Abstract
This research explores the correlation between the structural properties of supramolecular biocomposites and their mechanical strength. Hybrid biocomposites composed of surface-modified hydroxyapatite nano-particles and supramolecular polycaprolactone (SP PCL), were prepared at different compositions, and their mechanical, thermal and viscoelastic properties as well as biodegradability, biocompatibility and cytotoxicity were evaluated in vitro. The results were compared with those for SP PCL/naked hydroxyapatite nano-composites. We show that surface modification of hydroxyapatite nanoparticles resulted in outstanding improvement of tensile strength and modulus up to 3.6 and 2.2-fold, respectively. At above 10 wt% HAp and 20 wt% HApUPy, heterogeneous nano-composites with inferior mechanical properties were obtained. Based on rheological (in steady shear mode) and small/wide angle X-ray scattering measurements, unusual improved mechanical properties were ascribed to the formation of supramolecular clusters around nanoparticles. In-vitro degradation of the supramolecular nano-composites was also studied to investigate the overall product biodegradation as well as toxicity of the degradation product(s).








Similar content being viewed by others
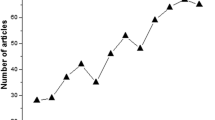
References
Farokhi M, et al. Porous crosslinked poly(ε-caprolactone fumarate)/nanohydroxyapatite composites for bone tissue engineering. J Biomed Mater Res Part A. 2012;100A(4):1051–60.
Kim SS, et al. Poly (lactide-co-glycolide)/hydroxyapatite composite scaffolds for bone tissue engineering. Biomaterials. 2006;27(8):1399–409.
Kunze C, et al. Surface modification of tricalcium phosphate for improvement of the interfacial compatibility with biodegradable polymers. Biomaterials. 2003;24(6):967–74.
Corno M, et al. Hydroxyapatite as a key biomaterial: quantum-mechanical simulation of its surfaces in interaction with biomolecules. Phys Chem Chem Phys. 2010;12(24):6309–29.
Torres N, et al. Stability of antibacterial self-assembled monolayers on hydroxyapatite. Acta Biomater. 2010;6(8):3242–55.
Bach LG, Rafiqul Islam MD, et al. Poly(allyl methacrylate) functionalized hydroxyapatite nanocrystals via a combination of surface initiated RAFT polymerization and tiol ene protocol; a potential anticancer drug carrier. J Colloid Interface Sci. 2013;394:132–40.
Aissa A, et al. Covalent modification of calcium hydroxyapatite surface by grafting phenyl phosphonate moieties. J Solid State Chem. 2007;180(8):2273–8.
Borum-Nicholas L, Wilson O. Surface modification of hydroxyapatite. Part I. Dodecyl alcohol. Biomaterials. 2003;24(21):3671–9.
Borum L, Wilson O. Surface modification of hydroxyapatite. Part II. Silica. Biomaterials. 2003;24(21):3681–8.
Hong Z, et al. Grafting polymerization of L-lactide on the surface of hydroxyapatite nano-crystals. Polymer. 2004;45(19):6699–706.
Qiu X, et al. Surface-modified hydroxyapatite linked by L-lactic acid oligomer in the absence of catalyst. J Polym Sci Part A: Polym Chem. 2005;43(21):5177–85.
Zeng L, et al. A new approach for synthesis of the comb-shaped poly (<i> ε </i>-caprolactone) brushes on the surface of nano-hydroxyapatite by combination of ATRP and ROP. J Colloid Interface Sci. 2010;352(1):36–42.
Wang Y, et al. Improved mechanical properties of hydroxyapatite/poly (ɛ-caprolactone) scaffolds by surface modification of hydroxyapatite. Appl Surf Sci. 2010;256(20):6107–12.
Miltner HE, et al. Quantifying the degree of nanofiller dispersion by advanced thermal analysis: application to polyester nanocomposites prepared by various elaboration methods. J Mater Chem. 2010;20(42):9531–42.
Cui Y, et al. The nanocomposite scaffold of poly (lactide-co-glycolide) and hydroxyapatite surface-grafted with L-lactic acid oligomer for bone repair. Acta Biomater. 2009;5(7):2680–92.
Lee HJ, et al. Modification of hydroxyapatite nanosurfaces for enhanced colloidal stability and improved interfacial adhesion in nanocomposites. Chem Mater. 2006;18(21):5111–8.
Liu A, et al. Surface modification of bioactive glass nanoparticles and the mechanical and biological properties of poly (L-lactide) composites. Acta Biomater. 2008;4(4):1005–15.
Liu Q, de Wijn JR, van Blitterswijk CA. Surface modification of nano-apatite by grafting organic polymer. Biomaterials. 1998;19(11–12):1067–72.
Xie J et al. Controlled biomineralization of electrospun poly(ε-caprolactone) fibers to enhance their mechanical properties. Acta Biomater. 2013;9(3):5698–707.
Keledi G, Hári J, Pukánszky B. Polymer nanocomposites: structure, interaction, and functionality. Nanoscale. 2012;4(6):1919–38.
Jancar J, et al. Current issues in research on structure–property relationships in polymer nanocomposites. Polymer. 2010;51(15):3321–43.
Bhattacharya P et al. Exploiting the physicochemical properties of dendritic polymers for environmental and biological applications. Phys Chem Chem Phys. 2013;15:4477–90.
Zhang W, et al. Correlation of polymer-like solution behaviors with electrospun fiber formation of hydroxypropyl-[small beta]-cyclodextrin and the adsorption study on the fiber. Phys Chem Chem Phys. 2012;14(27):9729–37.
Appel WPJ, Meijer E, Dankers PYW. Enzymatic activity at the surface of biomaterials via supramolecular anchoring of peptides: the effect of material processing. Macromol Biosci. 2011;11(12):1706–12.
Dankers PYW, et al. Bioengineering of living renal membranes consisting of hierarchical, bioactive supramolecular meshes and human tubular cells. Biomaterials. 2011;32(3):723–33.
Li YY, et al. Supramolecular polymer micelles prepared by steric effect tuned self-assembly: a new strategy for self-assembly. Soft Matter. 2011;7(10):4839–44.
Yang C, et al. A supramolecular gene carrier composed of multiple cationic α-cyclodextrins threaded on a PPO–PEO–PPO triblock polymer. Polymer. 2009;50(6):1378–88.
Zhang X, et al. Structure and properties of polysaccharide nanocrystal-doped supramolecular hydrogels based on cyclodextrin inclusion. Polymer. 2010;51(19):4398–407.
Shokrollahi P, et al. Biological and mechanical properties of novel composites based on supramolecular polycaprolactone and functionalized hydroxyapatite. J Biomed Mater Res Part A. 2010;95(1):209–21.
Shokrollahi P, et al. Effect of self-complementary motifs on phase compatibility and material properties in blends of supramolecular polymers. Polymer. 2010;51(26):6303–12.
Mehmanchi M, et al. Supramolecular polycaprolactone nanocomposite based on functionalized hydroxyapatite. J Bioactive Compat Polym. 2012;27(5):467–80.
Chen J, et al. Controlled surface-initiated ring-opening polymerization of L-lactide from risedronate-anchored hydroxyapatite nanocrystals: novel synthesis of biodegradable hydroxyapatite/poly (L-lactide) nanocomposites. J Polym Sci Part A: Polym Chem. 2011;49(20):4379–86.
Tsuji H, Ikada Y. Blends of aliphatic polyesters. II. Hydrolysis of solution-cast blends from poly (L-lactide) and poly (E-caprolactone) in phosphate-buffered solution. J Appl Polym Sci. 1998;67(3):405–15.
Kautz H, et al. Cooperative end-to-end and lateral hydrogen-bonding motifs in supramolecular thermoplastic elastomers. Macromolecules. 2006;39(13):4265.
Qiu X, et al. Hydroxyapatite surface modified by L-lactic acid and its subsequent grafting polymerization of L-lactide. Biomacromolecules. 2005;6(3):1193–9.
Utracki LA, Sepehr MM, Carreau PJ. Rheology of polymers with nanofillers. In: Utracki LA, Jamieson AM, editors. Polymer physics: from suspensions to nanocomposites and beyond. Hoboken, NJ: John Wiley & Sons, Inc.; 2010. p. 639–708.
Ray SS, Okamoto K, Okamoto M. Structure-property relationship in biodegradable poly (butylene succinate)/layered silicate nanocomposites. Macromolecules. 2003;36(7):2355–67.
Ray SS, et al. New polylactide/layered silicate nanocomposites. 3. High-performance biodegradable materials. Chem Mater. 2003;15(7):1456–65.
Kagarise C, et al. Transient shear rheology of carbon nanofiber/polystyrene melt composites. J Nonnewton Fluid Mech. 2010;165(3):98–109.
Wietor JL, et al. Effects of branching and crystallization on rheology of polycaprolactone supramolecular polymers with ureidopyrimidinone end groups. Macromolecules. 2011;44(5):1211.
Van Beek D, et al. Unidirectional dimerization and stacking of ureidopyrimidinone end groups in polycaprolactone supramolecular polymers. Macromolecules. 2007;40(23):8464–75.
Ahn SH, Lee HJ, Kim GH. Polycaprolactone scaffolds fabricated with an advanced electrohydrodynamic direct-printing method for bone tissue regeneration. Biomacromolecules. 2011;12(12):4256–63.
Herbst F, et al. Aggregation and chain dynamics in supramolecular polymers by dynamic rheology: cluster formation and self-aggregation. Macromolecules. 2010;43(23):10006–16.
Woodruff MA, Hutmacher DW. The return of a forgotten polymer—polycaprolactone in the 21st century. Prog Polym Sci. 2010;35(10):1217–56.
Kim WS, et al. Evaluation of mechanical interlock effect on adhesion strength of polymer–metal interfaces using micro-patterned surface topography. Int J Adhes Adhes. 2010;30(6):408–17.
Shen L, Liu T, Lv P. Polishing effect on nanoindentation behavior of nylon 66 and its nanocomposites. Polym Testing. 2005;24(6):746–9.
Shokrollahi P. Supramolecular approaches to materials with tunable biological and mechanical properties: composite and blending. PhD thesis, Cambridge University.
Acknowledgments
We would like to thank Ms. Mohsen Asl Rahimi for his excellent technical assistance and Dr Mahmood Rajabian for a fruitful discussion on rheological measurements.
Author information
Authors and Affiliations
Corresponding author
Rights and permissions
About this article
Cite this article
Shokrollahi, P., Mehmanchi, M., Atai, M. et al. Effect of interface on mechanical properties and biodegradation of PCL HAp supramolecular nano-composites. J Mater Sci: Mater Med 25, 23–35 (2014). https://doi.org/10.1007/s10856-013-5039-6
Received:
Accepted:
Published:
Issue Date:
DOI: https://doi.org/10.1007/s10856-013-5039-6