Abstract
Although silicon and similar bulk materials are widely used in today’s integrated circuits, the transition to lower dimensional structures such as two-dimensional graphene, one-dimensional graphene nanoribbons (GNRs) and silicene nanoribbons (SiNRs) seems inescapable due to the increment of inelastic scattering and related performance degrading effects in bulk circuit components. In this context, GNRs and SiNRs provide advantages such as low area consumption and the adjustment of their electronic behaviours by edge states and widths. On the other hand, rectifiers together with their static and dynamic behaviours constitute the basics of the electronics technology. In this paper, rectifier characteristics of bare-dihydrogenated junctions of GNR and SiNR structures are investigated and compared utilizing first-principles approach. Density functional theory in combination with non-equilibrium Green’s function formalism are used to obtain current–voltage characteristics, transmission eigenstates and dynamic electron densities of the considered GNR and SiNR rectifiers and then these quantities are processed to obtain the dynamical resistance, junction capacitance and time constants of these structures, which is essential for graphene and silicene based electronics design. The paper is concluded with the discussion of the large-signal and small-signal performances of the considered GNR and SiNR rectifiers for commercial integrated circuit applications.





Similar content being viewed by others
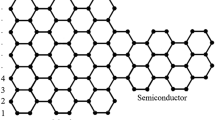
References
Samal, S.K., Peng, Y., Pathak, M., Lim, S.K.: Ultralow power circuit design with subthreshold/near-threshold 3-D IC technologies. IEEE Trans. Compon. Packag. Manuf. Technol. 5(7), 980–990 (2015)
Strawn, G., Strawn, C.: Moore’s law at fifty. IEEE IT Professional 17(6), 69–72 (2015)
Coffey, K.R., Barmak, K., Sun, T., Warren, A.P., Yao, B.: Grain boundary and surface scattering in interconnect metals. In: IEEE International Interconnect Technology Conference, pp. 1–3 (2013)
Olcan, D.I., Petrovich, D.S.: Kolundzica B.M., Comparison of scattering from 2-D and 3-D structures with frequency-dependent materials in time and frequency domains. In: IEEE International Conference on Microwaves, Communications, Antennas and Electronic Systems, pp: 1–5 (2013)
Shen, H., Shi, Y., Wang, X.: Synthesis, charge transport and device applications of graphene nanoribbons. Synth. Met. 210, 109–122 (2015)
Novoselov, K.S., Geim, A.K., Morozov, S.V., Jiang, D., Katsnelson, M.I., Grigorieva, I.V., Dubonos, S.V., Firsov, A.A.: Two-dimensional gas of massless Dirac fermions in graphene. Nature 438, 197–200 (2005)
Jiang, J.-W., Wang, J.-S., Li, B.: Young’s modulus of graphene: a molecular dynamics study. Phys. Rev. B 80, 113405 (2009)
Obrazstsov, A.N.: Chemical vapour deposition: making graphene on a large scale. Nat. Nanotechnol. 4, 212–213 (2009)
Allen, J.A., Tung, V.C., Kaner, R.B.: Honeycomb carbon: a review of graphene. Chem. Rev. 110(1), 132–145 (2009)
Bolotin, K.I., Sikes, K.J., Jiang, Z., Klima, M., Fudenberg, G., Hone, J., Kim, P., Stromer, H.L.: Ultrahigh electron mobility in suspended graphene. Solid State Commun. 146(10), 351–355 (2008)
Chen, Z., Lin, Y.-M., Rooks, M.J., Avouris, Ph: Graphene nano-ribbon electronics. Physica E 40(2), 228–232 (2007)
Yamacli, S.: Extraction of the voltage-dependent quantum capacitance and kinetic inductance of GNRFETs: a first-principles study. J. Comput. Electron. 14(1), 249–256 (2015)
Xue, G., Tang, Q., Tong, Y., Liu, Y.: Size-controlled ambipolar graphene nanoribbon transistors by an all-dry mask method. Synth. Met. 205, 6–10 (2015)
Jang, S.L., Li, S.H.: Gate coupled and zener diode triggering silicon-controlled rectifiers for electrostatic discharge protection circuits. Solid State Electron. 46(2), 263–267 (2002)
Foster, M.P., Stone, D.A.: Describing function model of series resonant inverter with current limiting diode-clamp. Electron. Lett. 47(25), 1363–1364 (2011)
Kimura, Y., Kiso, T., Higaki, T., Sun, Y.: Maemoto T., Sasa S., Inoue M., Rectification effects in ZnO-based transparent self-switching nano-diodes. In: IEEE International Meeting for Future of Electron Devices, pp. 1–2 (2012)
Micheli, G., Leblebici, Y., Gijs, J., Voros, J.: Nanosystems Design and Technology. Springer, New York (2009)
Appenzeller, J., Lin, Y.-M., Konch, J., Avouris, Ph: Band-to-band tunneling in carbon nanotube field-effect transistors. Phys. Rev. Lett. 93, 196805 (2004)
Ilatikhameneh, H., Tan, Y., Novakovic, B., Klimeck, G., Rahman, R., Appenzeller, J.: Tunnel field-effect transistors in 2-D transition metal dichalcogenide materials. IEEE J. Explor. Solid State Comput. Devices Circuits 1, 12–18 (2015)
Fiori, G., Bonaccorso, F., Iannaccone, G., Palacios, T., Neumaier, D., Seabaugh, A., Banerjee, S.K., Colombo, L.: Electronics based on 2-D materials. Nat. Nanotechnol. 9, 768–779 (2014)
Szabo A., Koester S.J., Luisier M.: Metal-dichalcogenide hetero-TFETs: are they a viable option for low power electronics? In: IEEE 72nd Annual Device Research Conference (DRC), pp. 19–20 (2014)
Ilatikhameneh, H., Ameen, T.A., Klimeck, G., Appenzeller, J., Rahman, R.: Deielctric engineered tunnel field effect transistor. IEEE Electron Device Lett. 36(10), 1097–1100 (2015)
Ilatikhameneh, H., Rahman, R., Appenzeller, J., Klimeck, G.: Electrically doped WTe\(_{2}\) transistors. In: IEEE International Conference on Simulation of Semiconductor Processes and Devices (SISPAD), pp: 270–272 (2015)
Fiori, G., Neumaier, D., Szafranek, B.N., Lannaccone, G.: Bilayer graphene transistors for analog electronics. IEEE Trans. Electron Devices 61(3), 729–733 (2014)
Chen, N.L.: Electronic properties of armchair graphene nanoribbons with BN-doping. Solid State Commun. 191, 56–65 (2014)
Zou, D.-Q., Song, Y., Xie, Z., Li, Z.-L., Wang, C.-K.: Large rectification ratio induced by nitrogen (boron) doping in graphene nanoribbon electrodes for OPE junctions. Phys. Lett. A 379, 1842–1846 (2015)
Wang, L.-H., Zhang, Z.-H., Ding, B.-J., Guo, Y.: Size dependence rectification performances induced by boron and nitrogen co-doping in rhombic graphene nanoribbons. Phys. Lett. A 378, 904–908 (2014)
Stander N.: Transport measurements on graphene p-n junctions. Ph.D. Thesis, Stanford University (2010)
An, Y., Wang, K., Yang, Z., Liu, Z., Jia, G., Jiao, Z., Wang, T., Xu, G.: Negative differential resistance and rectification effects in step-like graphene nanoribbons. Org. Electron. 17, 262–269 (2015)
Zhai, X., Jin, G.: Bipolar spin diode based on a bent graphene nanoribbon. Solid State Commun. 152, 2109–2112 (2012)
Al-Dirini, F., Hossain, F.M., Nirmalathas, A., Skafidas, E.: Alll-graphene planar double barrier resonant tunnelling diodes. J. Electron Devices Soc. 2(5), 118–122 (2014)
Kargar, A.: Analytical modeling of graphene nanoribbon Schottky diodes using asymmetric contacts. J. Comput. Theor. Nanosci. 8, 1–6 (2011)
Kang, J., Wu, F., Li, S.-S., Xia, J.-B., Li, J.: Antiferromagnetic coupling and spin filtering in asymmetrically hydrogenated graphene nanoribbon homojunction. Appl. Phys. Lett. 100, 153102 (2012)
Zeng, J., Chen, K.-Q., He, J., Zhang, X.-J., Sun, C.Q.: Edge hydrogenation-induced spin-filtering and rectifying behaviors in the graphene nanoribbon heterojunctions. J. Phys. Chem. C 115, 25072–25076 (2011)
Liu, J., Zhang, Z.H., Deng, X.Q., Fan, Z.Q., Tang, G.P.: Electronic structures and transport properties of armchair graphene nanoribbons by ordered doping. Organ. Electron. 18, 135–142 (2015)
Li, J., Zhang, Z.H., Zhang, J.J., Deng, X.Q.: Rectifying regularity for a combined nanostructure of two trigonal graphenes with different edge modifications. Organ. Electron. 13, 2257–2263 (2012)
Ling, Y.-C., Ning, F., Zhou, Y.-H., Chen, K.-Q.: Rectifying behavior and negative differential resistance in triangular graphene p-n junctions induced by vertex B-N mixture doping. Organ. Electron. 19, 92–97 (2015)
Zhao, P., Liu, D.S., Li, S.J., Chen, G.: Modulation of rectification and negative differential resistance in graphene nanoribbon by nitrogen doping. Phys. Lett. A 377, 1134–1138 (2013)
Peng, J., Zhou, Y.-H., Chen, K.-Q.: Influence of boundary types on rectifying behaviors in hexagonal boron-nitride/graphene nanoribbon heterojunctions. Organ. Electron. 27, 137–142 (2015)
Li, J., Zhang, Z.H., Qui, M., Yuan, C., Deng, X.Q., Fan, Z.Q., Tang, G.P., Liang, B.: High-performance current rectification in a molecular device with doped graphene electrodes. Carbon 80, 575–582 (2014)
Singh, A.K., Auton, G., Hill, E., Song, A.: Graphene based ballistic rectifiers. Carbon 84, 124–129 (2015)
Cao, C., Long, M.-Q., Zhang, X.-J., Mao, X.-C.: Giant magnetoresistance and spin-filtering effects in zigzag graphene and hexagonal boron nitride based heterojunction. Phys. Lett. A 379, 1527–1531 (2015)
Cao, C., Chen, N.-L., Long, M.-Q., Xu, H.: Rectifying performance in zigzag graphene nanoribbon heterojunctions with different edge hydrogenations. Phys. Lett. A 377, 1905–1910 (2013)
Deng, X.Q., Zhang, Z.H., Tang, G.P., Fan, Z.Q., Yang, C.H.: Spin filter effects in zigzag-edge graphene nanoribbons with symmetric and asymmetric edge hydrogenations. Carbon 66, 646–653 (2014)
Kang, J., Wu, F., Li, J.: Doping induced spin filtering effect in zigzag graphene nanoribbons with asymmetric edge hydrogenation. Appl. Phys. Lett. 98, 083109 (2011)
Cao, C., Chen, L., Huang, W., Xu, H.: Electronic transport of zigzag graphene nanoribbons with edge hydrogenation and oxidation. Open Chem. Phys. J. 4, 1–7 (2012)
Deng, X.Q., Zhang, Z.H., Yang, C.H., Zhu, H.L., Liang, B.: The design of spin filter junction in zigzag graphene nanoribbons with asymmetric edge hydrogenation. Org. Electron. 14(12), 3240–3248 (2013)
Son, Y.-L., Zhang, Y., Zhang, J.-M., Lu, D.-B.: Effects of the edge shape and the width on the structural and electronic properties of silicene nanoribbons. Appl. Surf. Sci. 256(21), 6313–6317 (2010)
Trivedi, S., Anurag, S., Rajnish, K.: Electronic and transport properties of silicene nanoribbons. J. Comput. Theor. Nanosci. 11(3), 789–794 (2014)
Kara, A., Enriquez, H., Seitsonen, A.P., Voon, L.C., Vizzini, S., Aufray, B., Aughaddou, H.: A review on silicene-new candidate for electronics. Surf. Sci. Rep. 67(1), 1–8 (2012)
Ni, Z., Liu, Q., Tang, K., Zheng, J., Zhou, J., Qin, R., Gao, Z., Yu, D., Lu, J.: Tunable bandgap in silicene and germanene. Nano Lett. 12, 113–118 (2012)
Zhou, B., Zhou, B., Zeng, Y., Zhou, G., Duan, M.: Tunable electronic and transport properties for ultranarrow armchair-edge silicene nanoribbons under spin-orbit coupling and perpendicular electric field. Phys. Lett. A 380(1–2), 282–287 (2016)
Li, H., Wang, L., Liu, Q., Zheng, J., Mei, W.-N., Gao, Z., Shi, J., Lu, J.: High performance silicene nanoribbon field effect transistors with current saturation. Eur. Phys. J. B 85, 1–6 (2012)
Zhang, D., Long, M., Zhang, X., Cao, C., Xu, H., Li, M., Chan, K.: Bipolar spin-filtering, rectifying and giant magnetoresistance effects in zigzag silicene nanoribbons with asymmetric edge hydrogenation. Chem. Phys. Lett. 616, 178–183 (2014)
Yamacli, S.: First principles study of the voltage-dependent conductance properties of n-type and p-type graphene-metal contacts. Comput. Mater. Sci. 81, 607–611 (2014)
Huang, Y., Zhang, Z., Ma, F., Chu, P.K., Dong, C., Wei, X.: First-principles calculation of the band structure, electronic states, and optical properties of Cr-doped ZnS double-wall nanotubes. Comput. Mater. Sci. 101, 1–7 (2015)
Srivastava, A., Tyagi, N., Ahuja, R.: First-principles study of structural and electronic properties of gallium based nanowires. Solid State Sci. 23, 35–41 (2013)
Min, Y., Fang, H.J., Zhong, C.G., Dong, Z.C., Chen, C.P., Yao, K.L.: Disconnect armchair carbon nanotube as rectifier predicted by first-principles study. Comput. Mater. Sci. 81, 418–422 (2014)
Min, Y., Fang, H.J., Zhong, C.G., Dong, Z.C., Zhao, Z.Y., Zhou, P.X., Yao, K.L.: Bias changing molecule-lead couple and inducing low bias negative differential resistance for electrons acceptor predicted by first-principles study. Phys. Lett. A 379(40–41), 2637–2640 (2015)
Atomistix Toolkit version 2014.1, Quantumwise A/S, Cophenagen, Denmark
Brandbyge, M., Mozos, J.-L., Ordejon, P., Taylor, J., Stokbro, K.: Density-functional method for nonequilibrium electron transport. Phys. Rev. B 65, 165401 (2002)
Soler, J.M., Artacho, E., Gale, J.D., Garcia, A., Junquera, J., Ordejon, P., Sanchez-Portal, D.: The Siesta method for ab initio order-N materials simulation. J. Phys. 14, 2745 (2002)
Abadir, G.B., Walus, K., Pulfrey, D.L.: Basis set choice for DFT/NEGF simulations of carbon nanotubes. J. Comput. Electron. 8, 1–9 (2009)
Zhang, X.-L., Liu, L.-F., Liu, W.-M.: Quantum anomalous Hall effect and tunable topological states in 3d transition metals doped silicene. Sci. Rep. 33, 2908 (2013)
Jia, T.T., Zheng, M.-M., Fan, X.-Y., Su, Y., Li, S.-J., Liu, H.-Y., Chen, G., Kawazoe, Y.: Band gap on/off switching of silicene superlattice. J. Phys. Chem. C 119(35), 20747–20754 (2015)
Datta, S.: Quantum Transport: Atom to Transistor. Cambridge University Press, Cambridge (2013)
Yamacli, S.: Algebraic current-voltage and voltage dependent resistance expressions for ballistic nano conductors and their low voltage nonlinearity. Nano Micro Lett. 5(3), 169–173 (2013)
The International Technology Roadmap for Semiconductors (ITRS), www.itrs.net (2015)
Sakuma, K., Koizumi, H.: Influence of junction capacitance of switching devices on Class E rectifier. In: IEEE International Symposium on Circuits and Systems, pp. 1965–1968 (2009)
Author information
Authors and Affiliations
Corresponding author
Rights and permissions
About this article
Cite this article
Yamacli, S. Investigation and comparison of bare-dihydrogenated junction rectifiers of graphene and silicene nanoribbons. J Comput Electron 15, 389–399 (2016). https://doi.org/10.1007/s10825-016-0805-6
Published:
Issue Date:
DOI: https://doi.org/10.1007/s10825-016-0805-6