Abstract
Detection of allergens in foods, including soybean, is relevant for food labelling requirements. Moreover, allergen-specific methods may allow standardisation of allergens in food matrices for use in food challenges as allergy diagnostic approaches. Rapid methods are preferred for screening and along the manufacturing line. Previously, we demonstrated sensitive and specific detection of soybean DNA by combining loop-mediated isothermal amplification (LAMP) and lateral flow device (LFD)-like visualisation. However, lengthy DNA extraction and potential contamination of subsequent by previous LAMP reactions from unclosed LFD may impact its use as a rapid and robust method. Here, we developed a rapid protocol for DNA extraction. Moreover, we identified phenol red for distinct visualisation of positive reactions in permanently closed reaction tubes. The optimised method was validated using complex foods (boiled sausage, instant soup, and chocolate) with known amounts of soybean. Further, its applicability was shown in 12 processed retail foods. Results were verified by orthogonal qPCR. The enhanced LAMP method allowed detection at or below 10 mg soybean per kg processed food. The method provides rapid and easy-to-use screening without the need for detection equipment. Hence, it may serve to verify the presence of soybean ingredients and support a risk-based precautionary labelling of non-ingredient soybean in compound foods. Also, as determination of clinical reaction thresholds before and after allergen immunotherapy (AIT) is both inclusion and exclusion criterion for clinical trials and success parameter of AIT, the method may allow verification of calculable soybean content in provocation meals and thus a standardised administration for threshold determination before and after AIT.
Similar content being viewed by others
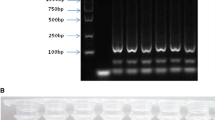
Avoid common mistakes on your manuscript.
Introduction
Allergy to soybean (Glycine max) is common and can lead to adverse and occasionally life-threatening reactions in sensitised subjects [1, 2]. Oral challenges with the culprit food are the definitive clinical diagnostic procedure in verification of a food allergy [3], such as to soybean, and the so-called double-blind placebo-controlled food challenge (DBPCFC) has been considered the “gold standard” to diagnose food allergy [4]. After unequivocal diagnosis of an allergy to soybean, soybean food avoidance remains the most effective method to prevent an allergic reaction because of a perpetual lack of causative immunotherapy of soybean allergy. To support soybean allergic individuals in a safe food choice, soybean-specific detection methods are essential to verify proper labelling of soybean ingredients and to support a risk-based precautionary labelling-approach concerning the presence of unintended and unavoidable non-ingredient allergens (allergenic cross-contact, e.g. during storage, manufacturing or packing) [5]. Moreover, analytical methods specific for the allergenic food can help validate and quality-assure diagnostic food matrices, as used in DBPCFC, which consist of active (contains allergenic food) and placebo (devoid of allergenic food) meals [6,7,8].
The detection of allergenic foods can be done by detecting specific proteins or DNA of the culprit food. The most common methods for direct determination of allergenic proteins are enzyme-linked immunosorbent assays (ELISA) and peptide-based mass spectrometric analysis (MS) [5]. If food allergen identification is aimed on the detection of a characteristic DNA sequence, polymerase chain reaction (PCR) is the method of choice [5]. However, these robust and reliable methods are time-consuming, are partly cost-intensive and require a range of laboratory equipment and qualified personnel. Accordingly, they are only suitable to a limited extent when simple and rapid analysis is required, as for example for the on-site investigation of allergens during food production.
Rapid antibody-based tests such as lateral flow devices (LFDs) have become valuable tools in allergen detection, but issues on specificity are similar to antibody-based ELISA [9,10,11]. Moreover, an undesirable hook effect at high target protein concentrations can potentially occur, leading to a false-negative result despite of a high concentration level of the allergenic food [12, 13]. Rapid and sensitive DNA-based methods have the advantage of high specificity due to higher complexity of the DNA sequence versus protein sequence. Previously, we described a highly sensitive and soybean-specific method targeting the multicopy gene mitochondrial open reading frame 160b (ORF 160b). This loop-mediated isothermal amplification (LAMP) of soybean DNA showed no hook effect of amplification products subsequently detected in LFD [14, 15]. Furthermore, the replacement of antibody-based methods by alternative techniques follows the 3R principle (replace, reduce, refine) to reduce animal experiments [16]. LAMP-based detection offers all the advantages of sensitivity, specificity and 3R-methodology and has become indispensable in clinical diagnostics [17,18,19]. In the field of food allergen analysis, there are few applications to date [15, 20,21,22,23,24,25,26,27,28,29].
The potential use of LAMP as a rapid test for direct on-site allergen screening is accomplished if the total analysis time from sample preparation to target detection is short and the result is rapidly detected, e.g. by the visual appearance of bands in an LFD assay or a colorimetric staining in the reaction tube. In our previously published soybean LAMP-LFD [14, 15], the DNA extraction protocol still comprised elaborate steps: One-hour thermal lysis with cetyltrimethylammonium bromide (CTAB), subsequent purification with chloroform and isopropanol, over-night solubilisation of precipitated DNA, and further DNA purification using a commercially available kit. Hence, the time efficiency of a rapid LAMP detection of soybean allergen was hampered by a time-consuming DNA extraction method. Furthermore, the use of LFD as the detection method requires to open the amplification tubes. Thus, the risk of a potential contamination of the test environment with amplicons, which may lead to false-positive results in subsequent LAMP reactions, cannot be ruled out if the steps of amplification and detection are not spatially separated from DNA extraction and LAMP set-up. Although the separation of crucial steps in DNA amplification is state-of-the art, avoidance of amplicon release is an additional safety measure for reliable and reproducible use of DNA amplification methods.
With this work, we aim to improve LAMP as a rapid and potential on-site method for soybean detection with a significant reduction of the extraction time as well as an optimised detection strategy that avoids the opening of reaction tubes. To address these two challenges, various rapid extraction methods and different detection dyes were investigated for qualitative visual detection in closed reaction tubes. After assessing the different methods in terms of functionality, time and conclusiveness, the best performing method was identified and its performance was validated against food matrices with known amounts of soybean. Consequently, its applicability was shown in retail food samples.
Materials and methods
Food samples
The production of the food matrices, boiled sausage, instant tomato soup, and dark chocolate, all with incurred soybean, and the procurement of the twelve commercial soybean products were previously described [15]. The food matrices were tested soybean negative using a lectin qPCR [30] before fortification at the distinct levels of 100 mg, 101 mg, 102 mg, 103 mg, 104 mg and 105 mg soybean per kg matrix. All food samples were homogenised and stored at − 20 ℃ until testing.
DNA extraction protocols
For comparison with rapid DNA extraction protocols, DNA was purified from food matrices using our in-house elaborate CTAB extraction protocol [14]. Briefly, 100 mg sample was lysed and DNA extracted in 1.4 mL CTAB buffer (55 mM CTAB, 1400 mM NaCl, 20 mM EDTA, 100 mM Tris, pH 8.0) and 20 µL proteinase K at 65 ℃ for 1 h. The supernatant was purified using chloroform, soluble DNA was precipitated using isopropanol and mussel glycogen, and the pellet was washed with 70% ethanol and dried. Resuspension was performed in 100 µL TE buffer (10 mM Tris–HCl, 1 mM EDTA, pH 8.0) overnight followed by purification using the QIAquick PCR Purification kit (Qiagen, Hilden, Germany).
The following rapid methods and commercial kits were tested: 30 s dipstick method using a cellulose-based filter paper [31], EchoLUTION Plant DNA kit (BioEcho Life Science, Köln, Germany), and EchoCLEAN DNA CleanUp kit (BioEcho Life Science) combined with prior extensive CTAB lysis according to Allgöwer et al. [14]. For this purpose, DNA was extracted from the sample with CTAB buffer and proteinase K for 1 h at 65 ℃ and 1000 rpm, followed by centrifugation at 13,200 rpm for 5 min (CTAB lysis according Allgöwer et al.), then the supernatant was used in the EchoCLEAN DNA CleanUp kit. Furthermore, the FastPrep-24 high-speed benchtop homogenizer for rapid lysis (MP Biomedicals, Eschwege, Germany) in combination with the SureFood PREP Advanced kit, protocol 1 (R-Biopharm, Pfungstadt, Germany) was tested according to the details given in the publications and manufacturer’s instructions, respectively. A schematic representation of the different methods can be found in Fig. 1.
Optimised DNA extraction protocol
Homogenised sample material (100 ± 2 mg) was extracted in 580 µL of lysis buffer and 20 µL of proteinase K of the SureFood Prep Advanced kit (R-Biopharm), a lysis matrix bead and approx. 1.5 mg of sea sand using a high-speed benchtop homogeniser (FastPrep-24 and Lysing Matrix A, MP Biomedicals). Lysis took place at a speed of 6.5 m/s for 60 s at ambient temperature. Subsequent purification of the released DNA was performed using SureFood Prep Advanced kit according to protocol 1 following the manufacturer’s instructions (R-Biopharm).
Dyes for colorimetric detection
Instead of the use of SYBR Green, either calcein (Carl Roth, Karlsruhe, Germany) [32], neutral red (Carl Roth) or phenol red (Carl Roth) [33] was integrated into the LAMP master mix that was previously described in Allgöwer et al. [14, 15], and the amount of ultrapure water was adjusted according to the applied and published concentration of dyes. The crystal violet (Carl Roth) solution, described in Miyamoto et al. [34], was prepared, lyophilised in 200 µL reaction tubes and used in the LAMP master mix described in Allgöwer et al. [14]. In addition, the commercial master mix WarmStart Colorimetric LAMP 2X Master Mix was tested according to the manufacturer’s instructions (New England Biolabs, Frankfurt am Main, Germany).
Optimised colorimetric LAMP protocol
Five microliters of extracted DNA was added to 20 µL of a master mix, containing 1.4 mM each of dATP, dGTP, dTTP, dCTP (Carl Roth), 10 mM (NH4)2SO4 (Merck, Darmstadt, Germany), 8 mM MgSO4 (New England Biolabs), 50 mM KCl (Carl Roth), 0.1% v/v Tween® 20 (VWR, Darmstadt, Germany), 100 µM phenol red (Carl Roth), 0.32 U/µL Bst 2.0 WarmStart DNA Polymerase (New England Biolabs) and the previously published primers (0.2 µM of each forward F3 und reverse B3 primer; 0.8 µM of each FIP und biotin-FIP primer; 1.6 µM BIP primer; 0.3 µM LoopF primer; 0.1 µM FITC-LoopF primer; 0.4 µM LoopB primer) [14]. The start-pH value was adjusted to approx. pH 7.8 with 1 M KOH before addition of the DNA and the polymerase. After adding the DNA solution or the elution buffer as the negative control, the colour in the reaction tube should be pink and the pH value should be exactly at the colour change point of the phenol red. Isothermal primer annealing and DNA amplification were performed at 62 ℃ for 50 min.
LFD for amplicon detection
LFD detection of LAMP amplicons was performed using a commercially available dipstick (Milenia HybriDetect, Milenia Biotec GmbH, Gießen, Germany) as previously described [15]. In brief, 100 µL TBS buffer pH 7.4 incl. 1% Tween® 20 was mixed with 2 µL LAMP reaction solution. The test strip was dipped into the diluted LAMP amplicons and incubated for 5 min.
Lectin qPCR
The composition of the master mix as well as the protocol of real-time PCR (qPCR) targeting the Lectin Le1 gene according to the German Food and Feed law [30] is described in Allgöwer et al. [15]. This qPCR method was used as a qualitative comparative method. Briefly, 5 µL of sample DNA was added to 20 µL of master mix, followed by amplification in a real-time PCR cycler (MX 3005P™, Stratagene, via Agilent Technologies, Waldbronn, Germany). The temperature programme included the following steps: 1 cycle 50 ℃ for 2 min (uracil-N-glycosylase (UNG) activity), 1 cycle 95 ℃ for 10 min (deactivation of UNG, and activation of polymerase), 45 cycles 95 ℃ for 15 s (DNA denaturation) and 60 ℃ for 1 min (primer annealing and DNA amplification), and final drop down to 25 ℃ for 30 s (cooling). Fluorescence (6-Carboxyfluorescein (FAM)) was recorded after each cycle (absorbance at 488 nm, emission at 517 nm).
Results
Efficient visual detection facilitated by phenol red
Crystal violet (CV), calcein, neutral red, phenol red and a ready-to-use master mix were tested for colorimetric detection of LAMP products. CV, as a tri-aryl-methane dye, leads to a colour change from colourless to violet during DNA amplification through its binding to the major groove of double-stranded DNA [34]. The other dyes tested are indirect detectors of target DNA amplification. Neutral red and phenol red as pH indicators can be used for colorimetric detection since protons are released during amplification, leading to a reduction in the pH value [33]. The fluorescent property of calcein is quenched by the presence of free magnesium ions which, during LAMP, are complexed by released pyrophosphate [32]. All dyes were tested for suitability in the own LAMP master mix with regard to the criteria unambiguity of colour intensity and change, handling and complexity of preparation to use them in a colorimetric detection method (Fig. 2).
Colorimetric LAMP detection with a 100 µM phenol red, b 100 µM calcein, c 100 µM neutral red, d 500 µM CV, e WarmStart Colorimetric LAMP 2X Master Mix. Analysis of extracted soybean DNA, according to the elaborate CTAB extraction protocol of Allgöwer et al. [15] diluted at 1:102 to 1:10.7. Elution buffer served as no template control (NTC)
In this study, calcein under UV light did not indicate changes between negative sample (NTC) and samples with soybean DNA (Fig. 2b). Neutral red did neither allow unambiguous discrimination between NTC and soybean DNA (Fig. 2c). CV (Fig. 2d) and phenol red (Fig. 2a), added to the own master mix, and the commercial WarmStart Colorimetric LAMP 2X Master Mix (Fig. 2e) showed clear differences between reactions having NTC and soybean DNA. All three approaches led to the visual detection of reactions with down to 10–5 diluted soybean DNA by colour change. Since CV-based detection of the LAMP reaction in real-time requires preparation of reaction tubes, which includes immediate preparation of a CV-sodium sulphite-β-cyclodextrin solution and subsequent drying of an aliquot in the appropriate reaction tubes, CV appears unsuitable as a detection dye for a rapid test in our opinion. The commercial WarmStart Colorimetric LAMP 2X Master Mix and the self-made phenol red-based master mix were easy to handle and achieved a significant colour change. Hence, the most suitable results for this application were obtained with these two LAMP master mixes (Fig. 2a, e).
As a next step, the colorimetric LAMP based on both the optimised master mix with phenol red and the commercial ready-to-use master mix was tested on three food matrices, boiled sausage, instant tomato soup, and dark chocolate, which contained soybean at levels of 0, 10 and 100 mg/kg. Using the self-made master mix with phenol red, in all three foods discrete colorimetric discrimination from pink (negative) to yellow (positive) was obtained for soybean at the levels of 10 and 100 mg/kg versus 0 mg/kg and NTC, respectively (Fig. 3a). Comparable results were obtained using the commercial ready-to-use master mix (Fig. 3b). However, the difference in colour change between negative (pink) and positive (orange) reactions was less pronounced than with our optimised master mix with phenol red. Despite the convenience of a ready-to-use master mix, its composition remains unknown. For reason of known composition and clear discrimination between negative and positive reactions, the optimised master mix with phenol red was used in the following investigations.
a Optimised colorimetric LAMP with 100 µM phenol red and b with ready-to-use commercial LAMP master mix, both of DNA extracts according to Allgöwer et al. [15] from boiled sausage (1), instant tomato soup (2), and dark chocolate (3) at soybean levels of 0, 10 and 100 mg/kg. Elution buffer served as no template control (NTC)
Ultra-short cell lysis and silica-based DNA purification for rapid DNA preparation
The primary objective of the DNA extraction experiments was to reduce the extraction time significantly over our elaborate CTAB lysis, chloroform extraction, isopropanol precipitation, and additional purification using silica columns, which served as a reference. For this purpose, different rapid protocols were tested for their applicability of allergen detection in complex food matrices including a published protocol using a cellulose-based filter paper [31], which promises lysis and purification within 30 s (“30 s dipstick method”). Further, the commercial EchoLUTION Plant DNA kit for rapid lysis and single-step purification of genomic DNA (BioEcho Life Science) was investigated. Also, we combined the rapid EchoCLEAN DNA CleanUp kit (BioEcho Life Science) to purify DNA obtained from our CTAB lysis protocol. In addition, the FastPrep-24 high-speed benchtop homogenizer for rapid lysis in combination with the SureFood PREP Advanced kit (R-Biopharm) for purification of released DNA was evaluated for their suitability. The different DNA preparation protocols were tested using pure soybean and soybean incurred in the food matrices boiled sausage, instant tomato soup, and dark chocolate. The obtained DNA preparations were analysed using the optimised phenol red-based colorimetric LAMP protocol and the results are shown in Fig. 4. In addition, the unspiked matrices (boiled sausage, instant tomato soup, and dark chocolate) were analysed and no amplification-related colour change was detected (data not shown).
Comparative analysis of DNA extraction and purification protocols. Soybean and the food matrices boiled sausage, instant tomato soup, and dark chocolate with incurred soybean at 10 and 100 mg/kg are depicted. Elution buffer served as no template control (NTC). CTAB method served as a reference protocol for highly pure DNA. “Time required” means for lysis, DNA extraction and purification, excluding time for additional preparatory steps, if not otherwise specified
The use of the EchoLUTION Plant DNA kit for extraction and purification did not lead to a clear colour change of soybean positive samples, i.e. it did not achieve a sufficiently high quantity and/or quality of soybean DNA both from pure soybean and from soybean in complex food matrices. A similar result was observed for DNA purified with the EchoCLEAN DNA Clean Up kit subsequent to DNA extraction using the CTAB lysis protocol. The 30 s dipstick method was successful to isolate and purify DNA from soybean for amplification in LAMP, but it failed in two out of three complex food matrices with no colour change in LAMP for soybean in boiled sausage and dark chocolate, respectively. The DNA preparation from instant tomato soup already caused a pH drop before amplification and consequently no colour change related to specific amplification of soybean DNA was possible. The extraction method using the high-speed benchtop homogenizer FastPrep-24 in combination with subsequent purification via spin filter column using the SureFood PREP Advanced kit led to comparable results to the long-term established but elaborate CTAB in-house reference method. DNA extracted from pure soybean as well as from soybean in the three complex food matrices was successfully detected in LAMP by a change in colour (Fig. 4). Thus, this optimised method can substitute the elaborate overnight CTAB method to isolate and purify DNA in around 20 min for use in the colorimetric soybean LAMP.
Investigation of sensitivity of the optimised DNA preparation and colorimetric LAMP in incurred food matrices
The sensitivity of the optimised DNA preparation and colorimetric LAMP protocol was validated in a wider range of soybean levels (1 to 100,000 mg soybean per kg matrix) incurred in boiled sausage, instant tomato soup, and dark chocolate (Table 1). At and above a level of 10 mg/kg (0.001%), soybean was detectable in all foods, except that one of eight reactions of the spiked instant tomato soup tested negative. High soybean concentration between 100 mg/kg (0.01%) and 100,000 mg/kg (10%) was detectable in all eight LAMP reactions performed out of two independent DNA preparations (“Iso”). Furthermore, at lower soybean levels of 1 mg/kg (0.0001%), seven out of eight LAMP reactions tested positive in dark chocolate, indicating some more sensitivity in this matrix compared to boiled sausage and instant tomato soup, respectively (Table 1). Accordingly, the detectability can be assumed around 10 mg soybean per kg processed food, with potentially higher sensitivity in the chocolate matrix.
Applicability in selected retail food samples
The optimised method (DNA preparation and colorimetric LAMP) was applied to twelve commercial processed foods, seven of which contained soybean or products thereof in the ingredient list, two with a voluntary precautionary allergen labelling (PAL), such as “may contain …”, and three with no soybean declaration at all. In addition to colorimetric detection, DNA isolations were also analysed by qPCR and in selected cases samples were also investigated for the presence of LAMP amplicons by subsequent LFD (Table 2). In all but one sample with declared soybean ingredient, soybean was detectable in all LAMP reactions and confirmed by lectin qPCR. The sample with negative result contained soy sauce as ingredient. All three samples without any type of soybean declaration were negative in both LAMP and qPCR in all reactions. However, one sample (“cereal-potato-snack western style flavour”) reaction already changed colour prior to DNA amplification in LAMP. This sample was verified negative by LFD detection of LAMP reactions, and also in qPCR. One (“oat porridge”) of two samples with soybean PAL tested negative in LAMP and qPCR in all reactions. The other sample with soybean PAL (“hazelnut cookies”) tested positive in one of two reactions in each DNA isolation and in all applied methods, indicating a low amount of soybean around the limit of detection of all methods. In general, the results obtained by LAMP were in good agreement with the expected presence of soybean according to ingredient labelling. Furthermore, two independent DNA isolations were performed, each of which was investigated twice by colorimetric LAMP. Each DNA isolation (Iso 1 and Iso 2) showed the same result, confirming the reproducibility of the DNA extraction method. More details are addressed below in the discussion section.
Discussion
Efficient visual detection facilitated by phenol red
A lab environment free from contamination with amplified DNA is of special importance for DNA-based detection methods. The avoidance of back-contamination with amplification products from previous analyses is essential. The most efficient measure to prevent back-contamination is a strict spatial separation of the crucial steps of master mix preparation, amplification, and detection. Also, the permanent closure of reaction tubes during amplification and detection further adds to the prevention of back-contamination. In PCR-based methods, this is achieved by real-time detection using fluorescent dyes. This approach can also be applied to LAMP methods, although this requires sophisticated fluorescence readers. Alternatively, a direct colour change during amplification offers the advantage of a contamination-free workflow without such additional laboratory equipment. In this work, different dyes were tested for their suitability. Calcein as a metal ion indicator with fluorescence changes from weak orange to intense green, depending on the concentration of free magnesium ions. Therefore, calcein can in principle be used for detection because the magnesium ion concentration changes due to the complexing of pyrophosphate released during the reaction [32]. However, empirical testing of calcein in our soybean-specific LAMP did not allow a distinction between a positive and a negative reaction (Fig. 2b). For the optimal utilisation of calcein, an ideal magnesium ion concentration is required in the master mix and the final reaction approach. However, a negative impact of food matrices on the magnesium ion concentration cannot be excluded. Also, UV source is needed to evaluate the result. The use of neutral red as a pH indicator basically led to a colour change, but it was not distinct enough to allow an unambiguous yes-or-no evaluation (Fig. 2c). A clear colour-based distinction between positive and negative results was fulfilled when using CV, phenol red or the ready-to-use WarmStart Colorimetric LAMP 2X Master Mix. Colorimetric detection using CV showed a significant colour change from colourless-light blue to dark blue (Fig. 2d). One clear disadvantage of the use of CV is the laborious preparation of the reaction tubes. The fresh preparation of the CV-leuco crystal violet (LCV) solution is mandatory due to the instability of the LCV. Colour fluctuations may occur if the CV-LCV solution is not prepared immediately prior to LAMP (details not shown). This is related to the kinetic equilibrium between CV and LCV, which shifts in favour of the more stable CV over time [34]. CV is generally suitable for the detection of LAMP products, however, ease of handling should be essential for use in a rapid method. Additionally, CV has been classified as a carcinogen [35]. For these reasons, CV was not preferred as a detection dye in this work.
The ready-to-use WarmStart Colorimetric LAMP 2X Master Mix was applicable for the use of our soybean-specific rapid method, as shown in this work. Unambiguous detection of soybean in dilution levels 1:102 to 1:105 by visual detection was successfully achieved (Fig. 2e). The possibility of an easy and ready-to-use reaction mixture is convenient in routine analysis. However, there is no transparency of the composition of such a master mix. Moreover, we observed limitations when applied to complex food matrices where the change in colour from negative to positive samples was less clear than in pure soybean material (Fig. 3b). This led us to choose our self-prepared master mix that uses phenol red as indicative detection dye.
Phenol red as a pH indicator is highly suitable for the application of colorimetric detection based on the pH-dependent colour change. pH buffer substances such as tris(hydroxymethyl)aminomethane (Tris), which is a component of the master mix according to Allgöwer et al., can inhibit such a change [14, 15]. Therefore, the composition of the master mix was adjusted, e.g. by omitting Tris and adding different salts, for an optimal colour change whilst maintaining the amplification efficiency in LAMP. The system was tested using different soybean DNA dilutions. After the termination of LAMP, a clear colour change was visible at dilution levels 1:102 to 1:105 (Fig. 2a). Furthermore, colorimetric detection was successfully applied to three food matrices with incurred soybean, namely boiled sausage, instant tomato soup, and dark chocolate (Fig. 3a). The use of phenol red for colorimetric LAMP has so far been limited in the field of food analysis. More commonly, the detection system is used for the detection of pathogenic viruses or for the detection of diseases such as α-thalassemia-1 by a specific colour change from pink to yellow by amplification of a specific sequence segment of the α-globin gene [36, 37]. The results of these works in terms of an unambiguous colour change are comparable to the present ones, indicating that phenol red as a detection dye of LAMP is also suitable for the detection of soybean in food. In our application, the self-developed phenol red-based master mix provided similar or even superior results in complex foods when compared to the ready-to-use master mix. In contrast to LCV, phenol red is stable under normal ambient conditions, so that no decomposition of the substance is to be expected. Therefore, all required solutions can be aliquoted and used over an extended period of time.
Ultra-short cell lysis and silica-based DNA purification for rapid DNA preparation
The two selected commercial kits (EchoLUTION Plant DNA kit and EchoCLEAN DNA CleanUp kit) combined with prior extensive CTAB lysis according to Allgöwer et al. [15] were not successful in the tested matrices (Fig. 4). The published “30 s dipstick method” according to Zou et al. was promising with having an enormously short performance time [31]. DNA isolated and purified from soybean with this method was amplified in LAMP and visually detected positive (Fig. 4). However, the method failed in the complex food matrices boiled sausage, instant tomato soup, and dark chocolate. Failure of DNA amplification may be due to insufficient DNA quantity resulting from suboptimal lysis or insufficient removal of inhibitors during the purification step. The coelution of inhibitors, like polyphenols in dark chocolate, during extraction can reduce amplification efficiency, and lead to false-negative results, which was tested and shown elsewhere [38, 39].
Clear positive results from samples having soybean at various levels were obtained by the rapid lysis and extraction procedure using a high-speed benchtop homogenizer in combination with downstream purification with the commercial SureFood PREP Advanced kit. The DNA extraction from soybean as well as from the three more complex foods led to clear results in colorimetric LAMP, which were comparable with those obtained by the elaborate CTAB method (Fig. 4). Finally, a sufficiently high amount and purity of target DNA was achieved by one-minute lysis and according to protocol 1 of the SureFood PREP Advanced kit within around 20 min.
The combination of the optimised extraction procedure with a colorimetric detection in the soybean LAMP method led to a significant reduction in analysis time compared to Allgöwer et al., whilst avoiding potential back-contamination of subsequent runs with previous LAMP products.
Validation and application
The optimised DNA extraction and the LAMP method detected soybean levels at or above 10 mg/kg in boiled sausage as well as in instant tomato soup and at 1 mg/kg in dark chocolate, respectively (Table 1). The results are identical to those obtained with our previously reported elaborate CTAB-based DNA extraction and LAMP-LFD detection protocols [15]. Of the analysed commercial food samples, soybean was detectable in those containing soybean, soy flour, soya protein or soy lecithin as ingredients (Table 2). Except for one item, all foods with soybean declaration were detected positive. The product “Asian-flavoured rice crackers” contained highly processed soya sauce in which the amount of amplifiable DNA is too small. Reportedly, DNA-based detection of soybean in soy sauce is challenging [40]. The foods that did not contain soybean as an ingredient or labelled with a PAL claim tested negative. Two foods contained the statement “may contain traces of soy”. In one of the two, no soybean could be detected in four out of four replicates, whilst in the second, soybean was detected in two out of four reactions. This could be due to very small amounts of soybean at the limit of detection that has not been specifically determined in this matrix. In the case of commercial food sample “cereal-potato-snack western style flavour”, no colorimetric assessment of the result could be performed since a colour change already occurred before LAMP after addition of the DNA solution to the master mix. Matrix components in the extracted DNA, which could not be removed by purification according to protocol 1 of the SureFood PREP Advanced kit, led to a lowering of the pH value of the master mix and accordingly to a colour change to yellow. To solve this problem, the sample can be further processed with additional purification steps (data not shown) or the sample can be detected in additional qPCR or, more rapid, by subsequent 10 min LFD of existing LAMP amplicons since biotinylated and FITC-labelled primers are already used in our LAMP master mix.
In our previous study, all retail samples were also analysed with the elaborate protocols for CTAB-based DNA extraction and LAMP-LFD-based detection [15]. Notably, in all but one sample, we obtained the same results with the optimised DNA extraction and detection protocol in this work. The divergent sample “cereal bar with chocolate” tested negative in both LAMP and qPCR in this study but positive in the previous analysis. According to the ingredient labelling or PAL, there was no indication for soybean presence provided by the food manufacturer. Moreover, in our previous study, this sample tested negative using one commercial soybean LFD and faintly positive using another commercial soybean LFD, which altogether indicates a very low amount of soybean at or below the limit of detection of approx. 2–10 mg soybean per kg processed food according to the manufacturers of the commercial LFD tests [15].
The optimisation of DNA extraction and the detection reduced the time for sample preparation and analysis with our previously published LAMP method [14, 15] by several fold and down to approx. 1 h and 10 min (Fig. 5). Protein-based alternatives for the detection of soybean are rapid quantitative ELISA or qualitative LFD [5], which, in the case of commercial kits, usually require around 30 min (range 5–60 min) for protein extraction and detection [9, 15]. With our optimised protocols, we achieved rapid analysis time that is comparable to that of commercial protein-based LFD and ELISA kits. Thus, the DNA-based soybean LAMP presented in this study is considered a rapid method. Moreover, the technique and protocol is easy-to-perform with limited use of laboratory equipment. Further, it follows the principles according to “EU Directive 2010/63” [16], which aims at the reduction and replacement of animal-based reagents, such as animal antibodies in LFD and ELISA, as soon as alternatives are available.
The optimised rapid LAMP method proved suitable for the sensitive and specific screening of soybean in various processed food samples. In addition, the results can be confirmed by LFD-based detection of LAMP amplicons or orthogonal qPCR in case of doubt or for general confirmation since the optimised DNA extraction method proved compatible for subsequent qPCR analysis. Furthermore, the DNA extracted by this method can also be used for the detection of other allergens. In conclusion, we suggest the use of our optimised specific and sensitive LAMP for rapid detection of soybean in various processed foods as a screening method for on-site environments, such as food manufacturing, or for the identification of only positive samples that may require further quantitative verification with more elaborate and pricy techniques. Moreover, allergen detection in provocation meals for threshold determination before and after allergen-specific immunotherapy, which are both inclusion and exclusion criteria for clinical trials and success parameters of allergen immunotherapy, is an essential element of standardised administration. Hence, our new method may also allow quality assurance of clinical diagnostic food challenge preparations [6,7,8] by verification of a calculable soybean content in active and absence in placebo meals, respectively.
References
Ballmer-Weber BK, Holzhauser T, Scibilia J et al (2007) Clinical characteristics of soybean allergy in Europe: a double-blind, placebo-controlled food challenge study. J Allergy Clin Immunol 119:1489–1496. https://doi.org/10.1016/j.jaci.2007.01.049
Worm M, Eckermann O, Dölle S et al (2014) Triggers and treatment of anaphylaxis: an analysis of 4,000 cases from Germany, Austria and Switzerland. Dtsch Arztebl Int 111:367–375. https://doi.org/10.3238/arztebl.2014.0367
Patel N, Shreffler WG, Custovic A et al (2023) Will oral food challenges still be part of allergy care in 10 years’ time? J Allergy Clin Immunol Pract. https://doi.org/10.1016/j.jaip.2023.02.010
Sampson HA, van Gerth WR, Bindslev-Jensen C et al (2012) Standardizing double-blind, placebo-controlled oral food challenges: American Academy of Allergy, Asthma & Immunology-European Academy of Allergy and Clinical Immunology PRACTALL consensus report. J Allergy Clin Immunol 130:1260–1274. https://doi.org/10.1016/j.jaci.2012.10.017
Holzhauser T, Johnson P, Hindley JP et al (2020) Are current analytical methods suitable to verify VITAL® 2.0/3.0 allergen reference doses for EU allergens in foods? Food Chem Toxicol 145:111709. https://doi.org/10.1016/j.fct.2020.111709
Treudler R, Franke A, Schmiedeknecht A et al (2016) Standardization of double blind placebo controlled food challenge with soy within a multicentre trial. Clin Transl Allergy 6:39. https://doi.org/10.1186/s13601-016-0129-4
Holzhauser T, Franke A, Treudler R et al (2017) The BASALIT multicenter trial: Gly m 4 quantification for consistency control of challenge meal batches and toward Gly m 4 threshold data. Mol Nutr Food Res. https://doi.org/10.1002/mnfr.201600527
Blumchen K, Trendelenburg V, Ahrens F et al (2019) Efficacy, safety, and quality of life in a multicenter, randomized, placebo-controlled trial of low-dose peanut oral immunotherapy in children with peanut allergy. J Allergy Clin Immunol Pract 7:479-491.e10. https://doi.org/10.1016/j.jaip.2018.10.048
Schubert-Ullrich P, Rudolf J, Ansari P et al (2009) Commercialized rapid immunoanalytical tests for determination of allergenic food proteins: an overview. Anal Bioanal Chem 395:69–81. https://doi.org/10.1007/s00216-009-2715-y
Walker MJ, Burns M, Quaglia M et al (2018) Almond or Mahaleb? Orthogonal allergen analysis during a live incident investigation by ELISA, molecular biology, and protein mass spectrometry. J AOAC Int 101:162–169. https://doi.org/10.5740/jaoacint.17-0405
Röder M, Vieths S, Holzhauser T (2011) Sensitive and specific detection of potentially allergenic almond (Prunus dulcis) in complex food matrices by Taqman® real-time polymerase chain reaction in comparison to commercially available protein-based enzyme-linked immunosorbent assay. Anal Chim Acta 685:74–83. https://doi.org/10.1016/j.aca.2010.11.019
Baumert JL, Tran DH (2015) Lateral flow devices for detecting allergens in food. In: Handbook of food allergen detection and control. Elsevier, p 219–228
Civera A, Galan-Malo P, Segura-Gil I et al (2022) Development of sandwich ELISA and lateral flow immunoassay to detect almond in processed food. Food Chem 371:131338. https://doi.org/10.1016/j.foodchem.2021.131338
Allgöwer SM, Hartmann CA, Holzhauser T (2020) The development of highly specific and sensitive primers for the detection of potentially allergenic soybean (Glycine max) using loop-mediated isothermal amplification combined with lateral flow dipstick (LAMP-LFD). Foods. https://doi.org/10.3390/foods9040423
Allgöwer SM, Hartmann CA, Lipinski C et al (2020) LAMP-LFD based on isothermal amplification of multicopy gene ORF160b: applicability for highly sensitive low-tech screening of allergenic soybean (Glycine max) in food. Foods. https://doi.org/10.3390/foods9121741
DIRECTIVE 2010/63/EU OF THE EUROPEAN PARLIAMENT AND OF THE COUNCIL of 22 September 2010 on the protection of animals used for scientific purposes. Official J Eur Union L 276/33
Parida M, Sannarangaiah S, Dash PK et al (2008) Loop mediated isothermal amplification (LAMP): a new generation of innovative gene amplification technique; perspectives in clinical diagnosis of infectious diseases. Rev Med Virol 18:407–421. https://doi.org/10.1002/rmv.593
Moehling TJ, Choi G, Dugan LC et al (2021) LAMP diagnostics at the point-of-care: emerging trends and perspectives for the developer community. Expert Rev Mol Diagn 21:43–61. https://doi.org/10.1080/14737159.2021.1873769
Notomi T, Okayama H, Masubuchi H et al (2000) Loop-mediated isothermal amplification of DNA. Nucleic Acids Res 28:E63. https://doi.org/10.1093/nar/28.12.e63
Yuan D, Kong J, Li X et al (2018) Colorimetric LAMP microfluidic chip for detecting three allergens: peanut, sesame and soybean. Sci Rep 8:8682. https://doi.org/10.1038/s41598-018-26982-5
Sheu S-C, Tsou P-C, Lien Y-Y et al (2020) Rapid and specific detection of mango (Mangifera indica) in processed food using an isothermal nucleic acid amplification assay. Eur Food Res Technol 246:759–766. https://doi.org/10.1007/s00217-020-03440-z
Zhang F, Gao C, Bai L et al (2022) Dual-color blending based visual LAMP for food allergen detection: a strategy with enlarged color variation range and contrast. Food Chem 13:100201. https://doi.org/10.1016/j.fochx.2021.100201
Mao R, Xie K, Zhao M et al (2020) Development and evaluation of a loop-mediated isothermal amplification (LAMP) assay for rapid detection of pistachio (Pistacia vera) in food samples. Food Anal Methods 13:658–666. https://doi.org/10.1007/s12161-019-01684-4
Sheu S-C, Yu M-T, Lien Y-Y et al (2020) Development of a specific isothermal nucleic acid amplification for the rapid and sensitive detection of shrimp allergens in processed food. Food Chem 332:127389. https://doi.org/10.1016/j.foodchem.2020.127389
Zahradnik C, Martzy R, Mach RL et al (2014) Detection of the food allergen celery via loop-mediated isothermal amplification technique. Anal Bioanal Chem 406:6827–6833. https://doi.org/10.1007/s00216-014-7873-x
Kim H-R, Suh S-M, Kang H-B et al (2022) Duplex loop-mediated isothermal amplification assay for peanut (Arachis hypogaea) and almond (Prunus dulcis) detection of allergen coding genes. Food Control 138:109003. https://doi.org/10.1016/j.foodcont.2022.109003
Sun M, Gao H, Xiao X et al (2015) A novel loop-mediated isothermal amplification method for detection of the carrot materials in foods. Eur Food Res Technol 241:295–302. https://doi.org/10.1007/s00217-015-2459-1
Deb R, Sengar GS, Singh U et al (2016) Application of a loop-mediated isothermal amplification assay for rapid detection of cow components adulterated in buffalo milk/meat. J Mol Biol 58:850–860. https://doi.org/10.1007/s12033-016-9984-4
Wang Y, Yu Z, Liu Y (2022) A high sensitivity method of closed-tube loop-mediated isothermal amplification developed for visual and rapid detection of cow milk adulteration. Int Dairy J 127:105214. https://doi.org/10.1016/j.idairyj.2021.105214
Official Collection of Test Methods (2012) Detection and quantification of mustard (Sinapis alba) and soybean (Glycine max) in boiled sausages with real-time PCR. German Food Feed Law-Food Anal 64:L 08.00-59
Zou Y, Mason MG, Wang Y et al (2017) Nucleic acid purification from plants, animals and microbes in under 30 seconds. PLoS Biol 15:e2003916. https://doi.org/10.1371/journal.pbio.2003916
Tomita N, Mori Y, Kanda H et al (2008) Loop-mediated isothermal amplification (LAMP) of gene sequences and simple visual detection of products. Nat Protoc 3:877–882. https://doi.org/10.1038/nprot.2008.57
Tanner NA, Zhang Y, Evans TC (2015) Visual detection of isothermal nucleic acid amplification using pH-sensitive dyes. Biotechniques 58:59–68. https://doi.org/10.2144/000114253
Miyamoto S, Sano S, Takahashi K et al (2015) Method for colorimetric detection of double-stranded nucleic acid using leuco triphenylmethane dyes. Anal Biochem 473:28–33. https://doi.org/10.1016/j.ab.2014.12.016
Mani S, Bharagava RN (2016) Exposure to Crystal Violet, its toxic, genotoxic and garcinogenic effects on environment and its degradation and detoxification for environmental safety. Rev Environ Contam Toxicol 237:71–104. https://doi.org/10.1007/978-3-319-23573-8_4
Kaarj K, Akarapipad P, Yoon J-Y (2018) Simpler, faster, and sensitive Zika virus assay using smartphone detection of loop-mediated isothermal amplification on paper microfluidic chips. Sci Rep 8:12438. https://doi.org/10.1038/s41598-018-30797-9
Chomean S, Pholyiam K, Thamwarokun A et al (2018) Development of visual detection of α-thalassemia-1 (the – – SEA deletion) using pH-sensitive loop-mediated isothermal amplification. Hemoglobin 42:171–177. https://doi.org/10.1080/03630269.2018.1488723
Schrader C, Schielke A, Ellerbroek L et al (2012) PCR inhibitors—occurrence, properties and removal. J Appl Microbiol 113:1014–1026. https://doi.org/10.1111/j.1365-2672.2012.05384.x
Costa J, Melo VS, Santos CG et al (2015) Tracing tree nut allergens in chocolate: a comparison of DNA extraction protocols. Food Chem 187:469–476. https://doi.org/10.1016/j.foodchem.2015.04.073
Mafra I, Silva SA, Moreira EJ et al (2008) Comparative study of DNA extraction methods for soybean derived food products. Food Control 19:1183–1190. https://doi.org/10.1016/j.foodcont.2008.01.004
Acknowledgements
We gratefully acknowledge technical support by Elke Völker and critical manuscript review by Vera Mahler.
Funding
Open Access funding enabled and organized by Projekt DEAL. This research received no external funding.
Author information
Authors and Affiliations
Contributions
Conceptualization: TH and SA. Methodology: LS and SA. Validation: LS. Formal analysis: LS and TH. Investigation: LS. Data curation: LS and TH. Writing-original draft: LS. Writing-review and editing: LS, TH and SA. Project administration: TH. Funding acquisition: TH. Resources: TH. Supervision: TH.
Corresponding author
Ethics declarations
Conflict of interest
The authors have no relevant financial or non-financial interests to disclose.
Compliance with ethics requirements
This work does not contain any studies with humans or animals.
Additional information
Publisher's Note
Springer Nature remains neutral with regard to jurisdictional claims in published maps and institutional affiliations.
Rights and permissions
Open Access This article is licensed under a Creative Commons Attribution 4.0 International License, which permits use, sharing, adaptation, distribution and reproduction in any medium or format, as long as you give appropriate credit to the original author(s) and the source, provide a link to the Creative Commons licence, and indicate if changes were made. The images or other third party material in this article are included in the article's Creative Commons licence, unless indicated otherwise in a credit line to the material. If material is not included in the article's Creative Commons licence and your intended use is not permitted by statutory regulation or exceeds the permitted use, you will need to obtain permission directly from the copyright holder. To view a copy of this licence, visit http://creativecommons.org/licenses/by/4.0/.
About this article
Cite this article
Schäfer, L., Allgöwer, S. & Holzhauser, T. Rapid DNA extraction and colorimetric amplicon visualisation speed up LAMP-based detection of soybean allergen in foods. Eur Food Res Technol 249, 2875–2886 (2023). https://doi.org/10.1007/s00217-023-04334-6
Received:
Revised:
Accepted:
Published:
Issue Date:
DOI: https://doi.org/10.1007/s00217-023-04334-6