Abstract
In this paper, the autonomous landing control issue on moving shipboard is investigated for unmanned helicopters subject to disturbances. The issue is studied by stabilizing the error system of the helicopter and the shipboard. The landing process is divided into two phases, i.e., homing phase, where a hierarchical double-loop control scheme is developed such that the helicopter is forced to hover synchronously at a certain altitude over the shipboard, and landing phase, where a composite landing control scheme is proposed such that the helicopter lands vertically on the shipboard in synchronization with its attitudes. The velocity and acceleration information of the shipboard as well as lump disturbances is estimated through joint state and disturbance observers. The estimates are then incorporated into the baseline feedback controller, formulating composite active anti-disturbance landing control schemes. A continuous terminal sliding mode control method is proposed for the feedback controller design, which not only effectively mitigates the chattering of the control action, but also simplifies the design process of the controller. Numerical simulations demonstrate the effectiveness and superiorities of the proposed control schemes.












Similar content being viewed by others
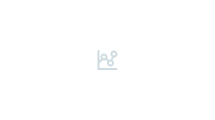
References
Shin, H., You, D., Shim, D.H.: Autonomous shipboard landing algorithm for unmanned helicopters in crosswind. J. Intell. Robot. Syst. 74(1–2), 347–361 (2014)
Jiang, J., Qi, Y., Ibrahim, M., et al.: Quadrotors’ low-cost vision-based autonomous landing architecture on a moving platform. In: 2018 15th International Conference on Control, Automation, Robotics and Vision, Singapore, pp. 448–453 (2018)
Chen, X., Phang, S.K., Shan, M., et al.: System integration of a vision-guided UAV for autonomous landing on moving platform. In: 2016 12th IEEE International Conference on Control and Automation, Kathmandu, Nepal, pp. 761–766 (2016)
Deng, Y., Duan, H.: Control parameter design for automatic carrier landing system via pigeon-inspired optimization. Nonlinear Dyn. 85(1), 97–106 (2016)
Li, J., Duan, H.: Simplified brain storm optimization approach to control parameter optimization in F/A-18 automatic carrier landing system. Aerosp. Sci. Technol. 42, 187–195 (2015)
Ali, U., Shah, M.Z., Samar, R., et al.: Robust level flight control design for scaled Yak-54 unmanned aerial vehicle using single sliding surface. In: 2012 24th Chinese Control and Decision Conference, Taiyuan, China, pp. 1209–1214 (2012)
Hoffmann, G.M., Huang, H., Waslander, S.L., et al.: Precision flight control for a multi-vehicle quadrotor helicopter testbed. Control Eng. Pract. 19(9), 1023–1036 (2011)
Venugopalan, T.K., Taher, T., Barbastathis, G.: Autonomous landing of an unmanned aerial vehicle on an autonomous marine vehicle. In: 2012 Oceans, Yeosu, Korea, pp. 1–9 (2012)
Subrahmanyam, M.B.: H-infinity design of F/A-18A automatic carrier landing system. J. Guid. Control Dyn. 17(1), 187–191 (1994)
Lungu, R., Lungu, M.: Design of automatic landing systems using the H-inf control and the dynamic inversion. J. Dyn. Syst. Meas. Control 138(2), 024501 (2016)
Ghommam, J., Saad, M.: Autonomous landing of a quadrotor on a moving platform. IEEE Trans. Aerosp. Electron. Syst. 53(3), 1504–1519 (2017)
Guan, Z., Ma, Y., Zheng, Z., et al.: Prescribed performance control for automatic carrier landing with disturbance. Nonlinear Dyn. 94(2), 1335–1349 (2018)
Zheng, Z., Jin, Z., Sun, L., et al.: Adaptive sliding mode relative motion control for autonomous carrier landing of fixed-wing unmanned aerial vehicles. IEEE Access 5, 5556–5565 (2017)
Huang, Y., Zhu, M., Zheng, Z., et al.: Fixed-time autonomous shipboard landing control of a helicopter with external disturbances. Aerosp. Sci. Technol. 84, 18–30 (2019)
Rao, D.V., Go, T.H.: Automatic landing system design using sliding mode control. Aerosp. Sci. Technol. 32(1), 180–187 (2014)
Zhao, Z., Yang, J., Li, S., et al.: Continuous output feedback TSM control for uncertain systems with a DC–AC inverter example. IEEE Trans. Circuits Syst. II-Express Briefs 65(1), 71–75 (2018)
Wang, L., Mishra, J., Zhu, Y., et al.: An improved sliding-mode current control of induction machine in presence of voltage constraints. IEEE Trans. Ind. Inform. 16(2), 1182–1191 (2020)
Oh, S.R., Pathak, K., Agrawal, S.K., et al.: Approaches for a tether-guided landing of an autonomous helicopter. IEEE Trans. Robot. 22(3), 536–544 (2016)
Daly, J.M., Ma, Y., Waslander, S.L.: Coordinated landing of a quadrotor on a skid-steered ground vehicle in the presence of time delays. Auton. Robot. 38(2), 179–191 (2015)
Wenzel, K.E., Masselli, A., Zell, A.: Automatic take off, tracking and landing of a miniature UAV on a moving carrier vehicle. J. Intell. Robot. Syst. 61(1–4), 221–238 (2011)
Madonski, R., Ramirez-Neria, M., Gao Z., et al.: Attenuation of periodic disturbances via customized ADRC solution: a case of highly oscillatory 3DOF torsional plant. In: 2019 8th Data Driven Control and Learning System Conference, Dali, China, pp. 1111–1116 (2019)
Wang, X., Yu, X., Li, S., et al.: Composite block backstepping trajectory tracking control for disturbed unmanned helicopters. Aerosp. Sci. Technol. 85, 386–398 (2019)
Yue, Y., Wang, H., Li, N., et al.: Automatic carrier landing system based on active disturbance rejection control with a novel parameters optimizer. Aerosp. Sci. Technol. 69, 149–160 (2017)
Chen, W.H.: Disturbance observer based control for nonlinear systems. IEEE-ASME Trans. Mechatron. 9(4), 706–710 (2004)
Levant, A.: Higher-order sliding modes, differentiation and output-feedback control. Int. J. Control 76(9–10), 924–941 (2003)
Shtessel, Y.B., Shkolnikov, I.A., Levant, A.: Smooth second-order sliding modes: Missile guidance application. Automatica 43(8), 1470–1476 (2007)
Madonski, R., Ramirez-Neria, M., Stanković, M., et al.: On vibration suppression and trajectory tracking in largely uncertain torsional system: an error-based ADRC approach. Mech. Syst. Signal Process. 134(1), 106300 (2019)
Madonski, R., Stanković, M., Shao, S., et al.: Active disturbance rejection control of torsional plant with unknown frequency harmonic disturbance. Control Eng. Pract. 100, 104413 (2020)
Khalil, H.K.: Nonlinear Systems, 3rd edn. Prentice Hall, London (2002)
Hardy, G.H., Littlewood, J.E., Pólya, G., et al.: Inequalities. Cambridge University Press, Cambridge (1952)
Bhat, S.P., Bernstein, D.S.: Finite-time stability of continuous autonomous systems. SIAM J. Control Optim. 38(3), 751–766 (2000)
Bhat, S., Bernstein, D.: Finite-time stability of homogeneous systems. Am. Control Conf. 4(4), 2513–2514 (1997)
Liu, C., Chen, W.H., Andrews, J.: Tracking control of small-scale helicopters using explicit nonlinear MPC augmented with disturbance observers. Control Eng. Pract. 20(3), 258–268 (2012)
Bogdanov, A., Wan, E.A.: State-dependent Riccati equation control for small autonomous helicopters. J. Guid. Control Dyn. 30(1), 47–60 (2007)
Yu, S., Yu, X., Shirinzadeh, B., et al.: Continuous finite-time control for robotic manipulators with terminal sliding mode. Automatica 41(11), 1957–1964 (2005)
Bhat, S.P., Bernstein, D.S.: Continuous finite-time stabilization of the translational and rotational double integrators. IEEE Trans. Autom. Control 43(5), 678–682 (1998)
Fang, X., Wu, A., Shang, Y., et al.: A novel sliding mode controller for small-scale unmanned helicopters with mismatched disturbance. Nonlinear Dyn. 83(1–2), 1053–1068 (2016)
Acknowledgements
The work was supported in part by the National Natural Science Foundation of China under Grants 61973080 and 61973081 and in part by the Shenzhen Science and Technology Innovation Committee (STIC) under Grant JCYJ20190813152603594.
Author information
Authors and Affiliations
Corresponding author
Ethics declarations
Conflict of interest
There is no conflict of interest.
Additional information
Publisher's Note
Springer Nature remains neutral with regard to jurisdictional claims in published maps and institutional affiliations.
Rights and permissions
About this article
Cite this article
Yu, X., Yang, J. & Li, S. Disturbance observer-based autonomous landing control of unmanned helicopters on moving shipboard. Nonlinear Dyn 102, 131–150 (2020). https://doi.org/10.1007/s11071-020-05915-w
Received:
Accepted:
Published:
Issue Date:
DOI: https://doi.org/10.1007/s11071-020-05915-w