Abstract
We report a study of an elastic buckled beam undergoing a contactless magnetic actuation of Laplace type. The beam model is based on the elastica beam theory including the beam extensibility. The Laplace force is produced by an electric current travelling along the beam placed in a magnetic induction. The magnitude of the electric current is the control parameter and by increasing the electric current the beam switches from one stable state to the other one for a given beam end-shortening. The main purpose of the study is to investigate the bistable response, more precisely, the diagram of the electric current as function of the midpoint vertical displacement of the beam according to the magnet location along the elastic beam. The model equations are established and they are numerically solved using an algorithm developed for nonlinear boundary value problem. A second part of the study is devoted to experimental validation of the model and comparisons with the results extracted from the numerical solutions to the model equations. Especially, the comparisons ascertain with good accuracy the approach of the proposed bista-ble beam model. In addition, the influence of the model parameters on the bistable response is clearly identified.






Similar content being viewed by others
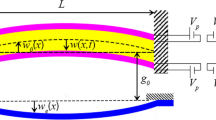
References
Amor A, Fernandes A, Pouget J (2020) Snap-through of elastic bistable beam under contactless magnetic actuation. Int J Non-Linear Mech 119:103358
Buchaillot L, Millet O, Quevy E, Collard D (2008) Post-buckling dynamic behavior of self-assembled 3D microstructures. Microsyst Technol 14:69–78
Casals-Terre J, Fargas-Marques A, Shkel AM (2008) Snap-action bistable micro-mechanisms actuated by nonlinear resonance. J Microelectromech Syst 17:1082–1093
Qiu J, Lang JH, Slocum AH (2004) A curved-beam bistable mechanism. J Microelectromech Syst 13:137–146
Krylov S, Ilic BR, Lulinsky S (2011) Bistability of curved microbeams actuated by fringing electrostatic fields. Nonlinear Dyn 66:403–426
Seunghoon P, Dooyoung H (2008) Pre-shaped buckled-beam actuators: theory and experiments. Sens Actuators A Phys 148:186–192
Saif MTA (2000) On a tunable bistable MEMS: theory and experiment. J Microelectromech Syst 9:157–170
Chen G, Gou Y, Zhang A (2011) Synthesis of compliant multistable mechanisms through use of a single bistable mechanism. J Mech Design 133:081007
Fu S, Ding G, Wang H, Yang Z, Feng J (2007) Design and fabrication of a magnetic bi-stable electromagnetic MEMS relay. Microelectron J 38:556–563
Hafez M (2007) Tactile interfaces: technologies, applications and challenges. Visual Compt 23:267–272
Niu X, Broche P, Stoyanov H, Yu SR, Pei Q (2012) Bistable electroactive polymer for refreshable braille display with improved actuation stability. In: Proc. of SPIE p.83400R1
Roodenburg D, Spronck JW, Van der Zant HSV, Venstra WJ (2009) Buckling beam micromechanical memory with on-chip readout. Appl Phys Lett 94:183501
Camescasse B, Fernandes A, Pouget J (2013) Bistable buckled beam: Elastica modeling and analysis of static actuation. Int J Solids Struct 50:2881–2893
Camescasse B, Fernandes A, Pouget J (2014) Bistable buckled beam and force actuation: experimental validations. Int J Solids Struct 51:1750–1757
Cazottes P, Fernandes A, Pouget J, Hafez M (2008) Design of actuation for bistable structures using smart materials. In: CIMTEC 3rd International Conference on Smart Materials, Structures and Systems, 8–13 June, 2008. Acireale, Sicily, Italy
Cazottes P, Fernandes A, Pouget J, Hafez M (2010) Bistable buckled beam: modeling and actuating force and experimental validations. ASME J Mech Des 131:1001001–1001011
Han JS, Ko JS, Kim YT, Kwak BM (2002) Parametric study and optimization of micro-optical switch with a laterally driven electromagnetic microactuator. J Micromech Microeng 12:39–47
Park S, Hah D (2008) Pre-shaped buckled-beam actuators, Theory and experiment. Sens Actuators A Phys 148:186–192
Stanciulescu I, Mitchell T, Chandra Y, Eason T, Spottswood M (2012) A lower bound on snap-through instability of curved beams under thermomechanical loads. Int J Non-linear Mech 47:561–575
Alcheikh N, Ben Mbarek S, Ouakad HM, Younis MI (2021) A highly sensitive and wide-range resonant magnetic micro-sensor based on a buckled micro-beam. Sens Actuators A Phys 328
Zaidi S, Lamarque F, Prelle C, Carton O, Zeinert A (2012) Contactless and selective energy transfer to a bistable micro-actuation using laser heated shape memory alloy. Smart Mater Struct 21:115027
Zhang Y, Wang Y, Li Z, Huang Y, Li D (2007) Snap-through and pull-in instabilities of an arch-shaped beam under an electrostatic loading. J Microelectromech Syst 16:684–693
Krylov S, Ilic BR, Schreiber D, Saratensky S, Craighead H (2008) The pull-in behavior of electrostatically actuated bistable microstructures. J Micromech Microeng 18:055026-1-055026-20
Das K, Batra RC (2009) Pull-in and snap-through instabilities in transient deformations of microelectromechanical systems. J Micromech Microeng 19:035008-1-035008-19
Younis MI, Ouakad HM, Alsaleem FM, Miles R, Cui W (2010) Nonlinear dynamics of MEMS arches under harmonic electrostatic actuation. J Microelectromech Syst 19:647–656
Aimmanee S, Tichakorn K (2018) Piezoelectrically induced snap-through buckling in a buckled beam bonded with a segmented actuator. J Intell Math Syst Struct
Hafez M, Lichter MD, Dubowsky S (2003) Optimized binary modular reconfigurable robotic devices. Mechatron IEEE/ASME Trans 8:18–25
Palathingal S, Ananthasuresh GK (2019) Analysis and design of fixed-fixed bistable arch-profiles using a bilateral relationship. J Mech Robot 11(3):031002
Hu N, Burgueño R (2015) Buckling-induced smart applications: recent advances and trends. Smart Math Struct 24(6)
Torteman B, Kessler Y, Liberzon A, Krylov S (2019) Electro-thermal excitation of parametric resonances in double-clamped micro beams. Appl Phys Let 115(19)
Hussein H, Khan F, Younis MI (2020) A monolithic tunable symmetric bistable mechanism. Smart Math Struct 29(7)
Xie Z, Kwuimy KCA, Wang Z, Huang W (2018) A piezoelectric energy harvester for broadband rotational excitation using buckled beam. AIP Adv 8(1)
Maugin GA (1988) Continuum mechanics of electromagnetic solids. North-Holland, Series Applied Mathematics and Mechanics, vol 33
Jackson JD (1998) Classical electrodynamics, 3rd edn. Wiley, New York
Love AEH (1944) A Treatise on the Mathematical Theory of Elasticity. Dover Publications, fourth Edition
Frasier CG (1991) Mathematical technique and physical conception in Euler‘s investigation of the elastica. Centaurus 34:211–246
Antman SS (2005) Nonlinear Problems of Elasticity. Applied Mathematical Series 107 (2nd ed.). Springer-Verlag
Magnusson A, Ristinmaa M, Ljung C (2001) Behaviour of the extensible elastica solution. Int J Solids Struct 38:8441–8457
Vangbo M (1998) An analytical analysis of a compressed bistable buckled beam. Sens Actuators A69:212–216
Hussein H, Younis MI (2020) Analytical study of the snap-through and bistability of beams with arbitrarily initial shape. J Mech Robot 12(4)
Nayfeh H, Emam SA (2008) Exact solution and stability of postbuckling configurations of beams. Nonlinear Dyn 54:395–408
Jiao P, Alavi AH, Borchani W, Lajnef N (2017) Small and large deformation models of post-buckled beams under lateral constraints. Math Mech Solids 24(2):386–405
Pinto OC, Gonçalves PB (2002) Active non-linear control of buckling and vibrations of a flexible buckled beam. Chaos Solitons & Fractals 14:227–239
Chandra Y, Stanciulescu I, Virgin LN, Eason TG, Spottswood SM (2013) A numerical investigation of snap-through in a shallow arch-like model. J Sound Vib 332:2532–2548
Tajaddodianfar F, Pishkenari HN, Yazdi MRH, Miandoab EM (2015) Size-dependent bistability of an electrostatically actuated arch NEMS based on strain gradient theory. J Phys D Appl Phys 48(24)
Nayfeh N, Emam SA (2004) On the nonlinear dynamics of a buckled beam subjected to a primary-resonance excitation. Nonlinear Dyn 35:1–17
Acknowledgements
The research work reported in the paper has been supported by the research project BISCELTECH funded by Fonds Unique Interministériel (FUI-APP21).
Author information
Authors and Affiliations
Corresponding author
Ethics declarations
Conflict of interest
The authors declare that they have no conflict of interest.
Additional information
Publisher's Note
Springer Nature remains neutral with regard to jurisdictional claims in published maps and institutional affiliations.
Rights and permissions
About this article
Cite this article
Amor, A., Fernandes, A. & Pouget, J. Numerical and experimental investigations of bistable beam snapping using distributed Laplace force. Meccanica 57, 109–119 (2022). https://doi.org/10.1007/s11012-021-01412-5
Received:
Accepted:
Published:
Issue Date:
DOI: https://doi.org/10.1007/s11012-021-01412-5