Abstract
Using comparative modeling, we have generated structural models of 475 α and β tubulins. Using these models, we observed a global, structural similarity between the tubulin isotypes. However, a number of subtle differences in the isotypes physical properties, including net electric charges, solvent accessible surface areas, and electric dipole moments were also apparent. In order to examine the roles that these properties may play in microtubule (MT) assembly and stability, we have created a model to evaluate the dipole–dipole interaction energies of varying MT lattice conformations, using human tubulin isotypes as particularly important examples. We conclude that the dipole moments of each tubulin isotype may influence their functional characteristics within the cell, resulting in differences for MT assembly kinetics and stability.





Similar content being viewed by others
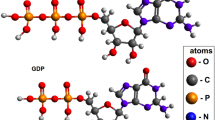
References
Aldaz H et al (2005) Insights into microtubule nucleation from the crystal structure of human gamma-tubulin. Nature 435:523–527
Baker N et al (2001) Electrostatics of nanosystems: application to microtubules and the ribosome. Proc Natl Acad Sci USA 98:10037–10041
Berman HM et al (2000) The protein data bank. Nucleic Acids Res 28:235–242
Boeckmann B et al (2003) The SWISS-PROT protein knowledgebase and its supplement TrEMBL in 2003. Nucleic Acids Res 31:365–370
Chothia C, Lesk A (1986) The relation between the divergence of sequence and structure in proteins. EMBO J 5:823–826
Connolly M (1983) Solvent-accessible surfaces of proteins and nucleic acids. Science 221:709–713
Gigant B et al (2000) The 4 A X-ray structure of a tubulin:stathmin-like domain complex. Cell 102:809–816
Gigant B et al (2005) Structural basis for the regulation of tubulin by vinblastine. Nature 435:519–522
Higgins D, Sharp P (1988) CLUSTAL: a package for performing multiple sequence alignment on a microcomputer. Gene 73:237–244
Hooft RW et al (1996) Errors in protein structures. Nature 381:272
Hoogland C et al (2004) SWISS-2DPAGE, ten years later. Proteomics 4:2352–2356
Horio T, Hotani H (1986) Visualization of the dynamic instability of individual microtubules by dark-field microscopy. Nature 321:605–607
Hyams JS, Stebbings H (1979) The mechanism of microtubule associated cytoplasmic transport. Isolation and preliminary characterisation of a microtubule transport system. Cell Tissue Res 196:103–116
Inclan Y, Nogales E (2001) Structural models for the self-assembly and microtubule interactions of gamma-, delta- and epsilon-tubulin. J Cell Sci 114:413–422
Jordan M, Wilson L (2004) Mary Ann Jordan, Leslie Wilson. Nat Rev Cancer 4:253–265
Kikkawa M et al (2001) Switch-based mechanism of kinesin motors. Nature 411:439–445
Kirschner M, Schulze E (1986) Morphogenesis and the control of microtubule dynamics in cells. J Cell Sci Suppl 5:293–310
Laskowski RA et al (1993) PROCHECK: a program to check the stereochemical quality of protein structures. J Appl Crystallogr 26:283–291
Li H et al (2002) Microtubule structure at 8 A resolution. Structure (Camb) 10:1317–1328
Lindahl E, Hess B, Van Der Spoel D (2001) GROMACS 3.0: a package for molecular simulation and trajectory analysis. J Mol Mod 7:306–317
Lowe J et al (2001) Refined structure of alpha beta-tubulin at 3.5 A resolution. J Mol Biol 313:1045–1057
Lu Q, Luduena R (1994) In vitro analysis of microtubule assembly of isotypically pure tubulin dimers. Intrinsic differences in the assembly properties of alpha beta II, alpha beta III, and alpha beta IV tubulin dimers in the absence of microtubule-associated proteins. J Biol Chem 269:2041–2047
Luduena R (1998) Multiple forms of tubulin: different gene products and covalent modifications. Int Rev Cytol 178:207–275
Mandelkow E, Mandelkow E (1992) Microtubule oscillations. Cell Motil Cytoskeleton 22:235–244
Margolis R, Wilson L (1981) Microtubule treadmills—possible molecular machinery. Nature 293:705–711
Melki R et al (1989) Cold depolymerization of microtubules to double rings: geometric stabilization of assemblies. Biochemistry 28:9143–9152
Minoura I, Muto E (2006) Dielectric measurements of individual microtubles using the electroorientation method. Biophys J 90:3739–3748
Mitchison T, Kirschner M (1984) Dynamic instability of microtubule growth. Nature 312:237–242
Nettles J et al (2004) The binding mode of epothilone A on alpha, beta-tubulin by electron crystallography. Science 305:866–869
Nogales E, Wolf S, Downing K (1998) Structure of the alpha beta tubulin dimer by electron crystallography. Nature 391:199–203
Oakley B et al (1990) Gamma-tubulin is a component of the spindle pole body that is essential for microtubule function in Aspergillus nidulans. Cell 61:1289–1301
Panda D et al (1994) Microtubule dynamics in vitro are regulated by the tubulin isotype composition. Proc Natl Acad Sci USA 91:11358–11362
Pichichero M, Avers C (1973) The evolution of cellular movement in eukaryotes: the role of microfilaments and microtubules. Subcell Biochem 2:97–105
Ravelli R et al (2004) Insight into tubulin regulation from a complex with colchicine and a stathmin-like domain. Nature 428:198–202
Roach MC et al (1998) Preparation of a monoclonal antibody specific for the class I isotype of beta-tubulin: the beta isotypes of tubulin differ in their cellular distributions within human tissues. Cell Motil Cytoskeleton 39:273–285
Sanchez R, Sali A (2000) Comparative protein structure modeling. Introduction and practical examples with modeller. Methods Mol Biol 143:97–129
Schlieper D et al (2005) Structure of bacterial tubulin BtubA/B: evidence for horizontal gene transfer. Proc Natl Acad Sci USA 102:9170–9175
Schoutens JE (2005) Dipole‚ dipole interactions in microtubules. J Biol Phys 31:35–55
Schuessler HA et al (2003) Surface plasmon resonance study of the actin-myosin sarcomeric complex and tubulin dimers. J Mod Optics 50:2381–2391
Sept D, Baker NA, Mccammon J (2003) The physical basis of microtubule structure and stability. Protein Sci 12:2257–2261
Stracke R et al (2002) Analysis of the migration behaviour of single microtubules in electric fields. Biochem Biophys Res Commun 293:602–609
van Gunsteren WF (1996) Biomolecular simulation: the GROMOS96 manual and user guide. Hochschulverlag AG an der ETH Zurich, Zurich
Walker R, Pryer NK, Salmon E (1991) Dilution of individual microtubules observed in real time in vitro: evidence that cap size is small and independent of elongation rate. J Cell Biol 114:73–81
Westermann S et al (2006) The Dam1 kinetochore ring complex moves processively on depolymerizing microtubule ends. Nature 440:565–569
Acknowledgments
This work has been supported by grants from NSERC (Canada), MITACS and grants to R. F. L. from the Welch Foundation (AQ-0726), US Department of Defense BCRP (W81XWH-05-1-0238) and the PCRP (W81XWH-04-1-0231). This work was also supported through a grant from the Canadian Prostate Cancer Research Initiative (016501). Additional support has come from grant P30-CA54174 from the National Institutes of Health to the San Antonio Cancer Institute. Financial support for this project from Technology Innovations, LLC of Rochester, NY, is gratefully acknowledged.
Author information
Authors and Affiliations
Corresponding author
Rights and permissions
About this article
Cite this article
Carpenter, E.J., Huzil, J.T., Ludueña, R.F. et al. Homology modeling of tubulin: influence predictions for microtubule’s biophysical properties. Eur Biophys J 36, 35–43 (2006). https://doi.org/10.1007/s00249-006-0088-0
Received:
Revised:
Accepted:
Published:
Issue Date:
DOI: https://doi.org/10.1007/s00249-006-0088-0