Abstract
In the present study, the adsorption of two types of hazardous atoms including arsenic and lead with TON zeolite and bilayer silica (2D-SiO2) have been investigated by employing Ab initio-based density functional theory (DFT) calculations. To reach a full structural optimization and the most stable configuration, four sites were considered for TON zeolite as well as five sites for 2D-SiO2, and adsorption energy along with equilibrium geometry was determined. The adsorption energies of arsenic atom on the surface of 2D-SiO2 absorbents and TON zeolite have obtained equal to and − 1.25 eV and − 2.76 eV, respectively, which both of them are chemisorption type. We also found that the adsorption of lead on the surface of 2D-SiO2 was physisorption type with the adsorption energy accounting for − 0.13 eV, while the adsorption energy between lead and TON was calculated equal to − 2.32 eV which was chemisorption type. Furthermore, our results demonstrate that the TON zeolite was more capable of adsorbing hazardous atoms compared with 2D-SiO2 due to having greater adsorption energy. The adsorption of arsenic on the 2D-SiO2 and TON adsorbents is also stronger than those of lead atom. Furthermore, we modeled and considered graphene, as a common adsorbent nanostructure, to compare and validate the accuracy of our simulations and obtained results. Finally, the electronic density of states (DOS) calculations and charge analysis were done by the use of Mulliken method, and the results confirmed those results that had already been obtained from adsorption energies.

Graphical abstract











Similar content being viewed by others
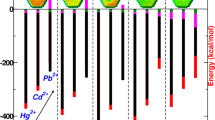
References
Rostamiyan Y, Hamed Mashhadzadeh A, SalmanKhani A (2014) Optimization of mechanical properties of epoxy-based hybrid nanocomposite: Effect of using nano silica and high-impact polystyrene by mixture design approach. Mater Des 56(1980–2015):1068–1077
Rostamiyan Y et al (2015) Experimental and optimizing flexural strength of epoxy-based nanocomposite: effect of using nano silica and nano clay by using response surface design methodology. Mater Des 69:96–104
Rostamiyan Y et al (2015) Using response surface methodology for modeling and optimizing tensile and impact strength properties of fiber orientated quaternary hybrid nano composite. Compos Part B 69:304–316
Rostamiyan Y et al (2015) Experimental study and optimization of damping properties of epoxy-based nanocomposite: effect of using nanosilica and high-impact polystyrene by mixture design approach. Mater Des 65(1980–2015):1236–1244
Hamed Mashhadzadeh A, Fereidoon A, Ahangari MG (2017) Combining density functional theory-finite element multi-scale method to predict mechanical properties of polypropylene/graphene nanocomposites: experimental study. Mater Chem Phys 201:214–223
Hamed Mashhadzadeh A, Fereidoon A, Ahangari MG (2017) Surface modification of carbon nanotubes using 3-aminopropyltriethoxysilane to improve mechanical properties of nanocomposite based polymer matrix: experimental and Density functional theory study. Appl Surf Sci 420:167–179
Hamed Mashhadzadeh A, Fereidoon A, Ahangari MG (2017) Atomistic modeling of interfacial interaction between polyvinyl chloride and polypropylene with Boron-Nitride monolayer sheet: a density functional theory study. Superlattice Microst 111:23–31
Rostamiyan Y et al (2013) Augmenting epoxy toughness by combination of both thermoplastic and nanolayered materials and using artificial intelligence techniques for modeling and optimization. J Polym Res 20(6):135
Hamed Mashhadzadeh A et al (2018) DFT study of Ni, Cu, Cd and Ag heavy metal atom adsorption onto the surface of the zinc-oxide nanotube and zinc-oxide graphene-like structure. Mater Chem Phys 220:366–373
Mashhadzadeh AH et al (2016) Investigation of heavy metal atoms adsorption onto graphene and graphdiyne surface: a density functional theory study. Superlattice Microst 100:1094–1102
Hamed Mashhadzadeh A et al (2018) Density functional theory study of adsorption properties of non-carbon, carbon and functionalized graphene surfaces towards the zinc and lead atoms. Phys E Low-dimens Syst Nanostruct 104:275–285
Sheng PX, Ting Y-P, Chen JP (2007) Biosorption of heavy metal ions (Pb, Cu, and Cd) from aqueous solutions by the marine alga Sargassum sp. in single- and multiple-metal systems. Ind Eng Chem Res 46(8):2438–2444
Srivastava NK, Majumder CB (2008) Novel biofiltration methods for the treatment of heavy metals from industrial wastewater. J Hazard Mater 151(1):1–8
Fu F, Wang Q (2011) Removal of heavy metal ions from wastewaters: a review. J Environ Manag 92(3):407–418
Harper TR, Kingham NW (1992) Removal of arsenic from wastewater using chemical precipitation methods. Water Environ Res 64(3):200–203
Li XZ, Zhao QL, Hao XD (1999) Ammonium removal from landfill leachate by chemical precipitation. Waste Manag 19(6):409–415
Zhang T, Ding L, Ren H (2009) Pretreatment of ammonium removal from landfill leachate by chemical precipitation. J Hazard Mater 166(2):911–915
Ghaemi N et al (2015) Polyethersulfone membrane enhanced with iron oxide nanoparticles for copper removal from water: application of new functionalized Fe3O4 nanoparticles. Chem Eng J 263:101–112
Yin J, Deng B (2015) Polymer-matrix nanocomposite membranes for water treatment. J Membr Sci 479:256–275
Tang W et al (2017) Optimization of sulfate removal from brackish water by membrane capacitive deionization (MCDI). Water Res 121:302–310
Leung WC et al (2000) Removal and recovery of heavy metals by bacteria isolated from activated sludge treating industrial effluents and municipal wastewater. Water Sci Technol 41(12):233–240
Gabarrón S et al (2016) Evaluation of emerging contaminants in a drinking water treatment plant using electrodialysis reversal technology. J Hazard Mater 309:192–201
Márquez F et al (2002) Observation of azo chromophore fluorescence and phosphorescence emissions from DBH by applying exclusively the orbital confinement effect in siliceous zeolites devoid of charge-balancing cations. J Am Chem Soc 124(25):7264–7265
Hamed Mashhadzadeh A et al (2019) Theoretical studies on the mechanical and electronic properties of 2D and 3D structures of beryllium-oxide graphene and graphene nanobud. Appl Surf Sci 476:36–48
Ahangari MG et al (2019) Effect of various defects on mechanical and electronic properties of zinc-oxide graphene-like structure: a DFT study. Vacuum 165:26–34
Ghorbanzadeh Ahangari M, Fereidoon A, Mashhadzadeh AH (2017) Interlayer interaction and mechanical properties in multi-layer graphene, Boron-Nitride, Aluminum-Nitride and Gallium-Nitride graphene-like structure: a quantum-mechanical DFT study. Superlattice Microst 112:30–45
Rostamiyan Y, Mohammadi V, Hamed Mashhadzadeh A (2020) Mechanical, electronic and stability properties of multi-walled beryllium oxide nanotubes and nanopeapods: a density functional theory study. J Mol Model 26(4):76
Ghorbanzadeh Ahangari M, Mashhadzadeh AH (2020) Density functional theory based molecular dynamics study on hydrogen storage capacity of C24, B12N12, Al12 N12, Be12O12, Mg12O12, and Zn12O12 nanocages. Int J Hydrog Energy 45(11):6745–6756
Ghorbanzadeh Ahangari M et al (2019) Density functional theory study on the mechanical properties and interlayer interactions of multi-layer graphene: carbonic, silicon-carbide and silicene graphene-like structures. Silicon 11(3):1235–1246
Hohenberg P, Kohn W (1964) Inhomogeneous electron gas. Phys Rev 136(3B):B864–B871
Kohn W (1999) Nobel lecture: electronic structure of matter\char22{}wave functions and density functionals. Rev Mod Phys 71(5):1253–1266
José MS et al (2002) The SIESTA method for ab initio order-N materials simulation. J Phys Condens Matter 14(11):2745
Perdew JP, Burke K, Ernzerhof M (1996) Generalized gradient approximation made simple. Phys Rev Lett 77(18):3865–3868
Pan H, Feng YP, Lin JY (2004) \textit{Ab initio} study of OH-functionalized single-wall carbon nanotubes. Phys Rev B 70(24):245425
Kim BG, Choi HJ (2012) Graphyne: hexagonal network of carbon with versatile Dirac cones. Phys Rev B 86(11):115435
Gao E, Xie B, Xu Z (2016) Two-dimensional silica: structural, mechanical properties, and strain-induced band gap tuning. J Appl Phys 119(1):014301
Nakada K, Ishii A (2011) Migration of adatom adsorption on graphene using DFT calculation. Solid State Commun 151(1):13–16
Author information
Authors and Affiliations
Corresponding author
Additional information
Publisher’s note
Springer Nature remains neutral with regard to jurisdictional claims in published maps and institutional affiliations.
Rights and permissions
About this article
Cite this article
Shahmoradi, A., Ghorbanzadeh Ahangari, M., Jahanshahi, M. et al. Adsorption of hazardous atoms on the surface of TON zeolite and bilayer silica: a DFT study. J Mol Model 26, 119 (2020). https://doi.org/10.1007/s00894-020-04381-w
Received:
Accepted:
Published:
DOI: https://doi.org/10.1007/s00894-020-04381-w