effect appeared to be driven by fish oil-derived ω-3 fatty acids (i.e., EPA and DHA), but not a plant-derived ω-3 fatty acid (ALA). The mechanism(s) of ω-3 fatty acid’s effect modification of the adverse effects of air pollution on fibrinogen, and the potential for regular ω-3 intake or supplementation to block adverse inflammatory effects of air pollution in populations regularly exposed to air pollution deserves further study.
References
Abdolahi A, Georas SN, Brenna JT, Cai X, Thevenet-Morrison K, Phipps RP et al (2014) The effects of aspirin and fish oil consumption on lysophosphatidylcholines and lysophosphatidic acids and their correlates with platelet aggregation in adults with diabetes mellitus. Prostaglandins Leukot Essent Fatty Acids 90:61–68
Anderson BM, Ma DW (2009) Are all n-3 polyunsaturated fatty acids created equal? Lipids Health Dis 8:33
Block RC, Abdolahi A, Smith B, Meednu N, Thevenet-Morrison K, Cai X, Cui H, Mousa S, Thomas Brenna J, Georas S (2013) Effects of low-dose aspirin and fish oil on platelet function and nf-kappab in adults with diabetes mellitus. Prostaglandins Leukot Essent Fatty Acids 89:9–18
Block RC, Abdolahi A, Tu X, Georas SN, Brenna JT, Phipps RP, Lawrence P, Mousa SA (2015) The effects of aspirin on platelet function and lysophosphatidic acids depend on plasma concentrations of epa and dha. Prostaglandins Leukot Essent Fatty Acids 96:17–24
Brook RD, Rajagopalan S, Pope CA 3rd, Brook JR, Bhatnagar A, Diez-Roux AV et al (2010) Particulate matter air pollution and cardiovascular disease: an update to the scientific statement from the American Heart Association. Circulation 121:2331–2378
Burr ML, Fehily AM, Gilbert JF, Rogers S, Holliday RM, Sweetnam PM, Elwood PC, Deadman NM (1989) Effects of changes in fat, fish, and fibre intakes on death and myocardial reinfarction: diet and reinfarction trial (dart). Lancet 2:757–761
Byelashov OA, Sinclair AJ, Kaur G (2015) Dietary sources, current intakes, and nutritional role of omega-3 docosapentaenoic acid. Lipid Technol 27:79–82
Calder PC (2015) Marine omega-3 fatty acids and inflammatory processes: effects, mechanisms and clinical relevance. Biochim Biophys Acta 1851:469–484
Chaddha A, Eagle KA (2015) Cardiology patient page. Omega-3 fatty acids and heart health. Circulation 132:e350–e352
Chapkin RS, Kim W, Lupton JR, McMurray DN (2009) Dietary docosahexaenoic and eicosapentaenoic acid: emerging mediators of inflammation. Prostaglandins Leukot Essent Fatty Acids 81:187–191
Chuang KJ, Chan CC, Su TC, Lee CT, Tang CS (2007) The effect of urban air pollution on inflammation, oxidative stress, coagulation, and autonomic dysfunction in young adults. Am J Respir Crit Care Med 176(4):370–376
Colley CM, Fleck A, Goode AW, Muller BR, Myers MA (1983) Early time course of the acute phase protein response in man. J Clin Pathol 36(2):203–207
Croft DP, Cameron SJ, Morrell CN, Lowenstein CJ, Ling F, Zareba W, Hopke PK, Utell MJ, Thurston SW, Thevenet-Morrison K, Evans KA, Chalupa D, Rich DQ (2017) Associations between ambient wood smoke and other particulate pollutants and biomarkers of systemic inflammation, coagulation and thrombosis in cardiac patients. Environ Res 154:352–361
de Oliveira Otto MC, Wu JH, Baylin A, Vaidya D, Rich SS, Tsai MY et al (2013) Circulating and dietary omega-3 and omega-6 polyunsaturated fatty acids and incidence of cvd in the multi-ethnic study of atherosclerosis. J Am Heart Assoc 2:e000506
Harris WS, Pottala JV, Sands SA, Jones PG (2007) Comparison of the effects of fish and fish-oil capsules on the n 3 fatty acid content of blood cells and plasma phospholipids. Am J Clin Nutr 86:1621–1625
Harris WS, Miller M, Tighe AP, Davidson MH, Schaefer EJ (2008) Omega-3 fatty acids and coronary heart disease risk: clinical and mechanistic perspectives. Atherosclerosis 197:12–24
Im DS (2016) Functions of omega-3 fatty acids and ffa4 (GPR120) in macrophages. Eur J Pharmacol 785:36–43
Lee KW, Blann AD, Lip GY (2006) Effects of omega-3 polyunsaturated fatty acids on plasma indices of thrombogenesis and inflammation in patients post-myocardial infarction. Thromb Res 118:305–312
Mackay I, Ford I, Thies F, Fielding S, Bachoo P, Brittenden J (2012) Effect of omega-3 fatty acid supplementation on markers of platelet and endothelial function in patients with peripheral arterial disease. Atherosclerosis 221:514–520
Mohebi-Nejad A, Bikdeli B (2014) Omega-3 supplements and cardiovascular diseases. Tanaffos 13:6–14
Mozaffarian D, Wu JH (2011) Omega-3 fatty acids and cardiovascular disease: effects on risk factors, molecular pathways, and clinical events. J Am Coll Cardiol 58:2047–2067
Rich DQ, Kipen HM, Huang W, Wang G, Wang Y, Zhu P, Ohman-Strickland P, Hu M, Philipp C, Diehl SR, Lu SE, Tong J, Gong J, Thomas D, Zhu T, Zhang JJ (2012a) Association between changes in air pollution levels during the Beijing Olympics and biomarkers of inflammation and thrombosis in healthy young adults. JAMA 307:2068–2078
Rich DQ, Zareba W, Beckett W, Hopke PK, Oakes D, Frampton MW, Bisognano J, Chalupa D, Bausch J, O’Shea K, Wang Y, Utell MJ (2012b) Are ambient ultrafine, accumulation mode, and fine particles associated with adverse cardiac responses in patients undergoing cardiac rehabilitation? Environ Health Perspect 120(8):1162–1169
Romieu I, Tellez-Rojo MM, Lazo M, Manzano-Patino A, Cortez-Lugo M, Julien P et al (2005) Omega-3 fatty acid prevents heart rate variability reductions associated with particulate matter. Am J Respir Crit Care Med 172:1534–1540
Ruckerl R, Greven S, Ljungman P, Aalto P, Antoniades C, Bellander T et al (2007) Air pollution and inflammation (interleukin-6, c-reactive protein, fibrinogen) in myocardial infarction survivors. Environ Health Perspect 115:1072–1080
Serhan CN, Dalli J, Colas RA, Winkler JW, Chiang N (2015) Protectins and maresins: new pro-resolving families of mediators in acute inflammation and resolution bioactive metabolome. Biochim Biophys Acta 1851:397–413
Siscovick DS, Barringer TA, Fretts AM, Wu JH, Lichtenstein AH, Costello RB et al (2017) Omega-3 polyunsaturated fatty acid (fish oil) supplementation and the prevention of clinical cardiovascular disease: a science advisory from the American Heart Association. Circulation 135:e867–e884
Superko HR, Superko SM, Nasir K, Agatston A, Garrett BC (2013) Omega-3 fatty acid blood levels: clinical significance and controversy. Circulation 128:2154–2161
Tong H, Rappold AG, Diaz-Sanchez D, Steck SE, Berntsen J, Cascio WE, Devlin RB, Samet JM (2012) Omega-3 fatty acid supplementation appears to attenuate particulate air pollution-induced cardiac effects and lipid changes in healthy middle-aged adults. Environ Health Perspect 120:952–957
Tong H, Rappold AG, Caughey M, Hinderliter AL, Bassett M, Montilla T, Case MW, Berntsen J, Bromberg PA, Cascio WE, Diaz-Sanchez D, Devlin RB, Samet JM (2015) Dietary supplementation with olive oil or fish oil and vascular effects of concentrated ambient particulate matter exposure in human volunteers. Environ Health Perspect 123:1173–1179
Wang Y, Hopke PK, Rattigan OV, Chalupa DC, Utell MJ (2012) Multiple-year black carbon measurements and source apportionment using delta-c in Rochester, New York. J Air Waste Manage Assoc 62:880–887
Funding
This work was funded by the New York State Energy Research and Development Authority (contract #32971) and grants from the National Institute of Environmental Health Sciences (Grant #P30 ES001247) and the Bruce and Marsha Moskowitz Foundation Inc. Scott Cameron, Kristin Evans, and Daniel Croft were supported by a National Institutes of Health training grant (T32-HL066988-1). Scott Cameron was also supported by a NIH clinical investigator award K08HL128856. All authors are employed by the University of Rochester Medical Center.
Author information
Authors and Affiliations
Corresponding author
Ethics declarations
Competing interests
The authors declare that they have no competing interests.
Electronic supplementary material
ESM 1
(DOCX 34 kb)
Rights and permissions
Open Access This article is distributed under the terms of the Creative Commons Attribution 4.0 International License (http://creativecommons.org/licenses/by/4.0/), which permits unrestricted use, distribution, and reproduction in any medium, provided you give appropriate credit to the original author(s) and the source, provide a link to the Creative Commons license, and indicate if changes were made.
About this article
Cite this article
Croft, D., Block, R., Cameron, S.J. et al. Do elevated blood levels of omega-3 fatty acids modify effects of particulate air pollutants on fibrinogen?. Air Qual Atmos Health 11, 791–799 (2018). https://doi.org/10.1007/s11869-018-0586-0
Received:
Accepted:
Published:
Issue Date:
DOI: https://doi.org/10.1007/s11869-018-0586-0