Abstract
Objective
A practical screening tool for chronic mesenteric ischemia (CMI) could facilitate early recognition and reduce undertreatment and diagnostic delay. This study explored the ability to discriminate CMI from non-CMI patients with a mesenteric artery calcium score (MACS).
Methods
This retrospective study included CTAs of consecutive patients with suspected CMI in a tertiary referral center between April 2016 and October 2019. A custom-built software module, using the Agatston definition, was developed and used to calculate the MACS for the celiac artery (CA), superior mesenteric artery (SMA), and inferior mesenteric artery. Scoring was performed by two blinded observers. Interobserver agreement was determined using 39 CTAs scored independently by both observers. CMI was defined as sustained symptom improvement after treatment. Non-CMI patients were patients not diagnosed with CMI after a diagnostic workup and patients not responding to treatment.
Results
The MACS was obtained in 184 patients, 49 CMI and 135 non-CMI. Interobserver agreement was excellent (intraclass correlation coefficient 0.910). The MACS of all mesenteric arteries was significantly higher in CMI patients than in non-CMI patients. ROC analysis of the combined MACS of CA + SMA showed an acceptable AUC (0.767), high sensitivity (87.8%), and high NPV (92.1%), when using a ≥ 29.7 CA + SMA MACS cutoff. Comparison of two CTAs, obtained in the same patient at different points in time with different scan and reconstruction parameters, was performed in 29 patients and revealed significant differences in MACSs.
Conclusion
MACS seems a promising screening method for CMI, but correction for scan and reconstruction parameters is warranted.
Key Points
• A mesenteric artery calcium score obtained in celiac artery and superior mesenteric artery has a high negative predictive value for chronic mesenteric ischemia and could serve as a screening tool.
• Interobserver agreement of the mesenteric artery calcium score is excellent.
• Scan and reconstruction parameters influence the mesenteric artery calcium score and warrant the development of a method to correct for these parameters.
Similar content being viewed by others
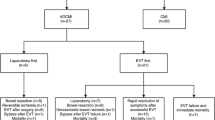
Avoid common mistakes on your manuscript.
Introduction
Postprandial abdominal pain and weight loss caused by fear of eating are incapacitating complaints associated with chronic mesenteric ischemia (CMI) [1, 2]. When left untreated, CMI has been reported to progress to acute mesenteric ischemia in 26–67% of patients [3, 4]. A recent study reported an overall annual CMI incidence of 9.2 per 100,000 inhabitants [5]. As previously shown, this study also reported atherosclerotic CMI (7.3 per 100,000) to be the greatest contributor to the overall incidence [5,6,7]. The incidence of atherosclerotic CMI showed a rising trend over the study period, suggesting that atherosclerotic CMI is an upcoming health issue. European experts agree that CMI still remains an underdiagnosed and undertreated disease, which is mainly caused by a lack of knowledge and awareness among physicians [8]. This observation is supported by a substantial diagnostic delay of approximately 6 months after onset of complaints [5, 9].
An easy to use non-invasive screening tool for CMI could facilitate early recognition and increase awareness for CMI, reducing undertreatment, diagnostic delay, and progression to acute mesenteric ischemia. The Agatston coronary artery calcium score (CACS) has been used to assess the risk of coronary artery disease and aid in clinical decision-making [10, 11]. A CACS of 0 has been shown to have a very high negative predictive value (NPV) of coronary artery disease [12]. A mesenteric artery calcium score (MACS) based on the same principles as the CACS could serve as a potential first test to identify patients in whom CMI is unlikely and identify patients in whom a further diagnostic workup is warranted. This study aimed to explore the ability to discriminate CMI patients from patients without CMI by using a MACS.
Methods
Study design
This single-center retrospective cohort study included consecutive patients analyzed for suspected CMI in a specialized tertiary referral center between April 2016 and October 2019. Patients were eligible for inclusion when a computed tomography (CT)—not older than 12 months before first presentation—was available. Patients were excluded when they had undergone a previous mesenteric artery revascularization, when an anatomical variation with a common origin of the celiac artery (CA) and superior mesenteric artery (SMA) was present, or when the duration of clinical follow-up after mesenteric artery revascularization was less than 3 months. The local medical research ethics committee decided that the Medical Research Involving Human Subjects Act does not apply to this study (MEC-2018-1414). The investigators complied with the Helsinki declaration on research ethics. The STROBE checklist for cohort studies was used to write this manuscript [13].
Diagnostic workup of chronic mesenteric ischemia
A standardized diagnostic workup was performed in all patients and consisted of an assessment of symptoms, computed tomography angiography (CTA), and when indicated assessment of mucosal ischemia by visible light spectroscopy (VLS) [14]. Results of the workup were discussed by an experienced multidisciplinary expert team consisting of gastroenterologists, interventional radiologists, and vascular surgeons. Patients were treated when a consensus diagnosis of CMI was established, which was based on presenting symptoms, imaging, VLS, and absence of a possible alternative diagnosis [15]. A definitive diagnosis of CMI was established when symptoms improved or resolved at three months after revascularization, or in case of chronic non-occlusive mesenteric ischemia during treatment with a vasodilator. Patients with a consensus diagnosis of no CMI or those initially labeled as CMI, but without improvement of symptoms after treatment, were classified as non-CMI. A definitive diagnosis of CMI was used for the primary outcome, which was to determine the ability of the MACS to discriminate patients with a definitive diagnosis of CMI from non-CMI patients.
Calcium scoring tool
The majority of the available CTs were contrast enhanced, which is explained by the fact that a CTA is indicated in the workup of CMI in order to assess vessel patency. When multiple series with different contrast enhancement phases were available, the series with the lowest slice thickness, generally the arterial contrast enhancement series, was used to calculate the MACS. Available semi-automated calcium scoring software can not differ calcified plaques from contrast; i.e., contrast is labeled as calcium, and therefore not suitable for use within this study. A custom-built software module in MeVisLab version 2.7.1 (MeVis Medical Solutions AG) was developed to identify calcified lesions by assigning hand drawn regions of interest on CTA (Fig. 1). The MACS was calculated according to the Agatston definition, which is calculated by multiplying the volume of a lesion with a density factor [10]. To assess the technical accuracy of the software, the CACS of six cardiac CTs without contrast enhancement analyzed by both Syngo.via (Siemens Healthcare) and by MeVisLab were compared.
Mesenteric artery calcium score
The MACS was obtained for all three mesenteric arteries individually; the total MACS was the sum of the MACS of the CA, SMA, and inferior mesenteric artery (IMA). Mesenteric artery stenoses most frequently occur at the origin of a mesenteric artery and involve the aorta wall directly surrounding the origin; distal stenoses are less common [16, 17]. Assumptions were made to standardize the areas of calcium scoring. The estimated volume of a calcified lesion causing a stenosis at the vessels’ origin consisted of the volume of the lesion that was located within a circle with a radius of 1 time the diameter of the vessels’ origin (Fig. 2). Calcium scoring was performed from origin until bifurcation for CA, from origin until the first large jejunal artery for SMA, and from origin until left colic artery for IMA.
Calcium scoring was performed by two observers, an interventional radiologist with 9 years of experience and a trainee with little experience. Both observers were blinded to patient history and clinical outcome. The first 9 CTAs served as a training set and were reviewed by both observers; scoring was performed based on consensus. The following 39 CTAs were used to determine interobserver agreement and scored independently by both observers, while remaining blinded to each other’s results. A sample size of 13 CTAs would suffice for assessment of the intraclass correlation coefficient (ICC), when comparing results of two observers, while using an alpha of 0.05 and a beta of 0.1 [18]. However, an atherosclerotic stenosis is observed in approximately 43–53% of patients [5, 7]. The required sample size of 13 CTAs was tripled to 39 CTAs in order to include sufficient CTAs with calcified lesions, since interobserver variability is expected to be greater when scoring CTAs with calcifications. Interobserver agreement was assessed directly upon completion of the 39 CTAs. When the interobserver agreement was good (ICC 0.75 to 0.9) or excellent (ICC ≥ 0.9), the remaining CTAs would be scored by either one of the observers [19]. When the interobserver agreement was poor (ICC < 0.5) or moderate (ICC 0.5 to 0.75), the remaining CTAs would be scored by both observers.
Variability scan protocols
The vast majority of patients were referred by other hospitals and CTA imaging had often been performed in the referring hospital, causing a large variety in the used scan protocols. A CTA, performed using a standardized mesenteric artery protocol, was repeated during the workup when the image quality was deemed insufficient to reliably assess mesenteric artery patency. When two scans of the same patient were available calcium scoring was performed on both scans. Both scans had to be performed within 12 months before presentation, since clinically significant progression of atherosclerosis was expected to be unlikely within this time window. The scan performed in the tertiary referral center was included in the analysis of the primary endpoint. A separate analysis was performed to determine whether imaging and reconstruction parameters influenced the MACS by comparing the MACS and CTA characteristics—i.e., slice thickness, pixel spacing, tube voltage, and contrast enhancement—of the two scans. The density of contrast enhancement was calculated by the sum of the mean and two times the standard deviation (sd) of the Hounsfield unit (HU) value measured within the center of the aorta at the level of the origin of the mesenteric artery.
Data collection and statistical analysis
Data regarding medical history, presenting symptoms, presence of a mesenteric artery stenosis (defined as ≥ 50% luminal reduction), diagnosis, follow-up, CTA characteristics, density of contrast enhancement, and MACS were collected and pseudo anonymized with a unique code.
Statistical analyses were performed with R version 3.5.3 (R Foundation for Statistical Computing) using packages pROC and irr. Continuous variables were not equally distributed and, therefore, shown as median and interquartile range (IQR). Baseline characteristics of CMI and non-CMI patients were compared using chi-square or Fisher’s exact testing for categorical variables and Wilcoxon rank testing for continuous variables. The ICC was used to assess MACS interobserver agreement and correlation between the CACS calculated by Syngo.via and MeVisLab. Receiver operator characteristics curves were computed to define MACS cutoff values to discriminate CMI patients from non-CMI patients. The area under the curve (AUC) was used to quantify the discriminative ability of the MACS. An AUC of 0.5 suggests no discrimination; AUC of 0.7 to 0.8 acceptable discrimination; AUC of 0.8 to 0.9 excellent discrimination; and AUC ≥ 0.9 outstanding discrimination [20]. The sign test was used to compare the characteristics and MACS of two CTAs of the same patient (obtained at two different points in time).
Results
During the study period, 203 patients were analyzed for suspected CMI. A total of 184 patients was included in the study. Reasons for exclusion were a previous mesenteric artery revascularization in 9 patients, a common origin of CA and SMA in 2 patients, and a follow-up duration of < 3 months after revascularization in 8 patients. A definitive diagnosis of CMI was established in 49 of the included patients, of whom 46 were diagnosed with atherosclerotic CMI, 2 with median arcuate ligament syndrome (MALS), and 1 with chronic non-occlusive mesenteric ischemia. The remaining 135 patients were classified as non-CMI.
Baseline and CTA characteristics
CMI patients were significantly older (CMI 70 (65–75) vs. non-CMI 64 (56–70)) and significantly more often female (CMI 81.6% vs. non-CMI 65.9%). Comparison of risk factors of cardiovascular disease (CVD) showed that a history of CVD, a history of peripheral artery disease, and smoking were significantly more prevalent among CMI patients (Table 1). The presenting symptoms of weight loss, postprandial abdominal pain, diarrhea, and an adapted eating pattern were also significantly more prevalent in CMI patients.
CTA revealed no mesenteric artery stenosis in 56 (30.4%) patients, CA stenosis in 45 (24.5%) patients, SMA stenosis in 17 (9.2%) patients, IMA stenosis in 10 (5.4%) patients, CA and SMA stenosis in 18 (9.8%) patients, CA and IMA stenosis in 10 (5.4%) patients, SMA and IMA stenosis in 10 (5.4%) patients, and CA, SMA, and IMA stenosis in 18 (9.8%) patients (Table 2). The nature of the stenosis was atherosclerosis in 77.1% of patients, compression in 19.1%, iatrogenic in 2.3%, and a combination of atherosclerosis and compression in 1.5% of patients (Table 2). Comparison of the CTA characteristics of CMI and non-CMI patients did not show a significant difference in tube voltage, slice thickness, pixel spacing, or contrast density at the level of CA, SMA, or IMA.
Calcium scoring software accuracy and interobserver agreement
The CACS on the cardiac CTs, calculated by Syngo.via and MeVisLab, showed an excellent correlation with an ICC of 0.998 (95% confidence interval (CI) 0.991–1.000). Proving technical accuracy after which the assessment of the MACS was commenced. MACS of the 39 CTAs scored by both observers showed an excellent correlation as well (ICC 0.910 (95% CI 0.834–0.951)). Hence, the remaining CTAs were scored by either one of the observers.
Mesenteric artery calcium score
The MACSs of CA, SMA, IMA, CA + SMA, and the total MACS were all significantly higher in CMI patients than in non-CMI patients (Table 3). The ability to discriminate CMI patients from non-CMI patients using the MACS was assessed by calculating the AUC. MACSs of CA + SMA and total MACS showed the highest discriminative ability with acceptable AUCs of 0.767 (95% CI 0.686–0.847) and 0.770 (95% CI 0.690–0.851) respectively (Table 4). The best test characteristics for the identification of CMI patients were found when using a MACS cutoff of ≥ 29.7 for CA + SMA: sensitivity 87.8%, specificity 51.9%, positive predictive value (PPV) 39.8%, and negative predictive value (NPV) 92.1%. Seventy of the 184 (38%) patients had a MACS < 29.7 and no definitive diagnosis of CMI.
Influence scan protocol on calcium score
A scan performed in both the tertiary referral center and the referring hospital was available for 29 patients. Comparison of the CTAs showed a significant difference in the used tube voltage and the contrast densities of the CTAs (Table 5). The slice thickness of the tertiary referral center CTAs was significantly thinner than the slice thickness of the CTAs of the referring hospital (tertiary referral center 0.8 (0.8–0.9) vs. referring hospital 3.0 (2.0–3.0)). When comparing the MACS of both CTAs, the MACS of SMA, CA + SMA, and the total MACS were all significantly higher when calculated on the CTA of the referring hospital.
Discussion
This study is the first to report the use of a MACS to discriminate CMI patients from non-CMI patients. A combined MACS of CA + SMA showed most promising for this purpose with an AUC of 0.767 and a high NPV (92.1%), using a ≥ 29.7 cutoff for CA + SMA. Interobserver agreement of the MACS was excellent (ICC 0.910). The used scan protocol and reconstruction parameters (slice thickness, tube voltage, and contrast enhancement) influenced the calculated MACS.
Limited awareness for CMI and lack of knowledge among physicians cause diagnostic delays. An easy to use screening tool for CMI could shorten this diagnostic delay, but is unavailable. The CA + SMA calcium score seems able to discriminate CMI patients from non-CMI patients. The discriminative ability was acceptable (AUC 0.767) and the sensitivity (87.8%) and NPV (92.1%) were high. Even though the MACS was calculated on CTA and reconstruction parameters varied between scans, the MACS still approximates the test characteristics of a CACS of 0 (sensitivity 99–100%, NPV 97–100%), indicating that the CA + SMA MACS is a promising screening method [12]. A second reason to opt for use of the CA + SMA MACS is the good recognizability of the origin of the CA and SMA. While the identification of the origin of the IMA can be more cumbersome, especially on non-contrast-enhanced CT, and the addition of the IMA to the MACS seems of limited value. Calculation of the CA + SMA MACS on CTs of patients with weight loss or postprandial abdominal pain in whom the diagnosis CMI is considered and, in whom an alternative diagnosis is not found, is an attractive clinical application of this score. A low calcium score effectively rules out CMI, implying that a CTA would not be indicated in approximately 38% of patients, which is advantageous to patients with impaired renal function. CMI is more probable in case of an increased CA + SMA MACS, warranting a diagnostic workup, including a CTA with ≤ 1-mm slice thickness to assess vessel patency [8].
The currently used software enabled calcium scoring on contrast-enhanced CT. The interobserver agreement was excellent (ICC 0.910), even though regions of interest were drawn by hand and were performed by an experienced interventional radiologist and a trainee, suggesting that the MACS on CTA does not depend on the scoring radiologist. The 39 CTs scored by both observers included too few non-contrast-enhanced CTs to reliably assess the effect of contrast enhancement on the interobserver agreement of the MACS. Observers could have falsely included contrast at the borders of the lesion and could have missed calcifications with a low intensity that were concealed by high intensity of contrast enhancement, which influences reliability of the MACS. The current necessity to draw regions of interest by hand is time consuming, especially when the slice thickness is ≤ 1 mm. Development of (semi-)automated calcium scoring software, such as currently used for the CACS, should be validated for several levels of intensity of contrast enhancement in order to solve the limitation of contrast enhancement and make mesenteric artery calcium scoring fast and feasible for clinical practice.
This retrospective study is a first attempt to identify CMI patients using a MACS and should be considered a proof of principle. The high NPV indicates that the MACS could potentially be a much desired screening tool for CMI. However, suggesting a suitable cutoff for clinical use is premature due to limitations of the current study, such as the large variation in CTA characteristics of the included scans, which influenced the MACS when comparing two CTAs performed in one patient. The influence of tube voltage, slice thickness, and reconstruction kernel on the calcium score has already been described in CACS literature [21,22,23]. The influence of tube voltage on the CACS has recently been studied by Booij et al; they showed that CACSs on a phantom significantly differed when tube voltage increased [21]. Qian et al compared the volume of coronary calcium on 0.5-mm and 3.0-mm slice thickness reconstructions, using intravascular ultrasound with radiofrequency backscatter-virtual histology as a reference [22]. Reconstructions of 0.5-mm reduced the overestimation of calcium volume as compared to conventional 3.0-mm reconstructions and had a higher sensitivity (0.5-mm 94%, 3.0 mm-56%), but lower specificity (0.5-mm 50%, 3.0-mm 93%). Mantini et al reported that the CACS on 0.5-mm slice thickness reconstructions resulted in significant reclassification of the cardiovascular risk when compared with standard 3.0-mm reconstructions [23]. The use of reconstructions with a different reconstruction kernel also resulted in significant reclassification of the cardiovascular risk, showing that variation in the used reconstruction parameters can influence clinical decision-making. The results of these studies support the observed influence of scan and reconstruction parameters on the MACS, underlining that correction factors for slice thickness, tube voltage, and contrast enhancement are needed to set a reliable MACS cutoff that is feasible for use in clinical practice. Correction factors could be calculated by a phantom study using different slice thicknesses, tube voltages, and intensities of contrast enhancement.
Other possible limitations of this study are related to the included patient population and retrospective study design. First, all included patients were referred because of suspected CMI based on typical symptoms and/or mesenteric artery stenosis, resulting in a population with a higher pretest probability of CMI. However, the prevalence of CVD risk factors and typical symptoms still differed significantly between CMI and non-CMI patients, suggesting that the predictive value of MACS could be improved by relating the MACS to history and clinical symptoms. Hence, the addition of the MACS to an existing CMI score chart could improve the accuracy of the predicted CMI probability and possibly prevent a missed diagnosis of CMI in patients with a non-calcified mesenteric artery stenosis [7]. Second, our inclusion strategy resulted in the inclusion of two MALS patients. These patients had a MACS of 0, since MALS is caused by CA compression and not by atherosclerosis. MALS is typically seen in patients of younger age, without cardiovascular risk factors [2, 24]. Non-atherosclerotic causes of CMI, such as MALS or vasculitis, are more probable in patients of younger age. Selection of patients based on age, e.g., ≥ 40 years of age, could be a viable strategy to improve the sensitivity of the MACS and avoid false-negative tests. Third, the follow-up duration of non-CMI patients was shorter than the follow-up duration of CMI patients. Non-CMI patients could have been treated in another center after their last outpatient clinic visit, although this is unlikely, since there is only one other mesenteric ischemia expert center in the country. Fourth, the number of included patients was too low to allow subgroup analysis, e.g., to determine MACS cutoffs for each level of tube voltage.
This proof of principle study demonstrates the ability to discriminate CMI patients from non-CMI patients using the MACS (AUC 0.767). The MACS could be a promising screening tool for CMI with a high sensitivity (87.8%) and high NPV (92.0%), despite the mentioned limitations. These results suggest that a low MACS rules out CMI and an increased MACS warrants and justifies further diagnostic workup with a ≤ 1-mm CTA [8]. Correction for scan and reconstruction parameters is needed, since we observed significant differences in MACSs scored on two CTAs of the same patient acquired with a different scan and/or reconstruction protocol. Implementation and validation of an algorithm to correct for scan and reconstruction parameters seem to be the next steps to further evaluate the clinical promise and applicability of this tool.
Abbreviations
- AUC:
-
Area under the curve
- CA:
-
Celiac artery
- CACS:
-
Coronary artery calcium score
- CI:
-
Confidence interval
- CMI:
-
Chronic mesenteric ischemia
- CT:
-
Computed tomography
- CTA:
-
Computed tomography angiography
- CVD:
-
Cardiovascular disease
- HU:
-
Hounsfield unit
- ICC:
-
Intraclass correlation coefficient
- IMA:
-
Inferior mesenteric artery
- IQR:
-
Interquartile range
- kVp:
-
Kilovoltage peak
- MACS:
-
Mesenteric artery calcium score
- MALS:
-
Median arcuate ligament syndrome
- NPV:
-
Negative predictive value
- PPV:
-
Positive predictive value
- Sd:
-
Standard deviation
- SMA:
-
Superior mesenteric artery
- VLS:
-
Visible light spectroscopy
References
Alahdab F, Arwani R, Pasha AK et al (2018) A systematic review and meta-analysis of endovascular versus open surgical revascularization for chronic mesenteric ischemia. J Vasc Surg 67:1598–1605
Mensink PB, Moons LM, Kuipers EJ (2011) Chronic gastrointestinal ischaemia: shifting paradigms. Gut 60:722–737
Kolkman JJ, Mensink PB, van Petersen AS, Huisman AB, Geelkerken RH (2004) Clinical approach to chronic gastrointestinal ischaemia: from ‘intestinal angina’ to the spectrum of chronic splanchnic disease. Scand J Gastroenterol Suppl:9–16
Thomas JH, Blake K, Pierce GE, Hermreck AS, Seigel E (1998) The clinical course of asymptomatic mesenteric arterial stenosis. J Vasc Surg 27:840–844
Terlouw LG, Verbeten M, van Noord D et al (2020) The incidence of chronic mesenteric ischemia in the well-defined region of a Dutch Mesenteric Ischemia Expert Center. Clin Transl Gastroenterol 11:e00200
Harki J, Vergouwe Y, Spoor JA et al (2017) Diagnostic accuracy of the combination of clinical symptoms and CT or MR angiography in patients with chronic gastrointestinal ischemia. J Clin Gastroenterol 51:e39–e47
van Dijk LJ, van Noord D, Geelkerken RH et al (2019) Validation of a score chart to predict the risk of chronic mesenteric ischemia and development of an updated score chart. United European Gastroenterol J 7:1261–1270
Terlouw LG, Moelker A, Abrahamsen J et al (2020) European guidelines on chronic mesenteric ischaemia - joint United European Gastroenterology, European Association for Gastroenterology, Endoscopy and Nutrition, European Society of Gastrointestinal and Abdominal Radiology, Netherlands Association of Hepatogastroenterologists, Hellenic Society of Gastroenterology, Cardiovascular and Interventional Radiological Society of Europe, and Dutch Mesenteric Ischemia Study group clinical guidelines on the diagnosis and treatment of patients with chronic mesenteric ischaemia. United European Gastroenterol J 8:371–395
ter Steege RW, Sloterdijk HS, Geelkerken RH, Huisman AB, van der Palen J, Kolkman JJ (2012) Splanchnic artery stenosis and abdominal complaints: clinical history is of limited value in detection of gastrointestinal ischemia. World J Surg 36:793–799
Agatston AS, Janowitz WR, Hildner FJ, Zusmer NR, Viamonte M Jr, Detrano R (1990) Quantification of coronary artery calcium using ultrafast computed tomography. J Am Coll Cardiol 15:827–832
Arnett DK, Blumenthal RS, Albert MA et al (2019) 2019 ACC/AHA guideline on the primary prevention of cardiovascular disease: a report of the American College of Cardiology/American Heart Association Task Force on Clinical Practice Guidelines. Circulation 140:e596–e646
Haberl R, Becker A, Leber A et al (2001) Correlation of coronary calcification and angiographically documented stenoses in patients with suspected coronary artery disease: results of 1,764 patients. J Am Coll Cardiol 37:451–457
von Elm E, Altman DG, Egger M et al (2007) The Strengthening the Reporting of Observational Studies in Epidemiology (STROBE) statement: guidelines for reporting observational studies. Lancet 370:1453–1457
Van Noord D, Sana A, Benaron DA et al (2011) Endoscopic visible light spectroscopy: a new, minimally invasive technique to diagnose chronic GI ischemia. Gastrointest Endosc 73:291–298
van Dijk LJ, van Noord D, de Vries AC et al (2019) Clinical management of chronic mesenteric ischemia. United European Gastroenterol J 7:179–188
Landis MS, Rajan DK, Simons ME, Hayeems EB, Kachura JR, Sniderman KW (2005) Percutaneous management of chronic mesenteric ischemia: outcomes after intervention. J Vasc Interv Radiol 16:1319–1325
Sheeran SR, Murphy TP, Khwaja A, Sussman SK, Hallisey MJ (1999) Stent placement for treatment of mesenteric artery stenoses or occlusions. J Vasc Interv Radiol 10:861–867
Bujang MA, Baharum N (2017) A simplified guide to determination of sample size requirements for estimating the value of intraclass correlation coefficient: a review. Arch Orofac Sci 12
Koo TK, Li MY (2017) A Guideline of Selecting and Reporting Intraclass Correlation Coefficients for Reliability Research. J Chiropr Med 15(2):155–163
Hosmer DWLS, Sturdivant RX (2000) Applied logistic regression, 2nd edn. John Wiley & Sons, New York, NY
Booij R, van der Werf NR, Budde RPJ, Bos D, van Straten M (2020) Dose reduction for CT coronary calcium scoring with a calcium-aware image reconstruction technique: a phantom study. Eur Radiol 30(6):3346–3355
Qian Z, Dhungel A, Vazquez G, Weeks M, Voros S, Rinehart S (2015) Coronary artery calcium: 0.5 mm slice-thickness reconstruction with adjusted attenuation threshold outperforms 3.0 mm by validating against spatially registered intravascular ultrasound with radiofrequency backscatter. Acad Radiol 22:1128–1137
Mantini C, Maffei E, Toia P et al (2018) Influence of image reconstruction parameters on cardiovascular risk reclassification by Computed Tomography Coronary Artery Calcium Score. Eur J Radiol 101:1–7
Kim EN, Lamb K, Relles D, Moudgill N, DiMuzio PJ, Eisenberg JA (2016) Median arcuate ligament syndrome-review of this rare disease. JAMA Surg 151:471–477
Funding
The authors state that this work has not received any funding.
Author information
Authors and Affiliations
Corresponding author
Ethics declarations
Guarantor
The scientific guarantor of this publication is Dr. A. Moelker.
Conflict of interest
The authors of this manuscript declare relationships with the following companies: AM is proctor for Terumo, Cook, and Angiocare. MB is consultant to Boston Scientific and Cook Medical and has received grants for investigator initiated and industry sponsored studies from Boston Scientific, Cook Medical, Pentax Medical, 3M, and Mylan. LT, DvN, and TvW declare no relationships with any companies, whose products or services may be related to the subject matter of the article.
Statistics and biometry
No complex statistical methods were necessary for this paper.
Informed consent
Written informed consent was not required for this study because the local medical research ethics committee decided that the Medical Research Involving Human Subjects Act does not apply to this study (MEC-2018-1414).
Ethical approval
Institutional Review Board approval was obtained.
Methodology
• retrospective
• diagnostic or prognostic study
• performed at one institution
Additional information
Publisher’s note
Springer Nature remains neutral with regard to jurisdictional claims in published maps and institutional affiliations.
Rights and permissions
Open Access This article is licensed under a Creative Commons Attribution 4.0 International License, which permits use, sharing, adaptation, distribution and reproduction in any medium or format, as long as you give appropriate credit to the original author(s) and the source, provide a link to the Creative Commons licence, and indicate if changes were made. The images or other third party material in this article are included in the article's Creative Commons licence, unless indicated otherwise in a credit line to the material. If material is not included in the article's Creative Commons licence and your intended use is not permitted by statutory regulation or exceeds the permitted use, you will need to obtain permission directly from the copyright holder. To view a copy of this licence, visit http://creativecommons.org/licenses/by/4.0/.
About this article
Cite this article
Terlouw, L.G., van Noord, D., van Walsum, T. et al. Mesenteric artery calcium scoring: a potential screening method for chronic mesenteric ischemia. Eur Radiol 31, 4212–4220 (2021). https://doi.org/10.1007/s00330-020-07530-0
Received:
Revised:
Accepted:
Published:
Issue Date:
DOI: https://doi.org/10.1007/s00330-020-07530-0