Abstract
Lead (Pb), a widespread contaminant in terrestrial landscape, is highly detrimental to plant and animal life. Specifically, Pb-contaminated soils cause a sharp decrease in crop productivity, thereby posing a serious risk to agriculture. A study was planned to investigate the toxic effect of Pb2+ (0, 16, 40 and 80 mg L−1) in the seedlings of maize (Zea mays), in terms of induced physiological and biochemical changes at initial hours of treatment (0–8 h). Increased accumulation of malondialdehyde (MDA) served as an indicator of cellular peroxidation. At 80 mg L−1 Pb2+, MDA content enhanced over the control by 175% after 2 h of exposure and increased further to 461% greater over the control after 8 h of exposure. Elevated superoxide ion (O −.2 ) and H2O2 contents suggested oxidative damage to the plants. The level of H2O2 increased over control by 70%, 80%, 135% and 182% at 2, 4, 6, and 8 h after exposure to 16 mg L−1 Pb2+, respectively. In situ histochemical localization confirmed the level of lipid peroxides, increased accumulation of OO −.2 and loss of membrane integrity upon Pb2+ treatment. Pb2+-induced oxidative stress triggered significant changes in the activities of antioxidant enzymes. A concentration-dependent increase was observed in the activities of the superoxide dismutase (SOD), ascorbate peroxidase (APX), guaiacol peroxidase (GPX) and glutathione reductase (GR) in response to Pb2+, whereas catalases (CAT) was not able to provide protection against oxidative stress. These observations imply that Pb2+ -induced oxidative stress during initial period (0–8 h) of exposure involved ROS accumulation and upregulation of scavenging enzymes except CAT as a defense against Pb2+-toxicity.
Similar content being viewed by others
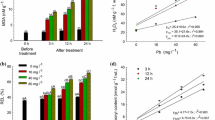
References
Alscher R.G., Erturk N. & Heath L.S. 2002. Role of superoxide dismutases (SODs) in controlling oxidative stress in plants. J. Exp. Bot. 53: 1331–1341.
Beauchamp C. & Fridovich I. 1971. Superoxide dismutase: improved assays and an assay applicable to acrylamide gels. Anal. Biochem. 44: 276–286.
Boveris A., Cadenas E. & Chance B. 1980. Low level chemiluminescence of the lipoxygenase reaction. Photobiochem. Photobiophys. 1: 175–182.
Cakmak I. & Marschner H. 1992. Magnesium deficiency and high light intensity enhance activities of superoxide dismutase, ascorbate peroxidase, and glutathione reductase in bean leaves. Plant Physiol. 98: 1222–1227.
Choudhury S. & Panda S.K. 2005. Toxic effects oxidative stress and ultrastructural changes in moss Taxithelium nepalense (Schwaegr.) Broth under chromium and lead phytotoxicity. Water Air Soil Pollut. 167: 73–90.
Devi S.R. & Prasad M.N.V. 1998. Copper toxicity in Ceratophyllum demersum L (Coontail), a free floating macrophyte: response of antioxidant enzymes and antioxidants Plant Sci. 138: 157–165.
Dixit V., Vivek P. & Shyam R. 2001. Differential antioxidative responses to cadmium in root and leaves of pea (Pisum sativum L. cv. Azad). J. Exp. Bot. 52: 1101–1109.
Dogan M., Saygideger S.D. & Colak U. 2009. Effect of lead toxicity on aquatic macrophyte Elodea canadensis Michx. Bull. Environ. Contam. Toxicol. 83: 249–254.
Egley G.H., Paul R.N., Vaughn K.C. & Duke S.O. 1983. Role of peroxidase in the development of water impermeable seed coats in Sida spinosa L. Planta 157: 224–232.
Ekmek¸ci Y., Tanyolac D. & Ayhan B. 2009. A crop tolerating oxidative stress induced by excess lead: maize. Acta Physiol. Plant. 31: 319–330.
Feieraband J. & Engel S. 1986. Photoinactivation of catalase in vitro and in leaves. Arch. Biochem. Biophys. 251: 567–576.
Foyer C.H., Souriau N., Perret S., Lelandais M., Kunert K.J., Pruvost C. & Jouanin L. 1995. Overexpression of glutathione reductase but not glutathione synthetase leads to increases in antioxidant capacity and resistance to photoinhibition in poplar trees. Plant Physiol. 109: 1047–1057.
Foyer C.H. & Halliwell B. 1976. The presence of glutathione and glutathione reductase in chloroplasts: A proposal role in ascorbic acid metabolism. Planta 133: 21–25.
Fridovich I. 1978. The biology of oxygen radicals. Science 201: 875–880.
Gaw˛eda M. 2007. Changes in the contents of some carbohydrates in vegetables cumulating Lead. Pol. J. Environ. Stud. 16: 57–62.
Godbold D.Y. & Ketner C. 1991. Lead influences root growth and mineral nutrition of Picea abies seedlings. J. Plant Physiol. 139: 95–99.
Gratäo P.L., Polle A, Lea P.J. & Azevedo R.A. 2005. Making the life of heavy metal-stressed plants a little easier. Funct. Plant Biol. 32: 481–494.
Gupta D.K., Nicoloso F.T., Schetinger M.R.C., Rossato L.V., Pereira L.B., Castro G.Y., Srivastava S. & Tripathi R.D. 2009. Antioxidant defense mechanism in hydroponically grown Zea mays seedlings under moderate lead stress. J. Hazard. Mater. 172: 479–484.
Heath R.L. & Packer L. 1968. Photoperoxidation in isolated chloroplast I Kinetics and stoichiometry of fatty acid peroxidation. Arch. Biochem. Biophys. 125: 189–198
Kaur G., Singh H.P., Batish D.R. & Kohli R.K. 2012. Growth, photosynthetic activity and oxidative stress in wheat (Triticum aestivum) after exposure of lead to soil. J. Environ. Biol. 33: 265–269.
Kaur G., Singh H.P., Batish D.R. & Kohli R.K. 2012. Lead (Pb)-induced biochemical and ultrastructural changes in wheat (Triticum aestivum) roots. Protoplasma 250: 53–62
Keser G. & Saygideger S. 2010. Effects of Pb on the activities of antioxidant enzymes in water cress, Nasturtium officinale R Br. Biol. Trace. Elem. Res. 137: 235–243.
Kopyra M. & Gwozdz E.A. 2003. Nitric oxide stimulates seed germination and counteracts the inhibitory effect of heavy metals and salinity on root growth of Lupinus luteus. Plant Physiol. Biochem. 41: 1011–1017.
Lamb D.T., Ming H., Megharaj M. & Naidu R. 2010. Phytotoxicity and accumulation of lead in Australian native vegetation. Arch. Environ. Contam. Toxicol. 58: 613–621.
Lee K.C., Cunningham B.A., Paulsen G.M., Liang G.H. & Moore R.B. 1976. Effects of cadmium on respiration rate and activities of several enzymes in soybean seedlings. Physiol. Plant. 36: 4–6.
Liu D., Zou J., Meng Q., Zou J. & Jiang W. 2009. Uptake and accumulation and oxidative stress in garlic (Allium sativum L) under lead phytotoxicity. Ecotoxicology 18: 134–143.
Lowry O.H., Rosebrough N.J., Farr A.L. & Randall R.J. 1951. Protein estimation with Folin-phenol reagent. J. Biol. Chem. 193: 265–278.
Małecka A., Piechalak A. & Tomaszewska B. 2009. Reactive oxygen species production and antioxidative defense system in pea root tissues treated with lead ions: the whole roots level. Acta Physiol. Plant. 31: 1053–1063.
Misra H.R. & Fridovich I. 1972. The univalent reduction of oxygen by reduced flavins and quinines. J. Biol. Chem. 247: 188–192.
Mittler R. 2002. Oxidative stress, antioxidants and stress tolerance. Trends Plant Sci. 7: 405–410.
Mustafa M.G. 1990. Biochemical basis of ozone toxicity. Free Rad. Biol. Medic. 9: 245–265.
Nakano Y. & Asada K. 1981. Hydrogen peroxide is scavenged by ascorbate-specific peroxidase in spinach chloroplasts. Plant Cell Physiol. 22: 867–880.
Piotrowska A., Bajguzn A., Godlewska-Zyłkiewicz B. & Zambrzycka E. 2010. Changes in growth biochemical components and antioxidant activity in aquatic plant Wolffia arrhiza (Lemnaceae) exposed to cadmium and lead. Arch. Environ. Contam. Toxicol. 58: 594–604.
Pompella A., Maellaro E., Casini A.F. & Comporti M. 1987. Histochemical detection of lipid peroxidation in the liver of bromobenzene-poisoned mice. Am. J. Pathol. 129: 295–301.
Qureshi M.I., Israr M., AbdinM.Z. & Iqbal M. 2005. Responses of Artemisia annua L. to lead and salt-induced oxidative stress. Environ. Exp. Bot. 53: 185–193.
Reddy A.M., Kumar S.G., Jyothsnakumari G., Thimmanaik S. & Sudhakar C. 2005. Lead induced changes in antioxidant metabolism of horsegram (Macrotyloma uniflorum (Lam.) Verdc.) and bangalgram (Cicer arietinum L.). Chemosphere 60: 97–104.
Ruley A.T., Sharma N.C. & Sahi S.V. 2004. Antioxidant defense in a lead accumulating plant, Sesbania drummmondi. Plant Physiol. Biochem. 2: 899–906.
Sandalio L.M., Dalurzo H.C., Gómez M., Romero-Puertas M.C. & del Rio L.A. 2001. Cadmium induces changes in the growth and oxidative metabolism of pea plants. J. Exp. Bot. 52: 2115–2126.
Sengar R.S., Gautam M., Sengar R.S., Garg S.K., Sengar K. & Chaudhary R. 2008. Lead stress effects on physiobiochemical activities of higher plants. Rev. Environ. Contam. Toxic. 196: 73–93.
Sobrino A.S., Miranda M.G., Alvarez C. & Quiroz A. 2010. Bioaccumulation and toxicity of lead (Pb) in Lemna gibba L. (duckweed). J. Environ Sci. Health 45: 107–110.
Stone J.R. & Yang S. 2006. Hydrogen peroxide: a signaling messenger. Antioxid. Redox. Signal. 8: 243–270.
Singh H.P., Batish D.R., Kaur G., Arora K. & Kohli R.K. 2008. Nitric oxide (as sodium nitroprusside) supplementation ameliorates Cd toxicity in hydroponically grown wheat roots. Environ. Exp. Bot. 63: 158–167.
Singh R.P., Tripathi R.D., Sinha S.K., Maheswari R. & Srivastava H.S. 1997. Response of higher plants to lead contaminated environment. Chemosphere 32: 2467–2493.
Velikova V., Yordanov I. & Edreva A. 2000. Oxidative stress and some antioxidant systems in acid rain treated bean plants. Plant Sci. 151: 59–66.
Verma S. & Dubey R.S. 2003. Lead toxicity induces lipid peroxidation and alters the activities of antioxidant enzymes in growing rice plants. Plant Sci. 164: 645–655.
Wierzbicka M. & Obidzinska J. 1998. The effect of lead on seed imbibition and germination in different plant species. Plant Sci. 137: 155–171.
Acknowledgements
Gurpreet Kaur is thankful to University Grants Commission (New Delhi, India) for research fellowship.
Author information
Authors and Affiliations
Corresponding author
Rights and permissions
About this article
Cite this article
Kaur, G., Singh, H.P., Batish, D.R. et al. Adaptations to oxidative stress in Zea mays roots under short-term Pb2+ exposure. Biologia 70, 190–197 (2015). https://doi.org/10.1515/biolog-2015-0023
Received:
Accepted:
Published:
Issue Date:
DOI: https://doi.org/10.1515/biolog-2015-0023