Abstract
Expanding on current efforts to evaluate the role of groundwater dynamics in managing and restoring Chesapeake Bay (USA), the integrated hydrologic model ParFlow-CLM was applied to a 374,976-km2 area encompassing the Chesapeake Bay watershed. The model included a representation of surface water, groundwater and land-surface energy fluxes with spatially variable atmospheric forcing at an hourly time step. The study tackled issues of data availability, access, assembly, and synthesis for estimating hydrogeologic properties in the context of the development of a large-scale model. Hydrogeologic properties from literature and other sources were assembled, processed, and synthesized to derive a conceptual hydrogeologic model consisting of 29 hydrofacies and a three-dimensional hydraulic conductivity field. Evaluation of the ParFlow-CLM model output showed that the constructed model captured seasonal and spatial variability in subsurface storage, surface storage and surface runoff, and produced water-table depths consistent with the topography, meteorological forcing, and hydrogeology. Comparison with well data from the US Geological Survey showed good agreement of model output with observed hydraulic heads for most of the data. Modeled terrestrial water storage changes compared well with GRACE satellite data with a root mean square error of 2.3 cm. Model results showed the dominant contribution of subsurface storage changes (90%) to terrestrial water storage changes in the region.
Résumé
Dans le cadre des efforts actuels visant à évaluer le rôle de la dynamique des eaux souterraines dans la gestion et la restauration de la baie de Chesapeake (États-Unis d’Amérique), le modèle hydrologique intégré ParFlow-CLM a été appliqué à une zone de 374 976 km2 englobant le bassin versant de la baie de Chesapeake. Le modèle comprend la représentation des eaux de surface, des eaux souterraines, et des flux d'énergie à la surface du sol, avec un forçage atmosphérique variable dans l’espace, et à un pas de temps horaire. L’étude a abordé les questions de disponibilité, d’accès, d’assemblage et de synthèse des données pour l’estimation des propriétés hydrogéologiques dans le contexte du développement d’un modèle à grande échelle. Les propriétés hydrogéologiques provenant de la littérature et d’autres sources ont été assemblées, traitées et synthétisées pour en déduire un modèle hydrogéologique conceptuel composé de 29 ompartiments hydrogeologiques et d’un champ de conductivité hydraulique tridimensionnel. L’évaluation des résultats du modèle ParFlow-CLM montre que le modèle construit reproduit la variabilité saisonnière et spatiale des stocks d'eaux souterraines, de surface, et des eaux de ruissellement, et fournit des profondeurs de nappe phréatique cohérentes avec la topographie, le forçage météorologique et l’hydrogéologie. La comparaison avec les données du Service Géologique des Etats Unis d’Amérique a montré une bonne adéquation des résultats du modèle compares aux charges hydrauliques observées, pour la plupart des données. Les variations des stocks d'eaux terrestres modélisées sont cohérentes avec les données du satellite GRACE, avec une erreur quadratique moyenne de 2.3 cm. Les résultats du modèle ont montré la contribution dominante des variations de stocks d'eaux souterraines (90 %) par rapport aux variations des stocks d'eaux terrestres dans la région.
Resumen
A partir de los esfuerzos actuales para evaluar el papel de la dinámica de las aguas subterráneas en la gestión y restauración de la bahía de Chesapeake (EE.UU.), se aplicó el modelo hidrológico integrado ParFlow-CLM a un área de 374,976 km2 que abarca la cuenca de la bahía de Chesapeake. El modelo incluía la representación de las aguas superficiales, las aguas subterráneas y los flujos de energía de la superficie terrestre con un condicionamiento atmosférico espacialmente variable en un paso de tiempo de una hora. El estudio abordó cuestiones relativas a la disponibilidad, el acceso, el montaje y la síntesis de datos para estimar las propiedades hidrogeológicas en el contexto del desarrollo de un modelo a gran escala. Se reunieron, procesaron y sintetizaron las propiedades hidrogeológicas procedentes de la literatura y de otras fuentes para derivar un modelo hidrogeológico conceptual compuesto por 29 hidrofacies y un campo de conductividad hidráulica tridimensional. La evaluación de la salida del modelo ParFlow-CLM mostró que el modelo construido capturó la variabilidad estacional y espacial en el almacenamiento subterráneo, el almacenamiento superficial y la escorrentía superficial, y produjo profundidades del nivel freático consistentes con la topografía, el forzamiento meteorológico y la hidrogeología. La comparación con los datos de los pozos del Servicio Geológico de los Estados Unidos mostró una buena concordancia entre los resultados del modelo y las alturas hidráulicas observadas para la mayoría de los datos. Los cambios de almacenamiento de agua terrestre modelados se compararon adecuadamente con los datos del satélite GRACE, con un error cuadrático medio de 2.3 cm. Los resultados del modelo mostraron la contribución dominante de los cambios de almacenamiento subterráneo (90%) a los cambios de almacenamiento de agua terrestre en la región.
摘要
基于目前评估地下水动态在管理和恢复美国切萨皮克湾中的作用而扩展开发的综合水文模型ParFlow-CLM,进一步应用于包括切萨皮克湾流域在内的374,976 km2 区域。该模型包括了以每小时为时间步长的空间可变的大气作用力下的地表水、地下水和地表能量通量的表征。在发展大尺度模型的背景下,该研究解决了用于评估水文地质特性的数据可用性、数据获取、数据汇编和数据生成等问题。通过对来自文献和其他来源的水文地质属性进行汇总、处理和综合,得出了一个由29种水相和1个三维渗透系数场组成的水文地质概念模型。对ParFlow-CLM模型输出的评价显示,构建的模型捕捉到了地下水储量、地表水储量和地表径流量的季节性和空间变化,生成的地下水位埋深与地形、气象作用和水文地质相一致。通过与美国地质调查局的水井数据进行比较,对于大多数数据而言,模型输出与观测的水力压头显示出了良好的一致性。模拟的陆地水体储量变化与GRACE卫星数据相比基本一致,均方根误差为2.3 cm。模型结果显示,该地区陆地水体储量变化主要由地下水储量变化引起(贡献率占90%)。
Resumo
Ampliando os esforços atuais para avaliar o papel da dinâmica das águas subterrâneas na gestão e restauração da Baía de Chesapeake (EUA), o modelo hidrológico integrado ParFlow-CLM foi aplicado a uma área de 374,976 km2 abrangendo a bacia hidrográfica da Baía de Chesapeake. O modelo incluiu a representação dos fluxos de energia das águas superficiais, subterrâneas e terrestres com forçamento atmosférico espacialmente variável em um intervalo de tempo de hora em hora. O estudo abordou questões de disponibilidade, acesso, montagem e síntese de dados para estimar propriedades hidrogeológicas no contexto do desenvolvimento de um modelo em grande escala. Propriedades hidrogeológicas da literatura e de outras fontes foram reunidas, processadas e sintetizadas para derivar um modelo hidrogeológico conceitual composto por 29 hidrofácies e um campo de condutividade hidráulica tridimensional. A avaliação da saída do modelo ParFlow-CLM mostrou que o modelo construído capturou a variabilidade sazonal e espacial no armazenamento subsuperficial, armazenamento superficial e escoamento superficial, e produziu profundidades do lençol freático consistentes com a topografia, forçamento meteorológico e hidrogeologia. A comparação com dados de poços do Serviço Geológico dos Estados Unidos mostrou boa concordância da saída do modelo com as cargas hidráulicas observadas para a maioria dos dados. As mudanças modeladas no armazenamento de água terrestre compararam-se bem com os dados do satélite GRACE com um erro quadrático médio de 2.3 cm. Os resultados do modelo mostraram a contribuição dominante das mudanças no armazenamento subterrâneo (90%) para as mudanças no armazenamento de água terrestre na região.












Similar content being viewed by others
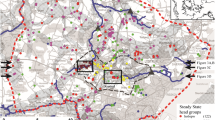
References
Ames DP, Horsburgh JS, Cao Y, Kadlec J, Whiteaker T, Valentine D (2012) HydroDesktop: Web services-based software for hydrologic data discovery, download, visualization, and analysis. Environ Model Softw 37(0):146–156
Ashby SF, Falgout RD (1996) A parallel multigrid preconditioned conjugate gradient algorithm for groundwater flow simulations. Nucl Sci Eng 124(1):145–149
Ator SW, Blomquist JD, Webber JS, Chanat JG (2020) Factors driving nutrient trends in streams of the Chesapeake Bay watershed. J Environ Qual 49(4):812–834
Bachman LJ (1998) Ground-water discharge and base-flow nitrate loads of nontidal streams, and their relation to a hydrogeomorphic classification of the Chesapeake Bay watershed, middle Atlantic coast. US Geol Surv Water Resour Invest Rep 98(4039):71
Back W, Rosenshein JS, Seaber PR (1988) Hydrogeology. Geological Society of America. https://doi.org/10.1130/DNAG-GNA-O2
Band L, Dillaha T, Duffy C, Reckhow K, Welty C (2008) Second review of the Phase V Community Chesapeake Bay Watershed Model. STAC Publ. 08-003, Scientific and Technical Advisory Committee, Edgewater, MD
Barnes M, Welty C, Miller AJ (2015) Global topographic slope enforcement to ensure connectivity and drainage in an urban terrain. J Hydrol Eng. https://doi.org/10.1061/14(ASCE)HE.1943-5584.0001306
Beven K (2006) Searching for the Holy Grail of scientific hydrology: Qt=(S, R, Δt)A as closure. Hydrol Earth Syst Sci Discuss 10(5):609–618
Bierkens MF, Bell VA, Burek P, Chaney N, Condon LE, David CH, de Roo A, Döll P, Drost N, Famiglietti JS, Flörke M (2015) Hyper-resolution global hydrological modelling: what is next? Hydrol Process 29(2):310–320
Chapelle FH (1985) Hydrogeology,digital solute transport simulation, and geochemistry of the Lower Cretaceous Aquifer System near Baltimore, Maryland. US Geol Surv Rep Invest 43:120
Chen X, Hu Q (2004) Groundwater influences on soil moisture and surface evaporation. J Hydrol 297(1–4):285–300
Cleaves ET (1968) Piedmont and Coastal Plain geology along the Susquehanna Aqueduct, Baltimore to Aberdeen, Maryland. MD Geol Survey Rep Invest 8
Cloke HL, Hannah DM (2011) Large-scale hydrology: advances in understanding processes, dynamics and models from beyond river basin to global scale. Hydrol Process 25(7):991–995
Condon LE, Maxwell RM, Gangopadhyay S (2013) The impact of subsurface conceptualization on land energy fluxes. Adv Water Resour 60:188–203
Cosgrove BA, Lohmann D, Mitchell KE, Houser PR, Wood EF, Schaake JC, Robock A, Sheffield J, Duan Q, Luo L, Higgins RW, Pinker RT, Tarpley JD (2003) Land surface model spin‐up behavior in the North American Land Data Assimilation System (NLDAS). J Geophys Res Atmos 108(D22): 2002JD003316. https://doi.org/10.1029/2002JD003316
Creutzfeldt B, Troch PA, Güntner A, Ferré T, Graeff T, Merz B (2014) Storage-discharge relationships at different catchment scales based on local high-precision gravimetry. Hydrol Process 28(3):1465–1475
Cunningham WL, Daniel CCI (2001) Investigation of ground-water availability and quality in Orange County, North Carolina. US Geol Surv Water Resour Invest Rep 00–4286, 65 pp
Dai Y et al (2003) The common land model. Bull Am Meteorol Soc 84(8):1013–1023
Daniel III CC, Harned D (1998) Ground-water recharge to and storage in the regolith-fractured crystalline rock aquifer system, Guilford County, North Carolina, vol 97, no. 4140. US Geological Survey, Reston, MD
Dankers R, Feyen L (2008) Climate change impact on flood hazard in Europe: an assessment based on high-resolution climate simulations. J Geophys Res 113:D19105. https://doi.org/10.1029/2007JD009719
De Buchananne GD, Richardson RM (1956) Groundwater resources of east Tennessee. Tennessee Division Geol Bull 58, part I, 393 pp
de Graaf IEM, Sutanudjaja EH, van Beek LPH, Bierkens MFP (2014) A high-resolution global scale groundwater model. Hydrol Earth Syst Sci Discuss 11(5):5217–5250
de Graaf I, Condon L, Maxwell R (2020) Hyper-resolution continental-scale 3-D aquifer parameterization for groundwater modeling. Water Resour Res 56(5):e2019WR026004
Duffy CJ (1996) A two-state integral-balance model for soil moisture and groundwater dynamics in complex terrain. Water Resour Res 32(8):2421–2434
Döll P, Fiedler K (2008) Global-scale modeling of groundwater recharge. Hydrol Earth Syst Sci 12(3):863–885
Döll P, Berkhoff K, Bormann H, Fohrer N, Gerten D, Hagemann S, Krol M (2008) Advances and visions in large-scale hydrological modelling: findings from the 11th Workshop on Large-Scale Hydrological Modelling. Adv Geosci 18:51–61
Fan Y, Miguez-Macho G, Weaver CP, Walko R, Robock A (2007) Incorporating water table dynamics in climate modeling: 1. water table observations and equilibrium water table simulations. J Geophys Res Atmos 112(D10)
Fan Y, Li H, Miguez-Macho G (2013) Global patterns of groundwater table depth. Science 339(6122):940–943
Fan Y et al (2014) DigitalCrust: a 4D data system of material properties for transforming research on crustal fluid flow. Geofluids 15:372–379
Fenneman NM (1938) Physiography of eastern United States. McGraw-Hill, New York
Focazio MJ, Plummer LN, Bohlke JK, Busenberg E (1998) Preliminary estimates of residence times and apparent ages of ground water in the Chesapeake Bay Watershed, and water-quality data from a survey of springs. US Geol Surv Water Resour Invest Rep 97(4225):75
Freeze RA, Cherry JA (1979) Groundwater. Prentice-Hall, Upper Saddle River, NJ
Getirana ACV, Bonnet MP, Rotunno Filho OC, Collischonn W, Guyot JL, Seyler F, Mansur WJ (2010) Hydrological modelling and water balance of the Negro River basin: evaluation based on in situ and spatial altimetry data. Hydrol Process 24(22):3219–3236
Gleeson T, Smith L, Moosdorf N, Hartmann J, Dürr HH, Manning AH, van Beek LPH, Jellinek AM (2011) Mapping permeability over the surface of the Earth. Geophys Res Lett 38(2):L02401
Gleeson T, Moosdorf N, Hartmann J, van Beek LPH (2014) A glimpse beneath earth’s surface: GLobal HYdrogeology MaPS (GLHYMPS) of permeability and porosity. Geophys Res Lett 41(11):2014GL059856
Gleeson T, Wagener T, Döll P, Zipper SC, West C, Wada Y, Taylor R, Scanlon B, Rosolem R, Rahman S, Oshinlaja N (2021) GMD Perspective: the quest to improve the evaluation of groundwater representation in continental to global scale models. Geoscientific Model Development Discussions, European Geosciences Union, Munich, Germany, pp 1–59
Greene EA, Shapiro AM, LaMotte AE (2004) Hydrogeologic controls on ground-water discharge to the Washington METRO subway tunnel near the Medical Center Station and Crossover, Montgomery County, Maryland. US Geol Surv Water Resour Invest Rep 2003-4294, 33 pp
Hagemann S, Göttel H, Jacob D, Lorenz P, Roeckner E (2008) Improved regional scale processes reflected in projected hydrological changes over large European catchments. Clim Dyn 32(6):767–781
Harlow Jr GE, LeCain GD (1993) Hydraulic characteristics of, and ground-water flow in, coal-bearing rocks of southwestern Virginia (no. 2388). US Government Printing Office, Washington, DC
Harned D (1989) The hydrogeological framework and a reconnaissance of ground-water quality in the Piedmont Province of North Carolina, with a design for future study, vol 88, no. 4130. US Geological Survey, Reston, VA
Heath, R. C (1984) Basic groundwater hydrogeology. US Geol Surv Water Suppl Pap 2220
Heidorn B (2008) Shedding light on the dark data in the long tail of science. Libr Trends 57(2):280–299
Herpers H, Barksdale HC (1951) Preliminary report on the geology and ground-water supply of the Newark, New Jersey, area (no. 10). New Jersey Department of Conservation and Economic Development, Division of Water Policy and Supply, Trenton, NJ
Hill MC (1990) Solving groundwater flow problems by conjugate-gradient methods and the strongly implicit procedure. Water Resour Res 26(9):1961–1969
Hobba WA (1979) Hydrology and geochemistry of thermal springs of the Appalachians, vol 1044). US Government Printing Office, Washington, DC
Hollyday EF, Hileman GE (1997) Hydrogeologic terranes and potential yield of water to wells in the Valley and Ridge Physiographic Province in the eastern and southeastern United States (No. 1422). US Government Printing Office, Washington, DC
Homer CH, Fry JA, Barnes CA (2012) The national land cover database. US Geol Surv Fact Sheet 3020(4):1–4
Hutchinson MF (2008) Adding the Z dimension. In: Wilson JP, Fotheringham AS (eds) The handbook of geographic information science. Blackwell, Oxford, UK, pp 144–168
Hutchinson MF, Gallant JC (2000) Digital elevation models and representation of terrain shape. In: Terrain analysis: principles and applications. Wiley, Chichester, UK, pp 29–50
Jones JE, Woodward CS (2001) Newton–Krylov-multigrid solvers for large-scale, highly heterogeneous, variably saturated flow problems. Adv Water Resour 24(7):763–774
King PB, Beikman HM (1974) Explanatory text to accompany the geologic map of the United States (no. 854). US Government Printing Office, Washington, DC
Kirchner JW (2009) Catchments as simple dynamical systems: catchment characterization, rainfall-runoff modeling, and doing hydrology backward. Water Resour Res 45(2)
Kollet SJ, Maxwell RM (2006) Integrated surface–groundwater flow modeling: a free-surface overland flow boundary condition in a parallel groundwater flow model. Adv Water Resour 29(7):945–958
Kollet SJ, Cvijanovic I, Schüttemeyer D, Maxwell RM, Moene AF, Bayer P (2009) The influence of rain sensible heat and subsurface energy transport on the energy balance at the land surface. Vadose Zone J 8(4):846–857
Kollet SJ, Maxwell RM, Woodward CS, Smith S, Vanderborght J, Vereecken H, Simmer C (2010) Proof of concept of regional scale hydrologic simulations at hydrologic resolution utilizing massively parallel computer resources. Water Resour Res 46(4):W04201
Leahy PP, Martin M (1993) Geohydrology and simulation of ground-water flow in the Northern Atlantic Coastal Plain Aquifer System, US Geol Surv Prof Pap 1404-K, 81 pp
Lehner B, Verdin K, Jarvis A (2008) New global hydrography derived from spaceborne elevation data. Eos Trans Am Geophys Union 89(10):93–94
LeGrand HE (1961) Summary of geology of Atlantic coastal plain. AAPG Bull 45(9):1557–1571
Liang X, Xie Z, Huang M (2003) A new parameterization for surface and groundwater interactions and its impact on water budgets with the variable infiltration capacity (VIC) land surface model. J Geophys Res Atmos 108(D16)
Lindsey BD, Phillips SW, Donnelly CA, Speiran GK, Plummer LN, Böhlke J-K, Focazio MJ, Burton WC, Busenberg E (2003) Residence times and nitrate transport in ground water discharging to streams in the Chesapeake Bay Watershed. US Geological Survey, Washington, DC
Low DJ, Hippe DJ, Yannaci D (2002) Geohydrology of southeastern Pennsylvania. US Geol Surv SciWater Resour Invest Rep 00-4166, 347 pp
Maxwell RM, Miller NL (2005) Development of a coupled land surface and groundwater model. J Hydrometeorol 6(3):233–247
Maxwell R, Kollet S (2008a) Interdependence of groundwater dynamics and land-energy feedbacks under climate change. Nat Geosci 1(10):665–669
Maxwell RM, Kollet SJ (2008b) Quantifying the effects of three-dimensional subsurface heterogeneity on Hortonian runoff processes using a coupled numerical, stochastic approach. Adv Water Resour 31(5):807–817
Maxwell RM, Kollet SJ, Smith SG, Woodward CS, Falgout RD, Ferguson IM, Baldwin C, Bosl WJ, Hornung R, Ashby S (2009) ParFlow user’s manual. International Ground Water Modeling Center Report GWMI 1(2009):129
Maxwell RM, Condon LE, Kollet SJ (2015) A high-resolution simulation of groundwater and surface water over most of the continental US with the integrated hydrologic model ParFlow v3. Geosci Model Dev 8(3):923–937
McFarland ER (1997) Groundwater flow, geochemistry, and effects of agricultural practices on nitorgen transport at study sites in the Piedmont and Coastal Plain Physiographic provinces, Patuxent River Basin, MD. US Geol Surv Water Suppl Pap 2449, 72 pp
McGreevy LJ, Sloto RA (1976) Selected hydrologic data, Chester County, Pennsylvania. US Geol Surv Open-file Rep 87-217, 138 pp
McNamara JP, Tetzlaff D, Bishop K, Soulsby C, Seyfried M, Peters NE, Aulenbach BT, Hooper R (2011) Storage as a metric of catchment comparison. Hydrol Process 25(21):3364–3371
Miguez-Macho G, Fan Y (2012) The role of groundwater in the Amazon water cycle: 1. influence on seasonal streamflow, flooding and wetlands. J Geophys Res Atmos 117(D15):n/a–n/a
Miguez-Macho G, Li H, Fan Y (2008) Simulated water table and soil moisture climatology over North America. Bull Am Meteorol Soc 89(5):663–672
Mitchell KE, Lohmann D, Houser PR, Wood EF, Schaake JC, Robock A, Cosgrove BA, Sheffield J, Duan Q, Luo L, Higgins RW (2004) The multi-institution North American Land Data Assimilation System (NLDAS): utilizing multiple GCIP products and partners in a continental distributed hydrological modeling system. J Geophys Res Atmos 109(D7):D07S90
NASA Land Processes Distributed Active Archive Center (2001) ASTER L1B. USGS/ Earth Resources Observation and Science (EROS) Center, Sioux Falls, SD
Natural Resources Conservation Service (2014) United States Department of Agriculture U.S. General Soil Map (STATSGO2). http://sdmdataaccess.nrcs.usda.gov/. Accessed June 2012
Niu J, Shen C, Li SG, Phanikumar MS (2014) Quantifying storage changes in regional Great Lakes watersheds using a coupled subsurface-land surface process model and GRACE, MODIS products. Water Resour Res 50(9):7359–7377
Nutter LJ, Otton EG (1969) Ground-water occurrence in the Maryland Piedmont. Maryland Geological Survey Report of Investigations no. 10, Maryland Geological Survey, Baltimore, MD, 56 pp
Phillips SW, Focazio MJ, Bachman LJ (1999) Discharge, nitrate load, and residence time of ground water in the Chesapeake Bay watershed. US Geol Surv Fact Sheet FS-150-99, 6 pp
Pokhrel YN, Fan Y, Miguez-Macho G, Yeh PJF, Han SC (2013) The role of groundwater in the Amazon water cycle: 3. influence on terrestrial water storage computations and comparison with GRACE. J Geophys Res Atmos 118(8):3233–3244
Preston SD, Brakebill JW (1999) Application of spatially referenced regression modeling for the evaluation of total nitrogen loading in the Chesapeake Bay Watershed. US Geol Surv Water Invest Rep 99-4054, 12 pp
Reilly TE (2008) Ground-water availability in the United States. US Geological Survey, Reston, VA
Richardson CA (1982) Ground water in the Piedmont Upland of central Maryland. US Geol Surv Water Supply Paper 2077, 42 pp
Rice KC, Jastram JD (2015) Rising air and stream-water temperatures in Chesapeake Bay region, USA. Climate Change 128:127–138
Rihani JF, Maxwell RM, Chow FK (2010) Coupling groundwater and land surface processes: idealized simulations to identify effects of terrain and subsurface heterogeneity on land surface energy fluxes. Water Resour Res 46(12)
Ritter WF (2019) Progress on the Chesapeake Bay TMDL and challenges in meeting the 2025 pollution-reduction loads. In: World Environmental and Water Resources Congress 2019: Watershed Management, Irrigation and Drainage, and Water Resources Planning and Management. American Society of Civil Engineers, Washington, DC, pp 63–70
Rodell M, Chen J, Kato H, Famiglietti JS, Nigro J, Wilson CR (2007) Estimating groundwater storage changes in the Mississippi River basin (USA) using GRACE. Hydrogeol J 15(1):159–166
Rodriguez-Iturbe I (2000) Ecohydrology: a hydrologic perspective of climate-soil-vegetation dynamics. Water Resour Res 36(1):3–9
Rossman NR, Zlotnik VA (2013) Regional groundwater flow modeling in heavily irrigated basins of selected states in the western United States. Hydrogeol J 21(6):1173–1192
Saar MO, Manga M (2004) Depth dependence of permeability in the Oregon Cascades inferred from hydrogeologic, thermal, seismic, and magmatic modeling constraints. J Geophys Res: Solid Earth 109(B4). https://doi.org/10.1029/2003JB002855
Salvucci GD, Entekhabi D (1995) Hillslope and climatic controls on hydrologic fluxes. Water Resour Res 31(7):1725–1739
Sanford WE (2017) Estimating regional-scale permeability-depth relation in a fractured-rock terrain using groundwater-flow model calibration. Hydrogeol J 25:405–419
Sanford WE, Pope JP (2013) Quantifying groundwater’s role in delaying improvements to Chesapeake Bay water quality. Environ Sci Technol 47(23):13330–13338
Sanford WE, Pope JP, Selnick DL, Stumvoll RF (2012) Simulation of groundwater flow in the shallow aquifer system of the Delmarva Peninsula, Maryland and Delaware. US Geol Surv Open-File Rep 2012-1140, 58 pp
Sayama T, McDonnell JJ, Dhakal A, Sullivan K (2011) How much water can a watershed store? Hydrol Process 25(25):3899–3908
Seck A, Welty C, Maxwell RM (2015) Spin-up behavior and effects of initial conditions for an integrated hydrologic model. Water Resour Res 51(4):2188–2210
Schwartz R (2007) Hurricanes and the middle Atlantic states. Blue Diamond, Alexandria, VA
Seilheimer TS, Zimmerman PL, Stueve KM, Perry CH (2013) Landscape-scale modeling of water quality in Lake Superior and Lake Michigan watersheds: how useful are forest-based indicators? J Great Lakes Res 39(2):211–223
Soulsby C, Tetzlaff D, Hrachowitz M (2009) Tracers and transit times: windows for viewing catchment scale storage? Hydrol Process 23(24):3503–3507
Soulsby C, Piegat K, Seibert J, Tetzlaff D (2011) Catchment-scale estimates of flow path partitioning and water storage based on transit time and runoff modelling. Hydrol Process 25(25):3960–3976
Spence C (2007) On the relation between dynamic storage and runoff: a discussion on thresholds, efficiency, and function. Water Resour Res 43(12)
Stewart JW (1962) Water-yielding potential of weathered crystalline rocks at the Georgia Nuclear Laboratory. US Geol Surv Prof Pap 450:106–107
Sutanudjaja EH, van Beek LPH, de Jong SM, van Geer FC, Bierkens MFP (2011) Large-scale groundwater modeling using global datasets: a test case for the Rhine-Meuse basin. Hydrol Earth Syst Sci 15(9):2913–2935
Swain LA, Hollyday EF, Daniel CCI, Zapecza OS (1991) Plan of Study for the regional aquifer-system analysis of the Appalachian Valley and Ridge, Piedmont, and Blue Ridge Physiographic provinces of the eastern and southeastern United States, with a description of study-area geology and hydrogeology. US Geol Surv Water Resour Invest Rep 91(4066):44
Swain LA, Mesko TO, Hollyday EF (2004) Summary of the Hydrogeology of the Valley and Ridge, Blue Ridge, and Piedmont Physiographic Provinces in the Eastern United States. US Geol Surv Prof Pap 1422(A):31
Tapley BD, Bettadpur S, Ries JC, Thompson PF, Watkins MM (2004) GRACE measurements of mass variability in the Earth system. Science 305(5683):503–505
Tarboton DG, Maidment DR, Zaslavsky I, Ames DP, Goodall J, Hooper RP, Horsburgh JS, Valentine D, Whiteaker T, Schreuders K (2011) Data interoperability in the hydrologic sciences. Environmental Information Management Conference, Santa Barbara, CA, September 2011
Tijerina D, Condon L, FitzGerald K, Dugger A, O’Neill MM, Sampson K, Gochis D, Maxwell R (2021) Continental Hydrologic Intercomparison Project, phase 1: a large-scale hydrologic model comparison over the continental United States. Water Resour Res 57(7):e2020WR028931
Trapp HJ (1992) Hydrogeologic framework of the Northern Atlantic Coastal Plain in parts of North Carolina, Virginia, Maryland, Delaware, New Jersey, and New York. US Geol Surv Prof Pap 1404-G, 59 pp
University of Texas, Center for Space Research (2014) Gravity Recovery and Climate Experiment GRACE CSR Release-05 Level-2 data products. http://www2.csr.utexas.edu/grace/RL05.html. Accessed December 2013
USEPA (US Environmental Protection Agency) (2002) The state of the Chesapeake Bay. US Environmental Protection Agency report 903-R-02-002, USEPA, Washington, DC
US Geological Survey (2014) National elevation data. http://nationalmap.gov/viewer.html . Accessed Jan 2012
van Genuchten MT (1980) A closed-form equation for predicting the hydraulic conductivity of unsaturated soils. Soil Sci Soc Am J 44(5):892–898
Vecchioli J, Gill HE, Lang SM (1962) Hydrologic role of the Great Swamp and other marshland in Upper Passaic River Basin. J Am Water Works Assoc 54(6):695–701
Vergnes JP, Roux N, Habets F, Ackerer P, Amraoui N, Besson F, Caballero Y, Courtois Q, De Dreuzy JR, Etchevers P, Gallois N (2020) The AquiFR hydrometeorological modelling platform as a tool for improving groundwater resource monitoring over France: evaluation over a 60-year period. Hydrol Earth Syst Sci 24(2):633–654
Vroblesky DA, Fleck WB (1991) Hydrogeologic framework of the coastal plain in Maryland, Delaware, and the District of Columbia. US Geol Surv Prof Pap 1404-E, 45 pp
Wagener T, Sivapalan M, Troch P, Woods R (2007) Catchment classification and hydrologic similarity. Geogr Compass 1(4):901–931
Wang H, Guan H, Gutiérrez-Jurado HA, Simmons CT (2014) Examination of water budget using satellite products over Australia. J Hydrol 511:546–554
Wood EF et al (2011) Hyperresolution global land surface modeling: meeting a grand challenge for monitoring Earth’s terrestrial water. Water Resour Res 47(5):W05301
Yang D, Musiake K (2003) A continental scale hydrological model using the distributed approach and its application to Asia. Hydrol Process 17(14):2855–2869
Yeh PJF, Eltahir EAB (2005) Representation of water table dynamics in a land surface scheme, part I: model development. J Clim 18(12):1861–1880
Acknowledgements
The contributions of Kelsey Weaver, Thomas Myers, Roxanne Sanderson, Cynthia Ward, Joshua Cole and Tracy Kerchkof in collecting and processing input data sets are acknowledged. Meteorological and hydrogeological data used in this analysis are publicly available, with sources as cited in the text. Land cover classes were obtained from the Oak Ridge National Laboratory Distributed Active Archive Center (ORNL DAAC) for biogeochemical dynamics. Elevation data were from the USGS National Elevation Data (US Geological Survey 2014)
Funding
This work was supported under NASA grant number NNA06CN09A and NOAA grant No. NA10OAR431220. Computing resources were provided in part by (1) the Climate Simulation Laboratory at NCAR’s Computational and Information Systems Laboratory, sponsored by the National Science Foundation and other agencies, (2) the UMBC High Performance Computing Facility (HPCF), supported by the US National Science Foundation through the MRI program (grant no. CNS-0821258) and the SCREMS program (grant No. DMS-0821311), with additional substantial support from the University of Maryland, Baltimore County (UMBC).
Author information
Authors and Affiliations
Corresponding author
Ethics declarations
Conflict of interest
On behalf of all authors, the corresponding author states that there is no conflict of interest.
Additional information
Publisher’s note
Springer Nature remains neutral with regard to jurisdictional claims in published maps and institutional affiliations.
Rights and permissions
Springer Nature or its licensor (e.g. a society or other partner) holds exclusive rights to this article under a publishing agreement with the author(s) or other rightsholder(s); author self-archiving of the accepted manuscript version of this article is solely governed by the terms of such publishing agreement and applicable law.
About this article
Cite this article
Seck, A., Welty, C. Quantifying groundwater storage dynamics in the Chesapeake Bay watershed (USA) using a large-scale integrated hydrologic model with detailed three-dimensional subsurface representation. Hydrogeol J 31, 127–146 (2023). https://doi.org/10.1007/s10040-022-02563-9
Received:
Accepted:
Published:
Issue Date:
DOI: https://doi.org/10.1007/s10040-022-02563-9