Abstract
A C++ algorithm was used to metallurgically design high-performance GMAW electrodes for joining HSLA-65 steel. The electrode design was based on: (1) a carbon content ≤0.06 wt.% for improved weldability, (2) a 5-15% lower Ar3 transformation temperature than HSLA-65 steel for enhanced strength and toughness, and (3) a desirable range of carbon equivalent number (CEN) for consistently overmatching the minimum specified tensile strength of HSLA-65 steel. The algorithm utilized a set of boundary conditions that included calculated Ar3, BS, BF, and MS transformation temperatures besides CEN. Numerical ranges for boundary conditions were derived from chemical compositions of commercial HSLA-65 steel, substituting thermomechanical effects with weld solidification effects. The boundary conditions were applied in evaluating chemical composition ranges of the following three prospective welding electrode specification groups that offered to provide ≤0.06 wt.% carbon, a minimum transverse-weld tensile strength of 552 MPa (80 ksi), and a minimum CVN impact toughness of 27 J at −29 °C through −51 °C (20 ft lbf at −20 °F through −60 °F) in the as-welded condition: (1) ER80S-Ni1, (2) E90C-K3, and (3) E80C-W2. At ≤0.06 wt.% carbon, the algorithm returned over 3100 results for E90C-K3 that satisfied the boundary conditions, but returned no acceptable results for other two electrode specification groups. Results revealed that welding electrode designs based on an Fe-C-Mn-Ni-Mo system, containing 0.06 wt.% C, 1.6 wt.% Mn, 0.8 wt.% Ni, and 0.3 wt.% Mo that provide weld metals characterized by an Ar3 of 690 °C, a CEN of 0.29, and a (BF − MS) of 30 °C are expected to consistently overmatch the minimum specified tensile strength of HSLA-65 steel while offering a minimum CVN impact toughness of 41 J at −40 °C (30 ft lbf at −40 °F).








Similar content being viewed by others
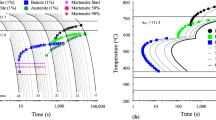
References
K. Sampath, 2006. An Understanding of HSLA-65 Plate Steels Journal of Materials Engineering and Performance 15(1):32–40
ASTM A945/A945M-00, 2000. “Standard Specification for High-Strength Low-Alloy Structural Steel Plate with Low Carbon and Restricted Sulfur for Improved Weldability, Formability, and Toughness,” American Society for Testing & Materials, Philadelphia, PA
P.J. Konkol, J.L. Warren, and P.A. Hebert, 1998. Weldability of HSLA-65 Steel for Ship Structures. Welding Journal, 77(9):361s–371s
A5.28–05, 2005. “Specification for Low-Alloy Steel Electrodes and Rods for Gas Shielded Arc Welding,” American Welding Society, Miami, FL
K. Sampath, 2007. How to Choose Electrodes for Joining High-Strength Steels. Welding Journal, 86(7):26–28
N. Yurioka H. Suzuki S., and Ohshita S. Saito, 1983. Determination of Necessary Preheating Temperature in Steel Welding. Welding Journal, 62(6):147s–153s
C. Ouchi T. Sampei, and I. Kozasu, 1982. The Effect of Hot Rolling Condition and Chemical Composition on the Onset Temperature of Austenite-to-Ferrite Transformation After Hot Rolling. Transactions of the Iron and Steel Institute of Japan, 22:214–222
W. Steven, and A. G. Haynes, 1956. The Temperature of Formation of Martensite and Bainite in Low-Alloy Steels. Journal of the Iron and Steel Institute, 183(8):349–359
K. W. Andrews, 1965. Empirical Formulae for the Calculation of Some Transformation Temperatures. Journal of the Iron and Steel Institute, 203(7):721–727
K. Sampath, 2005. Constraints Based Modeling Enables Successful Development of a Welding Electrode Specification for Critical Navy Applications. Welding Journal, 84(8):131s–138s
K. Sampath, and R. Varadan, 2006. Evaluation of Chemical Composition Limits of GMA Welding Electrode Specifications for HSLA-100 Steel. Welding Journal, 85(8):163s–173s
D.P. Fairchild, J. Koo, N.V. Bangaru, M.L. Macia, D.L. Beeson, and A. Ozekcin, Weld Metals with Superior Low Temperature Toughness for Joining High Strength, Low Alloy Steels, US Patent 6,565,678, May 2003
K. E. Dorschu, and A. Lesnewich, 1964. Development of a Filler Metal for a High-Toughness Alloy Plate Steel with a Minimum Yield Strength of 140 ksi. Welding Journal, 43(12):564s–576s
J. E. Lyttle K. E. Dorschu, and W. A. Fragetta, 1969. Some Metallurgical Characteristics of Tough, High-Strength Welds. Welding Journal, 48(11):493s–498s
R. J. Wong, and M. D. Hayes, 1990. The Metallurgy, Welding & Qualification of Microalloyed (HSLA) Steel Weldments, 450–489. American Welding Society, Miami, FL
P. T. Oldland, C. W. Ramsay, D. K. Matlock, and D. L. Olsen, 1989. Significant Features of High-Strength Steel Weld Metal Microstructures. Welding Journal, 68(4):158s–168s
Welding Handbook, 1989. Kobe Steel, Ltd. Welding Division, Chiyoda-Ku, Tokyo, Japan, p 160–161
http://www.hobartbrothers.com/pdf/datasheets/Metalloy90.pdf, Hobart Brothers Company, Troy, OH
D. Begg, Evaluation of Variable Balance AC Submerged Arc Welding and Metal-cored Electrode Technology for Panel Welding, BMT Fleet Technology Limited, Kanata, ON, Canada, K2K 1Z8, 2006
Navy Ships: Lessons of Prior Programs May Reduce New Attack Submarine Cost Increases and Delays, GAO/NSIAD-95-4, United States General Accounting Office GAO Report to Congressional Requesters, p 7, October 1994
K. Sampath, 2005. Strengthening Materials Specifications. Journal of Materials Engineering and Performance, 14(5):596–603
Author information
Authors and Affiliations
Corresponding author
Appendix A: An Algorithm for Evaluating Chemical Composition Limits of Welding Electrode Specifications Suitable for HSLA-65 Steel in Nonlanguage Specific “Pseudo Code”
Appendix A: An Algorithm for Evaluating Chemical Composition Limits of Welding Electrode Specifications Suitable for HSLA-65 Steel in Nonlanguage Specific “Pseudo Code”
-
1) Define new variables as real numbers for Carbon, Manganese, Nickel, Chromium, and Molybdenum content in wt.% as well as maximum and minimum values.
-
2) Define new variables as real numbers representing mean values of Silicon, Copper, Titanium, Vanadium, Niobium, and Boron content in wt.%.
-
3) Define new variables as real numbers for CEN, Ar3, BS, BF, MS, and BF-MS maximum values, minimum values, and calculated values.
-
4) Assign values for variables based on relevant electrode and base metal specifications.
-
5) Display on screen: “Welding Electrode Composition Evaluation”.
-
6) Display on screen: “Enter integer difference in temperature between calculated BF & calculated MS”.
-
7) Receive value for BF-MS.
-
8) If BF-MS is less than 0 or greater than 50 display on screen “Range incorrect. Enter value between 0 & 50”.
-
9) Else display on screen “filename name to store weld metal chemical compositions. Filename with .csv (comma separated values) would be best for viewing”).
-
10) User enters file name.
-
11) If Filename is NULL display “Error! The file could not be opened”
-
12) Else filename is OK then continue calculations
-
a) Print Carbon, Manganese, Nickel, Chromium, and Molybdenum minimum and maximum wt-%, CEN, Ar3, BS, BF, MS, and BF-MS values to spreadsheet (.csv)
-
b) For loop: Increment C wt-% until it hits the maximum defined.
-
i) For loop: Increment Mn wt-% until it hits the maximum defined.
-
(1) For loop: Increment Ni wt-% until it hits the maximum defined.
-
(a) For loop: Increment Cr wt-% until it hits the maximum defined.
-
(i) For loop: Increment Mo wt-% until it hits the max defined.
-
1. Calculate CEN.
-
2. If CEN is between max and min values
-
a. Calculate Ar3
-
b. If Ar3 is between max and min values
-
i. Calculate BS
-
ii. If BS is between max and min values
-
1. Calculate BF
-
2. If BF is between max and min values
-
(a) Calculate MS
-
(b) If MS is between max and min values
-
(i) If MS + BF-MS is less than BF
-
(1) Print C, Mn, Ni, Cr, Mo, Cu, Si, Ti, Nb, B, V, CEN, Ar3, BS, BF, MS values to spreadsheet
-
-
-
13) Close all statements
Rights and permissions
About this article
Cite this article
Sampath, V., Kehl, J., Vizza, C. et al. Metallurgical Design of High-Performance GMAW Electrodes for Joining HSLA-65 Steel. J. of Materi Eng and Perform 17, 808–819 (2008). https://doi.org/10.1007/s11665-008-9236-2
Received:
Revised:
Accepted:
Published:
Issue Date:
DOI: https://doi.org/10.1007/s11665-008-9236-2