Abstract
Purpose
Isometric training and pre-activation are proven to enhance acceleration performance. However, traditional strength training exercises do not mirror the acceleration-specific activation patterns of the gluteal muscles, characterized by ipsilateral hip extension during contralateral hip flexion. Therefore, the aim of the study was to determine gluteal muscle activity of acceleration-specific exercises compared to traditional strength training exercises.
Methods
In a cross-sectional study design, the peak electromyographic activity of two acceleration-specific exercises was investigated and compared to two traditional strength training exercises each for the gluteus maximus and medius. Twenty-four participants from various athletic backgrounds (13 males, 11 females, 26 years, 178 cm, 77 kg) performed four gluteus maximus [half-kneeling glute squeeze (HKGS), resisted knee split (RKS), hip thrust (HT), split squat (SS)] and four gluteus medius [resisted prone hip abduction (RPHA), isometric clam (IC), side-plank with leg abduction (SP), resisted side-stepping (RSS)] exercises in a randomized order.
Results
The RKS (p = 0.011, d = 0.96) and the HKGS (p = 0.064, d = 0.68) elicited higher peak gluteus maximus activity than the SS with large and moderate effects, respectively. No significant differences (p > 0.05) were found between the HT, RKS and HKGS. The RPHA elicited significantly higher gluteus medius activity with a large effect compared to RSS (p < 0.001, d = 1.41) and a moderate effect relative to the SP (p = 0.002, d = 0.78).
Conclusion
The acceleration-specific exercises effectively activate the gluteal muscles for pre-activation and strength training purposes and might help improve horizontal acceleration due to their direct coordinative transfer.
Similar content being viewed by others
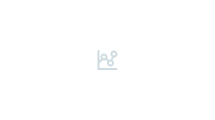
Avoid common mistakes on your manuscript.
Introduction
The interest in isometric training has risen over the last few years, both in sports practice and in research (Bartolomei et al. 2017; Cannon et al. 2022; Oranchuk et al. 2019). High-intensity isometric training allows long-term improvements in muscular activation, maximum force production (Oranchuk et al. 2019) and tendon stiffness (Bohm et al. 2015) as well as short-term performance enhancements after pre-activation tasks (Bartolomei et al. 2017; Seitz and Haff 2016; Wilson et al. 2013). Post-activation improvements have been identified in horizontal acceleration performance (Chatzopoulos et al. 2007; Smith et al. 2014) which is a key determinant in a large number of sports (Wild et al. 2022). A high coordinative transfer of the pre-activation task to horizontal acceleration is suggested to increase the benefits of post-activation potentiation (PAP) (Dello Iacono et al. 2018; Smith et al. 2014). However, most of the traditional pre-activation and strength training exercises such as squats, step-ups, hip thrusts, split squats, or Olympic weightlifting variations do not reflect the specific activation patterns that are present during horizontal acceleration. The term ’acceleration-specificity’ implies high muscle activity, high tendon stretch and the synergistic interaction of both legs during a sports-specific joint angle configuration, i.e. ipsilateral hip extension with high contralateral hip flexion (’hip-extensor driven’ movement pattern).
The thigh separation angle is described as a crucial factor for horizontal power generation (Walker et al. 2021). Hip flexor co-contraction causes reciprocal inhibition of the gluteus maximus (GMAX) in the stance leg. This impairs ipsilateral hip extension and contralateral hip flexion which results in a decreased thigh separation (Mills et al. 2015). Higher GMAX activity is suggested to decrease co-contraction of the hip flexor muscles and thus enhance the thigh separation angle (Neumann 2010). A recently published framework introduced the half-kneeling glute squeeze exercise to improve the acceleration-specific GMAX activation (Alt et al. 2022). Although the half-kneeling glute squeeze and the similar resisted knee split exercise are practically approved by elite Olympic athletes from different sports, they have not yet been examined.
A major synergistic muscle to support hip extension is the gluteus medius (GMED) (Neumann 2010), which has been identified as an important generator of horizontal forces during accelerated sprinting (Pandy et al. 2021). Therefore, it is imperative to improve his proper function to enhance acceleration-specific activation patterns. However, most training approaches for the GMED are based on low intensities and high volume instead of high activity levels (Macadam et al. 2015; Moore et al. 2020). Consequently, the resisted prone hip abduction was designed and the isometric clam exercise was modified to enable high GMED intensities. This might help athletes to gradually modulate activity levels and thus enhance their range of motion of active thigh separation.
In addition to performance enhancement, high GMAX and GMED activity is important for effective prevention and rehabilitation of lower extremity injuries (Chumanov et al. 2012; Distefano et al. 2009; Mills et al. 2015). Reciprocal inhibition of the GMAX and weakness of the GMED have been shown to increase the injury risk of the anterior cruciate ligament, the hip flexor, hamstring and adductor muscles, among others (Mills et al. 2015; Presswood et al. 2008). Furthermore, the acceleration-specific exercises could help elicit high GMAX and GMED activity with low joint stress, which might help maintain gluteal strength after lower extremity injuries (Cambridge et al. 2012). Due to the minimal equipment requirements, they can easily be incorporated in rehabilitation and pre-activation settings at any facility with a high number of athletes in a time-efficient manner.
To the best of our knowledge, no study has investigated muscular activity during these exercises. The objective of this investigation is to examine the gluteal activity of the four acceleration-specific exercises and to compare the GMAX exercises to the hip thrust and split squat and the GMED exercises to the side-plank with leg abduction and resisted side-stepping. The hypothesis is as follows:
Peak muscle activity of GMAX and GMED is higher in acceleration-specific exercises compared to traditional strength training exercises.
Methods
Participants
Twenty-four participants (25.6 ± 3.5 years, 177.7 ± 9.6 cm, 76.7 ± 13.9 kg, 13 males, 11 females) from various athletic backgrounds (supplementary data A.1) with a mean strength training experience of 5.5 ± 3.0 years were recruited for the investigation. Inclusion criteria required that participants were at least 18 years of age, were not acutely affected by any form of injuries, had at least one year of strength training experience and performed regular training two to three times a week. All participants were informed about the aims of the study, the procedure of electromyography (EMG) and provided written consent to their voluntary participation. The investigation was approved by the local ethics commission of the German Sport University Cologne (No. 168/2021) and met all requirements of the Declaration of Helsinki.
Study design
A cross-sectional within-subjects design was used to compare muscle activity of the gluteal muscles between two traditional strength training and two acceleration-specific exercises for both, GMAX and GMED. For GMAX, the hip thrust and split squat were performed as traditional exercises and compared with the acceleration-specific exercises half-kneeling glute squeeze and resisted knee split (Fig. 1, Table 1). The traditional GMED exercises side-plank with leg abduction and resisted side-stepping were compared with the acceleration-specific resisted prone hip abduction and isometric clam (Fig. 2, Table 1). Detailed information about the standardized criteria is provided in the supplementary materials (A.2). All participants completed two sessions separated by at least 5 and a maximum of 10 days.
Representative illustration of the four gluteus maximus exercises: A Half-kneeling glute squeeze, static phase one (left), increasing hip flexion in phase two (middle) and extension of the dominant leg in phase three (right); B resisted knee split; C split squat, starting position (left) and position after the eccentric phase (right); D hip thrust, starting (left), isometric hold (middle) and end position (right)
Exemplary setup for the four gluteus medius exercises: A Resisted prone hip abduction, starting position (left), position between eccentric and concentric phase (middle) and isometric hold and end position (right); B isometric clam with inclined pelvic position; C side-plank with leg abduction, adducted (left) and abducted position (right); D resisted side-stepping, starting position (left), position after abduction (middle) and reproduced starting position (right)
The familiarization session started with the collection of anthropometric data (weight, height, leg length, shoulder width) and a standardized warm-up. The warm-up included 5 min of self-paced low-intensity jogging and a dynamic movement preparation focusing the lower body. The participant’s three-repetition maximum (3-RM) was tested for the hip thrust and the split squat in randomized order according to the National Strength and Conditioning Association (Haff and Triplett 2016). Furthermore, the participants were familiarized with the other six exercises in detail until the examiner confirmed the quality of execution.
In the experimental session, the same warm-up was applied before the electrodes were placed and a quality check of the signal was conducted. Subsequently, maximum voluntary isometric contraction (MVIC) trials were performed. According to the results of block randomization, participants started with either the GMAX or GMED block. Each block started with the MVIC trials of the respective muscle followed by the four exercises in a randomized order. After the first block, the MVIC testing and the four exercises in a randomized order of the second muscle were performed. To reduce intra-session fatigue and promote maximum possible activity levels, the participants performed each exercise only once as long the form was accurate, and the EMG signal was recorded correctly. Between exercises, four minutes of rest were provided. Prior to the hip thrust and the split squat, a set of three repetitions was conducted with 60% of the 3-RM load. For all exercises, the EMG activity of the dominant limb was recorded. A speaker provided a standardized timer to ensure accurate time under tension (Table 1).
Electromyography
Data recording
Wireless surface EMG electrodes (Delsys® TrignoTM, Boston, MA) were used to record muscle activity. Skin preparation and electrode placement were conducted according to the guidelines of the project for surface electromyography for the non-invasive assessment of muscles (SENIAM) on the dominant limb (Hermens et al. 2000). The skin was shaved and slightly roughened with a hand razor and sterilized with alcohol before the electrodes were fixed using double-sided tape and Fixomull stretch (BSN medical, Hamburg, Germany).
For GMAX MVIC testing, participants lay in the prone position and extended their hip against manual resistance applied to the distal end of the thigh with 90° of knee flexion (Hermens et al. 2000; Worrell et al. 2001). GMED MVIC was determined in a side-lying position with the lower hip and knee joint in 30° of flexion for increased stability. The upper leg was elevated to approximately 10° before performing maximum abduction against manual resistance applied to the distal end of the thigh and the shank (Hermens et al. 2000; Widler et al. 2009). For both muscles, three attempts of 5 s were conducted under verbal encouragement of the examiner with 60 s of recovery after each trial (Boren et al. 2011).
Data processing
EMGworks® Acquisition (Delsys, Natick, MA, USA) was used to record the 2000 Hz electromyographic signal, which was exported as raw data and imported to R-Studio (Version 1.4.1106, PBC, Boston, USA) for further processing. The surface EMG raw data was rectified, band pass filtered between 20 and 500 Hz using a Butterworth 4th order recursive filter (Eliassen et al. 2018) and smoothed using root-mean-square with a 100 ms window. Peak data of the exercises were normalized to a mean of a 1000 ms window from the peak value of the three MVIC trials (Contreras et al. 2015).
Statistical analysis
All calculations were performed using R-Studio. EMG data are presented as mean and 95% confidence interval (CI) of the peak %MVICs. Statistical significance was set at p ≤ 0.05. For all data, normal distribution was assessed by the Shapiro–Wilk test and sphericity of data was tested using Mauchly’s test. A one-way repeated-measures analysis of variance (ANOVA) was used to analyze the differences between exercises. Partial eta squared (ηpp2) was calculated with 95% CI as effect size and interpreted upon the guidelines of Cohen with ηp2 ≥ 0.26 large; 0.26–0.13 moderate; 0.13–0.02 small; < 0.02 negligible (Cohen 2013). Bonferroni post hoc tests were used for pairwise comparisons between exercises. Cohen’s d effect sizes are reported with 95% CI for all pairwise comparisons interpreted as d ≥ 0.8 large; 0.8–0.5 moderate; 0.5–0.2 small; < 0.2 negligible (Cohen 2013).
Results
For the two traditional strength training and the two acceleration-specific exercises of each muscle, the EMG data of all n = 24 participants were analyzed and included. The mean load for the 3-RM hip thrust (154 ± 51 kg) was twice as high as for the split squat (77 ± 28 kg, p < 0.001). EMG data are displayed as peak activity with 95% CI averaged across all participants (Table 2) and individual peak activities expressed as %MVIC (Table 3, Fig. 3). The time courses of the hip thrust, resisted prone hip abduction and half-kneeling glute squeeze are presented as mean ± standard deviation to identify the different phases of the exercises (Fig. 4).
Individual peak electromyographic activities expressed as a percentage of maximum voluntary isometric contraction with mean and 95% confidence interval for all gluteus maximus (A) and gluteus medius (B) exercises. The acceleration-specific exercises are emphasized in red, the traditional strength training exercises in blue. *Significant difference with p ≤ 0.05; d = Cohen’s d effect size
Gluteus maximus
For the GMAX, one-way repeated-measures ANOVA revealed a significant main effect for the factor exercise with a large effect size (ηp2 = 0.34, 95% CI = 0.16–0.48; p < 0.001). No significant differences were found between the hip thrust, resisted knee split and half-kneeling glute squeeze. The hip thrust (d = 1.40, 95% CI = 0.74–2.06; p < 0.001) and the resisted knee split (d = 0.96, 95% CI = 0.30–1.62; p = 0.011) resulted in significantly higher peak GMAX activity than the split squat with large effect sizes. The half-kneeling glute squeeze failed statistical significance but showed a moderate effect size compared to the split squat (d = 0.68, 95% CI = 0.13–1.22; p = 0.064) (Table 2, Fig. 3A). The intra-individual analysis for the GMAX exercises demonstrated that 42% of the participants achieved the highest activity in one of the acceleration-specific exercises. Twelve participants elicited peak activity in the hip thrust, six participants in the resisted knee split, four participants in the half-kneeling glute squeeze and two participants in the split squat (Table 3).
Gluteus medius
Concerning the GMED, one-way repeated-measures ANOVA revealed a significant main effect for the factor exercise with a large effect size (ηp2 = 0.39, 95% CI = 0.20–0.53; p < 0.001). The resisted prone hip abduction demonstrated significantly higher peak activity than resisted side-stepping (d = 1.41, 95% CI = 0.80–2.01; p < 0.001), the side-plank with leg abduction (d = 0.78, 95% CI = 0.36–1.20, p = 0.0016) and the isometric clam (d = 1.01, 95% CI = 0.44–1.58; p = 0.0014). The side-plank revealed significantly higher peak activity than resisted side-stepping (d = 0.71, 95% CI = 0.17–1.24; p = 0.0395) (Table 2, Fig. 3B). The highest activity of the GMED was generated in 83% of the participants during one of the acceleration-specific exercises (18 resisted prone hip abduction, 3 side-plank with leg abduction, 2 isometric clam, 1 resisted side-stepping) (Table 3).
Discussion
The current investigation examined the EMG activity of four acceleration-specific exercises for the GMAX and GMED. For each muscle, two traditional strength training exercises were compared to two acceleration-specific exercises. The hypothesis was that peak EMG activity is higher during the acceleration-specific compared to the traditional strength training exercises. The highest peak GMAX activity averaged over all participants was found for the hip thrust (143% MVIC) with no significant difference to the resisted knee split (128% MVIC) and half-kneeling glute squeeze (125% MVIC). The hip thrust and the resisted knee split elicited significantly higher peak EMG activity than the split squat (100% MVIC) with large effect sizes (Table 2, Fig. 3A). Therefore, the hypothesis was not confirmed for GMAX. However, 42% of all participants achieved the highest peak GMAX activity during one of the acceleration-specific exercises (Table 3). For GMED, the hypothesis was approved because the resisted prone hip abduction elicited the highest peak EMG activity (149% MVIC) with a significant difference and large effect compared to resisted side-stepping (93% MVIC) and moderate effect compared to the side-plank with leg abduction (118% MVIC) (Table 2, Fig. 3B).
Acceleration-specific gluteus maximus activation
The high GMAX activity during the acceleration-specific exercises is very promising, especially in terms of the high coordinative transfer to horizontal acceleration, which is recommended for pre-activation and strength training tasks (Smith et al. 2014; Walker et al. 2021). The resisted knee split is performed solely isometric and supports the acceleration-specific position by adding isometric contraction of the contralateral hip flexor muscles during maximum activation of the GMAX (Fig. 1B). The half-kneeling glute squeeze consists of three phases with an increasing contralateral hip flexion during the second phase. This dynamic movement makes it more difficult to achieve a sustained high level of GMAX activity which explains the decreasing activity in phase two. Mean peak activity occurs in the third phase when the dominant knee is extended (Fig. 4B).
This final position of the half-kneeling glute squeeze induces a large thigh separation and a low trunk angle, which have been demonstrated as vital components of enhanced acceleration performance (Walker et al. 2021). The thigh separation angle depends on the range of motion of hip extension in the stance leg and concomitant hip flexion in the swing leg (Alt et al. 2022). Co-contraction of the antagonistic muscles during activation of the agonistic muscles is a common phenomenon (Frey-Law and Avin 2013). The theory of muscular stabilization is a potential mechanism responsible for the increase in hip flexor co-contraction during GMAX activation with increasing separation of the thighs (Thorborg et al. 2016). High iliacus activity in the late stance phase (Andersson et al. 1997) and high psoas major activity during contralateral hip flexion (Hu et al. 2010) have been found in past investigations. Reciprocal inhibition results in decreased GMAX activity in the stance leg and impairs hip flexion in the swing leg (Mills et al. 2015). The decreasing GMAX activity during increasing contralateral hip flexion in the second phase of the half-kneeling glute squeeze emphasized this distinctive activation pattern (Fig. 4B). Both, lower GMAX activity and decreased hip flexion range of motion result in a smaller thigh separation angle and might impair performance. Reduced hip flexor tightness enhanced GMAX activity, pelvic position and performance (Konrad et al. 2021).
The acceleration-specific exercises focus on ’reciprocal activation’ of the GMAX which is intended to enhance the following characteristics:
-
(1)
Ipsilateral hip extension: Higher levels of GMAX activity enable improved control of ipsilateral hip extension through reduced co-contraction of the ipsilateral hip flexor muscles (Mills et al. 2015; Neumann 2010).
-
(2)
Contralateral hip flexion: The decrease in hip flexor co-contraction is suggested to enable an increase in contralateral hip flexion range of motion due to improved muscular stabilization of the pelvis (Thorborg et al. 2016). This might further support a decrease in activity of the adductor magnus, which functions as a hip extensor, especially when the hip is in a flexed position (Ward et al. 2010). The adductor magnus has been shown to remain strongly excitated during the swing phase which might inhibit hip flexion range of motion (Gazendam and Hof 2007).
-
(3)
Anterior pelvic tilt: The sustained high level of GMAX activity is suggested to enhance dynamic control of the anterior pelvic tilt (Neumann 2010), which is a common consequence of insufficient hip extension capability during maximal sprinting (Schache et al. 2000). High pelvic control through a synergistic interaction of concomitant hip flexion and extension might improve the trunk position during sprinting, which results in a more horizontally orientated force vector (Walker et al. 2021).
This simultaneous enhancement of ipsilateral hip extension and contralateral hip flexion ability could improve both the thigh separation angle and ‘scissor-action’ of the thighs due to increased thigh angular velocity (Clark et al. 2020).
Furthermore, reciprocal inhibition of the GMAX has been shown to increase the risk of injury to the hamstring and adductor muscles and anterior cruciate ligament through synergistic dominance. Increasing the GMAX activity may help reduce the reliance on the secondary hip extensors and improve the balance between the hamstring, hip adductor and gluteal muscles in generating hip extension torque (Mills et al. 2015). In addition, a decrease in hip flexor co-contraction is thought to reduce the injury prevalence of the hip flexors. Overuse injuries are very common during sports that require maximal sprinting and are exacerbated by reciprocal inhibition of the GMAX (Thorborg et al. 2016).
The previously mentioned acceleration-specific activation patterns are not mirrored in traditional strength training exercises. However, the hip thrust and the split squat elicited high GMAX activity, which is in line with past findings (Contreras et al. 2015; Neto et al. 2020; Williams et al. 2021). The horizontal force-vector direction and the peak activity occurring during the isometric phase with a neutral hip position (Fig. 4A) support the use of the hip thrust in strength training programs to improve horizontal acceleration (Loturco et al. 2018; Williams et al. 2021). The free barbell split squat demands a high level of core and lower limb stability which might have caused lower GMAX activity in some participants (Macadam and Feser 2019).
Higher peak gluteus medius activity during acceleration-specific exercises
Of all participants, 83% achieved the highest peak activity in the GMED during one of the acceleration-specific exercises (Table 3), which supports their use to elicit high-intensity muscle contractions. These high intensities of the GMED are important for horizontal acceleration performance for the following threereasons:
-
(1)
Hip stabilization: The GMED is the most important hip stabilizer in the frontal plane (Neumann 2010). A lack of hip stabilization during the stance phase causes a lateral tilt of the pelvis, causing a shift of the body’s center of mass that impairs force transmission of the lower limb and increases the risk of injuries (Presswood et al. 2008).
-
(2)
Activation ratio between abductors and adductors: Groin injuries among athletes caused by overuse are very common (Eckard et al. 2017). Higher GMED activity in the late swing phase was associated with lower peak adduction angles in the early stance phase (Chumanov et al. 2012). It is suggested that high GMED activity during sprinting improves the adductor-abductor ratio and lowers the risk of overuse injuries of the adductor muscles. Furthermore, this might help increase the thigh separation angle due to improved hip flexion range of motion in the swing leg as described earlier in this paper.
-
(3)
Secondary hip extensor: The GMED functions as a secondary hip extensor and thus supports high GMAX activation (Neumann 2010). This has been shown to be essential during accelerated sprinting, as the GMED acts as an important generator of propulsive impulses (Pandy et al. 2021).
The mean activity during the resisted prone hip abduction was found to be high during the entire time under tension, irrespective of muscle length and type of contraction (Fig. 4C). During sprinting, the GMED primarily works isometrically in a position of approximately 10° of adduction (Neumann 2010). Consequently, the exercise can easily be adjusted to highly activate the GMED during an extended and adducted hip position as it is necessary for horizontal acceleration.
The contraction of the GMED during the isometric clam exercise is primarily induced by external rotation. This supports a higher GMED activity compared to the tensor fasciae latae (Selkowitz et al. 2013). A higher inclination of the pelvis improves GMED activity during the clam exercise (Willcox and Burden 2013). Therefore, the isometric clam in this investigation was performed with a high inclination of the pelvis (Fig. 2B). However, performing maximum isometric external rotation without reclining the pelvis was challenging for some participants, which may have limited higher activity levels. The peak GMED activity of the isometric clam might increase when athletes are familiar with the exercise.
The high muscle activity during the side plank with leg abduction confirms the results of past investigations (Boren et al. 2011). The isometric GMED contraction in the lower leg is intensified by the upward acceleration of the non-dominant leg during abduction when compared to normal side planks (Ekstrom et al. 2007). The high muscular activity and the stabilizing role in a neutral adducted and extended hip position make the exercise interesting for sprint training approaches (Hamner et al. 2010). With the modification of abduction speed and external loads, the side plank with leg abduction might be customized to suit different training concepts of the GMED. The resisted side-stepping elicited the lowest peak GMED activity (93% MVIC), although it was performed with the best possible execution criteria concerning side-step length and resistance. Therefore, it seems reasonable to suggest that it is hardly possible to achieve higher muscular activities during this exercise than in the present investigation. The resisted side-stepping might be an appropriate exercise in rehabilitation settings, but not to highly activate the GMED in athletes.
Enhanced acceleration performance after gluteal pre-activation
The investigation confirmed that the acceleration-specific exercises elicited very high muscle activity for both GMAX and GMED. Accordingly, they might be suited for high-intensity pre-activation in training sessions and competitions. Especially in sports that require the generation of maximum power, such as multiple track and field disciplines, but also in team sports such as soccer, American football, basketball or ice hockey, the use of PAP in competitions could be a crucial factor for performance (DeRenne 2010). A high association between hip thrust pre-activation and the acceleration phase (up to 10 m) supports the effect of GMAX PAP on horizontal acceleration (Loturco et al. 2018; Neto et al. 2019). Dello Iacono et al. (2018) described the importance of sport-specific pre-activation tasks and supported the use of hip thrusts before sprinting due to their horizontal force vectors. The acceleration-specific exercises for the GMAX are highly specific and might therefore be even more beneficial as pre-activation tasks. Furthermore, as demonstrated in this study, very high external loads are required to elicit the highest amount of muscle activity with the hip thrust or similar strength training exercises (mean 3-RM load: 154 ± 51 kg) (Dello Iacono et al. 2018). This is usually possible in separate strength training sessions, but prior to sprinting sessions or even competitions, the high amount of equipment might be a logistical challenge. The introduced acceleration-specific exercises require little equipment and setup and might therefore be preferential for pre-activation tasks. Furthermore, a high number of athletes can simultaneously perform the exercises, which is of great advantage for team sports. The acceleration-specific exercises can complement the warm-up process by increasing activation levels to maximum pre-activation and thus provide an appropriate balance between potentiation and accumulating fatigue (Rassier and Macintosh 2000; Seitz and Haff 2016).
The acceleration-specific exercises in daily training routines
Besides the use as pre-activation, the acceleration-specific exercises are also intended to be used in daily training routines to strengthen the gluteal muscles and improve task-specific activation patterns. Isometric training has been shown to elicit neurological and morphological adaptations (Oranchuk et al. 2019), which also transfer to dynamic movement tasks (Burgess et al. 2007). Significant improvements in GMAX activity during double-leg and single-leg squats after an isometric activation program have been demonstrated (Cannon et al. 2022). The acceleration-specific exercises are not intended to replace traditional strength training exercises with external loads, but to act mutually supportive. They reveal new opportunities to improve acceleration-specific activation, maximum strength of GMAX and GMED and lower extremity injury prevention. Due to the high muscle activity, they might also support tendon adaptations and increase muscular stiffness to improve the transmission of force between muscle and bone (Brazier et al. 2019; Oranchuk et al. 2019).
The findings of this study are also of interest for rehabilitation. Especially in the early stages of rehabilitation, high-intensity isometric contractions have been suggested to improve and maintain motor unit recruitment. Due to higher voluntary muscle activations compared to concentric and eccentric contractions, isometric training could help prevent post-injury activation deficits (Macdonald et al. 2019). The acceleration-specific exercises enable voluntary activation at various activity levels up to maximum intensities with very low joint stress. Therefore, they might help maintain gluteal strength and neuromuscular control after hip joint or hamstring injuries.
Limitations and perspectives
The results of this study rely on a very heterogeneous group of participants from various athletic backgrounds with different performance levels and strength training experience (supplementary data A.1). The participants had only one familiarization session and were not used to the acceleration-specific or similar exercises. In addition, only one set of each exercise was performed during the experimental session due to the risk of accumulating fatigue. Especially the half-kneeling glute squeeze and the isometric clam revealed a high inter-individual variability which is suggested to be caused by the high coordinative demands (Table 3). A more homogeneous sample with high-performance athletes who are experienced in these exercises could specify the results.
This investigation did not determine hip flexor muscle activity, because it is hardly possible with surface EMG (Andersson et al. 1997; Hu et al. 2010). However, the extent of hip flexor co-contraction in the maximum possible thigh separation angle and its alteration through acceleration-specific exercises should be investigated. Furthermore, the transfer of the exercises to horizontal acceleration is not yet known. An intervention study is needed to examine the effects of the acceleration-specific exercises on GMAX and intramuscular hip flexor EMG activity during acceleration as well as maximum velocity sprints, and its influence on performance. In addition, potential acceleration performance enhancements after pre-activation with these exercises and the influence on overuse injury prevalence of the hip flexor, hamstring and adductor muscles should be part of further investigations. In case of additional evidence of the acceleration-specific exercises, transferring this training approach to other muscle groups like the hamstrings, calves or shoulders seems feasible.
Seven out of eight exercises determined in this investigation achieved values greater than 100% of MVIC. Although similar results for peak EMG values were found in past investigations (Boren et al. 2011; Contreras et al. 2015; Williams et al. 2021), the MVIC testing positions should be questioned to allow more reliable classifications of exercise intensities. Concerning normalization methods, but also for the acceleration-specific exercises, visual feedback should be considered. Providing visual feedback in the form of a real-time EMG activity graph while voluntary activations are performed has been shown to increase activity levels (Amagliani et al. 2010). In addition to visual feedback, surface EMG could also be used as a training tool to ascertain which exercises work best for each individual and where the greatest potentials are in terms of acceleration-specific activation patterns. Further research with a more detailed analysis of inter-individual variabilities is needed to determine adaptations after exercises that elicit different EMG activities.
Conclusion
Despite the lack of participants’ experience in the acceleration-specific exercises, 42% and 83% achieved the highest peak activity of the GMAX and GMED, respectively, during one of these exercises. Ipsilateral hip extension and concomitant contralateral hip flexion decisively determine the thigh separation angle, which is essential for horizontal acceleration performance. In contrast to traditional strength training, the acceleration-specific exercises mirror this specific activation pattern of the gluteal muscles, which is suggested to transfer to horizontal acceleration. Given the high activity levels with low joint stress and minimal equipment requirements, the exercises appear to be suitable for task-specific pre-activation, strengthening and rehabilitation of the gluteal muscles. In addition, they might support the prevention of lower extremity injuries due to improved activation ratios of the hip muscles. After the acceleration-specific exercises have been practically approved, this study provides the first scientific findings. Further intervention studies are needed to examine the direct transfer to acceleration performance after training and pre-activation with the acceleration-specific exercises.
Data availability
A data availability statement is not applicable, however data is provided in the supplementary information.
Abbreviations
- 3-RM:
-
Three-repetition maximum
- ANOVA:
-
Analysis of variance
- CI:
-
Confidence interval
- EMG:
-
Electromyography
- GMAX:
-
Gluteus maximus
- GMED:
-
Gluteus medius
- MVIC:
-
Maximum voluntary isometric contraction
- PAP:
-
Post-activation potentiation
- SENIAM:
-
Surface electromyography for the non-invasive assessment of muscles
References
Alt T, Oeppert TJ, Zedler M, Goldmann J-P, Braunstein B, Willwacher S (2022) A novel guideline for the analysis of linear acceleration mechanics—outlining a conceptual framework of ‘shin roll’ motion. Sports Biomech. https://doi.org/10.1080/14763141.2022.2094827
Amagliani RM, Peterella JK, Jung AP (2010) Type of encouragement influences peak muscle force in college-age women. Int J Exerc Sci 3(4):165–173
Andersson EA, Nilsson J, Thorstensson A (1997) Intramuscular EMG from the hip flexor muscles during human locomotion. Acta Physiol Scand 161(3):361–370. https://doi.org/10.1046/j.1365-201X.1997.00225.x
Bartolomei S, Fukuda DH, Hoffman JR, Stout JR, Merni F (2017) The influence of isometric preload on power expressed during bench press in strength-trained men. Eur J Sport Sci 17(2):195–199. https://doi.org/10.1080/17461391.2016.1222000
Bohm S, Mersmann F, Arampatzis A (2015) Human tendon adaptation in response to mechanical loading: a systematic review and meta-analysis of exercise intervention studies on healthy adults. Sports Med - Open 1(1):7. https://doi.org/10.1186/s40798-015-0009-9
Boren K, Conrey C, Le Coguic J, Paprocki L, Voight M, Robinson TK (2011) Electromyographic analysis of gluteus medius and gluteus maximus during rehabilitation exercises. Int J Sports Phys Ther 6(3):206–223
Brazier J, Maloney S, Bishop C, Read PJ, Turner AN (2019) Lower extremity stiffness: considerations for testing, performance enhancement, and injury risk. J Strength Cond Res 33(4):1156–1166. https://doi.org/10.1519/JSC.0000000000002283
Burgess KE, Connick MJ, Graham-Smith P, Pearson SJ (2007) Plyometric vs. isometric training influences on tendon properties and muscle output. J Strength Cond Res 21(3):986–989. https://doi.org/10.1519/R-20235.1
Cambridge EDJ, Sidorkewicz N, Ikeda DM, McGill SM (2012) Progressive hip rehabilitation: the effects of resistance band placement on gluteal activation during two common exercises. Clin Biomech (Bristol, Avon) 27(7):719–724. https://doi.org/10.1016/j.clinbiomech.2012.03.002
Cannon J, Weithman BA, Powers CM (2022) Activation training facilitates gluteus maximus recruitment during weight-bearing strengthening exercises. J Electromyogr Kinesiol : off J Int Soc Electrophysiol Kinesiol 63:102643. https://doi.org/10.1016/j.jelekin.2022.102643
Chatzopoulos DE, Michailidis CJ, Giannakos AK, Alexiou KC, Patikas DA, Antonopoulos CB, Kotzamanidis CM (2007) Postactivation potentiation effects after heavy resistance exercise on running speed. J Strength Cond Res 21(4):1278–1281. https://doi.org/10.1519/R-21276.1
Chumanov ES, Wille CM, Michalski MP, Heiderscheit BC (2012) Changes in muscle activation patterns when running step rate is increased. Gait Posture 36(2):231–235. https://doi.org/10.1016/j.gaitpost.2012.02.023
Clark KP, Meng CR, Stearne DJ (2020) “Whip from the hip”: thigh angular motion, ground contact mechanics, and running speed. Biol Open. https://doi.org/10.1242/bio.053546
Cohen J (2013) Statistical power analysis for the behavioral sciences. Routledge
Contreras B, Vigotsky AD, Schoenfeld BJ, Beardsley C, Cronin J (2015) A comparison of gluteus maximus, biceps femoris, and vastus lateralis electromyographic activity in the back squat and barbell hip thrust exercises. J Appl Biomech 31(6):452–458. https://doi.org/10.1123/jab.2014-0301
Dello Iacono A, Padulo J, Seitz LD (2018) Loaded hip thrust-based PAP protocol effects on acceleration and sprint performance of handball players. J Sports Sci 36(11):1269–1276. https://doi.org/10.1080/02640414.2017.1374657
DeRenne C (2010) Effects of postactivation potentiation warm-up in male and female sport performances: a brief review. Strength Conditio J 32(6):58–64. https://doi.org/10.1519/SSC.0b013e3181f412c4
Distefano LJ, Blackburn JT, Marshall SW, Padua DA (2009) Gluteal muscle activation during common therapeutic exercises. J Orthop Sports Phys Ther 39(7):532–540. https://doi.org/10.2519/jospt.2009.2796
Eckard TG, Padua DA, Dompier TP, Dalton SL, Thorborg K, Kerr ZY (2017) Epidemiology of hip flexor and hip adductor strains in national collegiate athletic association athletes, 2009/2010-2014/2015. Am J Sports Med 45(12):2713–2722. https://doi.org/10.1177/0363546517716179
Ekstrom RA, Donatelli RA, Carp KC (2007) Electromyographic analysis of core trunk, hip, and thigh muscles during 9 rehabilitation exercises. J Orthop Sports Phys Ther 37(12):754–762. https://doi.org/10.2519/jospt.2007.2471
Eliassen W, Saeterbakken AH, van den Tillaar R (2018) Comparison of bilateral and unilateral squat exercises on barbell kinematics and muscle activation. Intl J Sports Phys Ther 13(5):871–881. https://doi.org/10.26603/ijspt20180871
Frey-Law LA, Avin KG (2013) Muscle coactivation: a generalized or localized motor control strategy? Muscle Nerve 48(4):578–585. https://doi.org/10.1002/mus.23801
Gazendam MGJ, Hof AL (2007) Averaged EMG profiles in jogging and running at different speeds. Gait Posture 25(4):604–614. https://doi.org/10.1016/j.gaitpost.2006.06.013
Haff G, Triplett NT (eds) (2016) Essentials of strength training and conditioning, 4th edn. Human Kinetics, Champaign, US
Hamner SR, Seth A, Delp SL (2010) Muscle contributions to propulsion and support during running. J Biomech 43(14):2709–2716. https://doi.org/10.1016/j.jbiomech.2010.06.025
Hermens HJ, Freriks B, Disselhorst-Klug C, Rau G (2000) Development of recommendations for SEMG sensors and sensor placement procedures. J Electromyogr Kinesiol: off J Int Soc Electrophysiol Kinesiol 10(5):361–374. https://doi.org/10.1016/s1050-6411(00)00027-4
Hu H, Meijer OG, van Dieën JH, Hodges PW, Bruijn SM, Strijers RL, Nanayakkara PW, van Royen BJ, Wu W, Xia C (2010) Muscle activity during the active straight leg raise (ASLR), and the effects of a pelvic belt on the ASLR and on treadmill walking. J Biomech 43(3):532–539. https://doi.org/10.1016/j.jbiomech.2009.09.035
Konrad A, Močnik R, Titze S, Nakamura M, Tilp M (2021) The influence of stretching the hip flexor muscles on performance parameters. a systematic review with meta-analysis. Int J Environ Res Public Health 18(4):1936. https://doi.org/10.3390/ijerph18041936
Loturco I, Contreras B, Kobal R, Fernandes V, Moura N, Siqueira F, Winckler C, Suchomel T, Pereira LA (2018) Vertically and horizontally directed muscle power exercises: relationships with top-level sprint performance. PLoS ONE 13(7):e0201475. https://doi.org/10.1371/journal.pone.0201475
Macadam P, Feser EH (2019) Examination of gluteus maximus electromyographic excitation associated with dynamic hip extension during body weight exercise: a systematic review. Intl J Sports Phys Ther 14(1):14–31. https://doi.org/10.26603/ijspt20190014
Macadam P, Cronin J, Contreras B (2015) An examination of the gluteal muscle activity associated with dynamic hip abduction and hip external rotation exercise: a systematic review. Int J Sports Phys Ther 10(5):573–591
Macdonald B, McAleer S, Kelly S, Chakraverty R, Johnston M, Pollock N (2019) Hamstring rehabilitation in elite track and field athletes: applying the British athletics muscle injury classification in clinical practice. Br J Sports Med 53(23):1464–1473. https://doi.org/10.1136/bjsports-2017-098971
Mills M, Frank B, Goto S, Blackburn T, Cates S, Clark M, Aguilar A, Fava N, Padua D (2015) Effect of restricted hip flexor muscle length on hip extensor muscle activity and lower extremity biomechanics in college-aged female soccer players. Int J Sports Phys Ther 10(7):946–954
Moore D, Semciw AI, Pizzari T (2020) A systematic review and meta-analysis of common therapeutic exercises that generate highest muscle activity in the gluteus medius and gluteus minimus segments. Int J Sports Phys Ther 15(6):856–881. https://doi.org/10.26603/ijspt20200856
Neto WK, Vieira TL, Gama EF (2019) Barbell hip thrust, muscular activation and performance: a systematic review. J Sports Sci Med 18(2):198–206
Neto WK, Soares EG, Vieira TL, Aguiar R, Chola TA, Sampaio VdL, Gama EF (2020) Gluteus maximus activation during common strength and hypertrophy exercises: a systematic review. J Sports Sci Med 19(1):195–203
Neumann DA (2010) Kinesiology of the hip: a focus on muscular actions. J Orthop Sports Phys Ther 40(2):82–94. https://doi.org/10.2519/jospt.2010.3025
Oranchuk DJ, Storey AG, Nelson AR, Cronin JB (2019) Isometric training and long-term adaptations: effects of muscle length, intensity, and intent: a systematic review. Scand J Med Sci Sports 29(4):484–503. https://doi.org/10.1111/sms.13375
Pandy MG, Lai AKM, Schache AG, Lin Y-C (2021) How muscles maximize performance in accelerated sprinting. Scand J Med Sci Sports 31(10):1882–1896. https://doi.org/10.1111/sms.14021
Presswood L, Cronin J, Keogh JWL, Whatman C (2008) Gluteus medius: applied anatomy, dysfunction, assessment, and progressive strengthening. Strength Cond J 30(5):41–53. https://doi.org/10.1519/SSC.0b013e318187f19a
Rassier DE, Macintosh BR (2000) Coexistence of potentiation and fatigue in skeletal muscle. Braz J Med Biol Res 33(5):499–508. https://doi.org/10.1590/s0100-879x2000000500003
Schache AG, Blanch PD, Murphy AT (2000) Relation of anterior pelvic tilt during running to clinical and kinematic measures of hip extension. Br J Sports Med 34(4):279–283. https://doi.org/10.1136/bjsm.34.4.279
Seitz LB, Haff GG (2016) Factors modulating post-activation potentiation of jump, sprint, throw, and upper-body ballistic performances: a systematic review with meta-analysis. Sports Medicine (Auckland, NZ) 46(2):231–240. https://doi.org/10.1007/s40279-015-0415-7
Selkowitz DM, Beneck GJ, Powers CM (2013) Which exercises target the gluteal muscles while minimizing activation of the tensor fascia lata? Electromyographic assessment using fine-wire electrodes. J Orthop Sports Phys Ther 43(2):54–64. https://doi.org/10.2519/jospt.2013.4116
Smith CE, Hannon JC, McGladrey B, Shultz B, Eisenman P, Lyons B (2014) The effects of a postactivation potentiation warm-up on subsequent sprint performance. Human Mov. https://doi.org/10.2478/humo-2013-0050
Thorborg K, Bandholm T, Zebis M, Andersen LL, Jensen J, Hölmich P (2016) Large strengthening effect of a hip-flexor training programme: a randomized controlled trial. Knee Surg, Sports Traumatol, Arthrosc: off J ESSKA 24(7):2346–2352. https://doi.org/10.1007/s00167-015-3583-y
Walker J, Bissas A, Paradisis GP, Hanley B, Tucker CB, Jongerius N, Thomas A, von Lieres Und Wilkau HC, Brazil A, Wood MA, Merlino S, Vazel P-J, Bezodis IN (2021) Kinematic factors associated with start performance in World-class male sprinters. J Biomech 124:110554. https://doi.org/10.1016/j.jbiomech.2021.110554
Ward SR, Winters TM, Blemker SS (2010) The architectural design of the gluteal muscle group: implications for movement and rehabilitation. J Orthop Sports Phys Ther 40(2):95–102. https://doi.org/10.2519/jospt.2010.3302
Widler KS, Glatthorn JF, Bizzini M, Impellizzeri FM, Munzinger U, Leunig M, Maffiuletti NA (2009) Assessment of hip abductor muscle strength A validity and reliability study. J Bone Joint Surg 91(11):2666–2672. https://doi.org/10.2106/JBJS.H.01119
Wild JJ, Bezodis IN, North JS, Bezodis NE (2022) Characterising initial sprint acceleration strategies using a whole-body kinematics approach. J Sports Sci 40(2):203–214. https://doi.org/10.1080/02640414.2021.1985759
Willcox EL, Burden AM (2013) The influence of varying hip angle and pelvis position on muscle recruitment patterns of the hip abductor muscles during the clam exercise. J Orthop Sports Phys Ther 43(5):325–331. https://doi.org/10.2519/jospt.2013.4004
Williams MJ, Gibson NV, Sorbie GG, Ugbolue UC, Brouner J, Easton C (2021) Activation of the gluteus maximus during performance of the Back squat, split squat, and barbell hip thrust and the relationship with maximal sprinting. J Strength Cond Res 35(1):16–24. https://doi.org/10.1519/JSC.0000000000002651
Wilson JM, Duncan NM, Marin PJ, Brown LE, Loenneke JP, Wilson SMC, Jo E, Lowery RP, Ugrinowitsch C (2013) Meta-analysis of postactivation potentiation and power: effects of conditioning activity, volume, gender, rest periods, and training status. J Strength Cond Res 27(3):854–859. https://doi.org/10.1519/JSC.0b013e31825c2bdb
Worrell TW, Karst G, Adamczyk D, Moore R, Stanley C, Steimel B, Steimel S (2001) Influence of joint position on electromyographic and torque generation during maximal voluntary isometric contractions of the hamstrings and gluteus maximus muscles. J Orthop Sports Phys Ther 31(12):730–740. https://doi.org/10.2519/jospt.2001.31.12.730
Acknowledgements
We would like to thank all participants who volunteered to participate in this study and demonstrated great motivation and commitment. We would like to express our appreciation to the Institute of Movement and Neurosciences of the German Sport University Cologne with special thanks to Dr. Axel J. Knicker.
Funding
Open Access funding enabled and organized by Projekt DEAL. No funding was received to assist with the preparation of this manuscript.
Author information
Authors and Affiliations
Contributions
The original idea for this study was initiated by TA. MG and TA advanced the idea and started the conception. All authors were included in the design of the study. MG was responsible for the acquisition and analysis of data. MG and TA interpreted the data. MG wrote the manuscript, TA and OJQ revised it critically for important intellectual content. All authors were included in the final approval of the version to be submitted. All authors agree to be accountable for all aspects of the work in ensuring that questions related to the accuracy or integrity of any part of the work are appropriately investigated and resolved.
Corresponding author
Ethics declarations
Conflict of interest
The authors have no relevant financial or non-financial interests to disclose.
Ethical approval
All participants were informed about the aims of the study, the procedure of electromyography (EMG) and provided written consent to their voluntary participation and the publication of their data. The investigation was approved by the local ethics commission of the German Sport University Cologne (No. 168/2021) and met all requirements of the Declaration of Helsinki.
Additional information
Communicated by Toshio Moritani.
Publisher's Note
Springer Nature remains neutral with regard to jurisdictional claims in published maps and institutional affiliations.
Supplementary Information
Below is the link to the electronic supplementary material.
Rights and permissions
Open Access This article is licensed under a Creative Commons Attribution 4.0 International License, which permits use, sharing, adaptation, distribution and reproduction in any medium or format, as long as you give appropriate credit to the original author(s) and the source, provide a link to the Creative Commons licence, and indicate if changes were made. The images or other third party material in this article are included in the article's Creative Commons licence, unless indicated otherwise in a credit line to the material. If material is not included in the article's Creative Commons licence and your intended use is not permitted by statutory regulation or exceeds the permitted use, you will need to obtain permission directly from the copyright holder. To view a copy of this licence, visit http://creativecommons.org/licenses/by/4.0/.
About this article
Cite this article
Goller, M., Quittmann, O.J. & Alt, T. How to activate the glutes best? Peak muscle activity of acceleration-specific pre-activation and traditional strength training exercises. Eur J Appl Physiol 124, 1757–1769 (2024). https://doi.org/10.1007/s00421-023-05400-3
Received:
Accepted:
Published:
Issue Date:
DOI: https://doi.org/10.1007/s00421-023-05400-3