Abstract
Background
Elevated serum levels of serum amyloid A (SAA) are associated with increased risk of cardiovascular disease. In this study, we examine associations between allelic variation in the rs11024595 single nucleotide polymorphism (SNP) in the 5’ flanking region of the SAA1 gene and adipose tissue gene expression, serum levels of SAA and cardiovascular risk factors.
Methods
DNA samples from 729 participants in the SibPair study, comprising weight discordant siblings and their biological parents, and 3542 participants (1783 patients treated with bariatric surgery and 1759 controls) from the Swedish Obese Subjects (SOS) study were used. The rs11024595 SNP was genotyped in both cohorts using Pyrosequencing or the Sequenom MassARRAY platform, respectively. Blood chemistry and anthropometry were assessed at study start. Adipose tissue SAA1 gene expression and serum levels of SAA in the SibPair study were analyzed with DNA microarray or immunoassay, respectively.
Results
In the SibPair study, the rs11024595 SNP was associated with serum levels of SAA (P = 0.0050) where T allele carriers displayed lower levels of SAA (P = 0.0025) but no association between genotype and adipose tissue SAA1 gene expression was found. In the SOS study, the rs11024595 SNP was associated with serum levels of HDL cholesterol (P = 0.0045), triglycerides (P = 0.025) and apolipoprotein E (P = 0.026). Moreover, T allele carriers had lower levels of HDL cholesterol (P = 0.0148), but higher levels of triglycerides (P = 0.0418) and apolipoprotein E (P = 0.028) compared to C allele homozygotes. The rs11024595 SNP was also associated with plasma glucose (P = 0.044).
Conclusions
The rs11024595 SNP in the 5’ flanking region of the SAA1 gene is associated with both serum levels of SAA and other cardiovascular risk factors. Future studies are required to elucidate whether the rs11024595 SNP can affect the risk of cardiovascular events.
Trial registration
ClinicalTrials.gov Identifier: NCT01479452 Registered 24 November 2011 - retrospectively registered.
Similar content being viewed by others
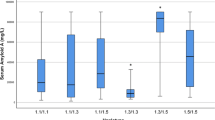
Background
Established risk factors for cardiovascular disease include obesity, smoking, diabetes, dyslipidemia and hypertension [1,2,3]. In addition, studies have shown that chronic inflammation plays a role in the pathophysiology of atherosclerosis [4], and markers of inflammation, such as serum amyloid A (SAA), are modestly elevated in patients with atherosclerosis and in patients with obesity and insulin resistance [5,6,7,8,9].
SAA functions as an exchangeable apolipoprotein [10], mainly of high density lipoprotein (HDL) [10] but also of low density lipoprotein (LDL) and very low density lipoprotein (VLDL) [11]. It has been suggested that SAA modifies the function of lipoproteins resulting in a proatherogenic effect [12], although the role of SAA in the pathophysiology of cardiovascular disease is not clear [13]. Serum levels of SAA are usually in the range of 1–2 µg/ml in healthy individuals but can rise 1000-fold during an acute phase reaction [14] due to increased production of SAA in the liver [14]. However, during non-acute phase we [7, 15] and others [16] have previously shown that adipocytes are the main source of SAA in individuals with obesity, and both SAA expression in adipose tissue and serum levels of SAA are reduced after diet-induced weight loss [15].
In humans, SAA comprises a family of proteins encoded by four different SAA genes (SAA1, SAA2, SAA3, SAA4) located at chromosome 11p15.1. The SAA1 and SAA2 genes encode the acute phase SAA and share the most homology among the SAA gene family [14]. The promoter regions of the SAA1 and SAA2 genes contain transcription factor recognition sequences that allow induction of gene expression by IL-1, IL-6, NF kappa-B [17,18,19] and SAA activating factor [20]. The rs11024595 SNP is located in the 5’ flanking sequence of the SAA1 coding region and thus could possibly affect SAA1 transcription [21]. However, whether this SNP has an impact on SAA1 gene expression in adipose tissue, serum levels of SAA or is associated with cardiovascular risk factors has not been investigated.
We here examine if allelic variation in the rs11024595 SNP (-13T/C) in the 5’ flanking region of the SAA1 gene influences SAA1 gene expression in adipose tissue, serum levels of SAA and if the rs11024595 SNP is associated with cardiovascular risk factors in the SOS study comprising of patients with obesity and the SibPair study, a study of adult weight discordant siblings and their biological parents.
Methods
Ethics statement
All study protocols were approved by seven regional ethics committees (Göteborg, Linköping, Lund/Malmö, Stockholm, Umeå, Uppsala and Örebro). All participants gave informed consent to participate. The studies were performed in accordance with the Declaration of Helsinki.
The SibPair study
The SibPair study consists of 732 individuals from 154 families with a pair of adult siblings with a BMI difference of 10 kg/m2 or more [22]. DNA was only available for 729 individuals, who were included in the current analysis. An examination including venous blood sampling was performed at study start. Blood chemistry was analyzed at the central laboratory of the Sahlgrenska University Hospital (accredited according to International Organization for Standardization/International Electrochemical Commission 15189:2007 standards). Analysis of serum levels of SAA was performed on frozen serum from 654 individuals with a human SAA immunoassay kit (Invitrogen, Carlsbad, CA, USA) according to the protocol.
Levels of SAA1 gene expression in subcutaneous adipose tissue were available for 376 offsprings from the SibPair study. RNA extraction and subsequent gene expression analysis with DNA microarray (Human Genome U133 plus 2.0 Affymetrix, Santa Clara, CA, USA) was performed as previously described [23]. SAA1 gene expression was assessed by the 214456_x_at probe set.
The swedish obese subjects (SOS) Study
The SOS study is an ongoing study with 2007 patients with obesity who underwent bariatric surgery, and 2040 matched controls who received usual obesity care at primary health care centers as previously described [22]. In brief, the patients were recruited between September 1, 1987 and January 31, 2001. Inclusion criteria were a body mass index (BMI) of 34 kg/m2 or more for men and 38 kg/m2 or more for women and an age between 37 and 60 years [22]. A physical examination including venous blood sampling was performed at study start. All blood chemistry was analyzed at the central laboratory, Sahlgrenska University Hospital.
Genotyping of the rs11024595 SNP
In the SibPair study, the SAA1 gene was amplified from DNA using PCR (Applied Biosystems, Foster City, CA, USA) and subsequent genotyping of the rs11024595 SNP was performed with Pyrosequencing using the PyroMark Gold Q96 platform (Qiagen, Hilden, Germany). Primer sequences for SAA1 DNA amplification and the probe sequence for genotyping of the rs11024595 SNP are presented in supplementary Table 1.
In the SOS study, the rs11024595 SNP was genotyped using the Sequenom MassARRAY platform (Sequenom, San Diego, CA, USA) at the Mutation Analysis Facility, MAF, Clinical Research Center, Karolinska University Hospital, Stockholm, Sweden. The MassARRAY Assay Design 3.1 Software (Sequenom) was used for assay design.
Statistical analyses
Genotype frequencies were analyzed for deviations from the Hardy-Weinberg equilibrium. Differences in baseline characteristics were analyzed with independent T-test or Fisher’s exact test in the SOS study and with mixed model adjusted for non-independence among related individuals in the SibPair study. All continuous variables were transformed by Box-Cox power transformation to obtain approximate normal distribution prior to analysis. A mixed model analysis was used to assess the association of the rs11024595 SNP and adipose tissue SAA1 gene expression, SAA serum levels and cardiovascular risk factors in the SibPair study. The mixed model analyses were adjusted for BMI, age, sex and non-independence among related individuals in all analyses except for analyses including indices for adiposity (BMI and sagittal diameter) that were adjusted only for sex, age and non-independence among related individuals. Due to convergence problems, seven individuals from four families were excluded in the mixed model analysis with genotype data. General linear models, adjusted for age and sex, were used for analyses of associations between the rs11024595 SNP with anthropometry and cardiovascular risk factors in the SOS study. In analyses not including indices of adiposity, models were also adjusted for BMI. The parameter estimates from the models provide an adjusted estimate of difference in mean values among the C allele homozygotes compared with the T allele carriers. All analyses were performed as dominant genetic models because of few T allele homozygotes. All statistical analyses were performed with SAS v.9.4 (SAS institute, Cary, North Carolina, USA).
Results
Participant characteristics
Characteristics of participants in the SibPair study and the SOS study are presented in Tables 1 and 2, respectively. No significant differences in proportion of men/women or age were observed between the T allele carriers and homozygote carriers of the C allele in neither the SibPair study, nor the SOS study (Tables 1 and 2, respectively).
In the SibPair study, a 98.6% success rate yielded genotypes for 719 subjects. Of these, 623 participants (86.6%) were homozygotes for the C allele, 91 participants (12.7%) were carriers of the heterozygotes (C/T) and 5 participants (0.7%) were homozygotes for the T allele.
In the SOS study, a 99.3% success rate yielded genotypes for 3542 subjects. Of these, 3146 (88.8%) were homozygotes for the C allele, 379 were (10.7%) heterozygotes (C/T) and 17 participants (0.5%) were homozygotes for the minor T allele.
Genotypes were in Hardy-Weinberg equilibrium in both cohorts. No mendelian inconsistencies were found in the SibPair study.
The rs11024595 SNP, serum levels of SAA and adipose tissue SAA1 gene expression in the SibPair study
We have previously shown that adipocytes are the main source of SAA in individuals with obesity [7, 15] and that adipose tissue gene expression is associated with serum levels of SAA [15]. The association between adipose tissue SAA1 gene expression and serum levels of SAA was also confirmed in the SibPair study (P < 0.0001; parameter estimate 0.26; 95% CI 0.14–0.37). Since the rs11024595 SNP is located in the 5’ flanking region of the SAA1 gene [21] it may influence SAA1 gene transcription. A bioinformatic analysis for the rs11024595 SNP was performed on Gene Browser UCSC using the tool JASPAR CORE 2022 to search for predicted transcription factors binding sites that could be affected by the SNP [24]. Three transcription factors were identified, CTCFL (CCCTC-binding factor like), ZNF610 (Zinc finger protein 610) and ISNM1 (INSM transcriptional repressor 1), but we were unable to identify reports suggesting an effect of these factors on SAA1 transcription from the literature. Moreover, we investigated associations between the rs11024595 SNP and serum levels of SAA and adipose tissue SAA1 gene expression. The rs11024595 SNP was associated with serum levels of SAA (P = 0.0050; parameter estimate 0.32; 95% CI 0.10–0.53), and serum SAA levels were lower in the T allele carriers compared with the C allele homozygotes (P = 0.0025; Fig. 1 A). However, no association between the rs11024595 SNP and SAA1 gene expression in adipose tissue was observed (P = 0.99; parameter estimate − 0.00098; 95% CI -0.25–0.25) and there was no difference in SAA1 adipose tissue gene expression between the C allele homozygotes and carriers of the T allele (P = 0.27; Fig. 1B).
Serum levels of SAA and cardiovascular risk factors in the SibPair study
Elevated serum levels of SAA are associated with increased risk of cardiovascular disease [5, 6] so we aimed to investigate associations between serum levels of SAA and cardiovascular risk factors. In the SibPair study, serum levels of SAA were positively associated with indices of adiposity (BMI, sagittal diameter, body fat) and metabolic parameters such as s-insulin, HOMA-IR, s-cholesterol, and s-triglycerides. P-values and parameter estimates for the associations between serum levels of SAA and investigated metabolic parameters are shown in supplementary Table 2.
The rs11024595 SNP and cardiovascular risk factors in the SOS study and SibPair study
Serum levels of SAA were associated with multiple cardiovascular risk factors in the SibPair study and our data also show an association between rs11024595 genotype and serum levels of SAA. Hence, we analyzed whether there were significant associations between the rs11024595 SNP and metabolic parameters. Serum levels of triglycerides, HDL-cholesterol and apolipoprotein E (ApoE) were associated with the rs11024595 SNP in the SOS study (Table 3). T allele carriers had higher serum levels of triglycerides, lower serum levels of HDL-cholesterol and higher levels of ApoE compared with the C allele homozygotes (P = 0.0418; P = 0.0148; P = 0.0280 respectively). Furthermore, an association between the rs11024595 SNP and p-glucose levels was observed (P = 0.044). An association between the SNP rs11024595 and serum levels of triglycerides was also found in the SibPair study (P = 0.041, Table 3).
Discussion
We here demonstrate that allelic variation in the rs11024595 SNP located in the 5’ flanking region of the SAA1 gene is associated with serum levels of SAA and cardiovascular risk factors such as HDL-cholesterol, apoE, triglycerides and p-glucose. T allele carriers displayed lower serum levels of SAA, which according to previous studies, could be cardioprotective [5, 6]. However, T allele carriers also displayed a worse metabolic profile with respect to lipid metabolism, which seems contradictive in relation to the lower SAA levels in terms of cardiovascular risk.
Our results show that the rs11024595 SNP is associated with serum levels of SAA and that the T allele carriers display significantly lower levels. We [7, 15] and others [16] have previously shown that adipocytes are the main source of SAA in individuals with obesity and our initial hypothesis was that the rs11024595 SNP could alter the adipose tissue SAA1 gene expression and thereby affect the serum levels of SAA. However, adipose tissue SAA1 gene expression was similar in the different genotype groups in our study. A previous study has shown that the T allele of the rs11024595 SNP is associated with higher SAA gene expression in a hepatocyte cell line [25], which is contradictive to our results if the higher SAA gene expression in liver also results in higher serum levels of SAA. However, there are other mechanisms that could explain lower serum levels of SAA in the T allele carriers in our study. The T allele of the rs11024595 SNP has been reported to be in strong linkage disequilibrium to the SAA1.1 haplotype in a Caucasian population [21], and a previous study has shown that carriers of the SAA1.1 haplotype have a faster plasma clearance than carriers of other SAA haplotypes (SAA1.5) which could result in lower serum levels of SAA [26].
The T allele of the rs11024595 SNP was associated with higher levels of triglycerides and lower levels of HDL-cholesterol. Low HDL-cholesterol is traditionally associated with a higher risk of cardiovascular disease [27] because of the cardioprotective role of HDL in the reverse cholesterol transport [28]. However, the T allele carriers in our study also displayed lower levels of SAA which in previous studies have been associated with a lower cardiovascular risk [5, 6, 8]. Previous studies investigating links between serum levels of SAA and the risk for cardiovascular event report different results regarding the relationship between serum levels of SAA and HDL-cholesterol. While Ridker et al. report higher levels of SAA in patients with cardiovascular disease together with lower levels of HDL-cholesterol [6], Johnson et al. report a positive correlation between HDL-cholesterol and serum levels of SAA [8].
Our results with lower serum levels of SAA together with lower HDL-cholesterol levels in T allele carriers may seem contradictive in terms of cardiovascular risk. However, a previous study has shown that a high cholesterol efflux capacity from peripheral tissues is associated with a lower risk of coronary artery disease, independent of HDL-cholesterol levels [29]. SAA is an apolipoprotein of HDL [10] and has been suggested to alter the HDL function in the reverse cholesterol transport and change the function of the HDL-particle to become proatherogenic [12]. Interestingly, a previous study has shown that high HDL-levels in combination with high SAA levels was associated with increased all-cause and cardiovascular mortality whereas low SAA levels in combination with high HDL-cholesterol levels were associated with lower all-cause and cardiovascular mortality [30]. In addition, we here also show that T-allele carriers had higher levels of apoE, an apolipoprotein that can be associated with the HDL-particle [31]. Previous studies have shown that that high levels of ApoE-HDL cholesterol are associated with lower cardiovascular risk [32,33,34] and thus, it is possible that the lower SAA levels and higher ApoE levels in the T allele carriers can be cardioprotective regardless of HDL-cholesterol levels.
Hypothetically, there are two possible mechanisms that could explain how the rs11024595 SNP affect cardiovascular risk via serum lipids. We here show the rs11024595 SNP is associated with circulating levels of SAA which can exert a proatherogenic effect of the HDL-particle in a dose-response manner. However, it is also possible that the rs11024595 SNP is in linkage disequilibrium with other polymorphisms in the SAA1 gene that leads to a functional amino acid change in the SAA protein, which in turn can affect the proatherogenic function of the HDL-particle. Future studies are needed to investigate the role of the rs11024595 SNP in SAA binding to the HDL-particle and its effects on cardiovascular disease.
The rs11024595 SNP was also associated with levels of plasma glucose in the SOS study, and it has previously been reported that other polymorphisms in the SAA1 gene are associated with plasma glucose levels [35]. Indeed, several studies have investigated the link between serum amyloid A and impaired glucose homeostasis, which is associated with increased cardiovascular risk [36]. Elevated circulating levels of SAA are associated with insulin resistance [7, 9] and depletion of SAA in antisense oligonucleotide SAA treated mice prevented development of insulin resistance [37]. In addition, recombinant SAA regulates the expression of genes related to glucose homeostasis in adipocytes [38, 39]. However, the results regarding the role of SAA in development of insulin resistance are conflicting [40] and hard to interpret since the endogenous SAA does not exhibit the same proinflammatory characteristics as the recombinant form [41]. Thus, the role of SAA in the development of insulin resistance requires further investigation.
This study has limitations. We could not confirm our results of an association between the rs11024595 SNP and serum levels of HDL cholesterol or plasma glucose observed in the SOS study in the SibPair study. This could be due to lack of power, with fewer study participants and additional adjustment for non-independence among related individuals in the mixed model analyses. Another limitation of this study is that the human SAA immunoassay kit used in this study was not able to distinguish between the different SAA protein isoforms. SAA1 and SAA2 share an extremely high sequence homology and in the circulation these forms represent acute phase SAA [14]. Hence, although SAA1 is the dominating SAA protein in the circulation [42], the higher levels of SAA detected in the rs11024595 C allele carriers may partly be due to SAA2.
Conclusions
In conclusion, we here show that the T allele carriers of the rs11024595 SNP display lower levels of circulating SAA, which according to previous studies may have a cardioprotective effect, but at the same time also display a worse metabolic profile with dyslipidemia. How these findings relate to cardiovascular risk remains unclear and whether allelic variation in the rs11024595 SNP is associated with differences in risk of cardiovascular event require further studies.
Availability of data and materials
The data is subject to legal restrictions according to national legislation. Confidentiality regarding personal information in studies is regulated in the Public Access to Information and Secrecy Act (SFS 2009:400), OSL. There is a possibility to apply to get access to public documents that an authority holds. In this case, the University of Gothenburg is the specific authority that holds the documents. A request to get access to public documents can be rejected or granted with reservations. If the authority refuses to disclose the documents the applicant is entitled to get a written decision that can be appealed to the administrative court of appeal.
Contact person, data inquiries from fellow researchers: Jan Borén, Professor, Prefect; Head of Institute of Medicine, the Sahlgrenska Academy, University of Gothenburg jan.boren@wlab.gu.se.
Abbreviations
- ApoA1:
-
Apolipoprotein A1
- ApoB:
-
Apolipoprotein B
- ApoE:
-
Apolipoprotein E
- BMI:
-
Body Mass Index
- CTCFL:
-
CCCTC-binding factor like
- HDL:
-
High density lipoprotein
- HOMA-IR:
-
Homeostatic model assessment for insulin resistance
- ISNM1:
-
INSM transcriptional repressor 1
- IL-1:
-
Interleukin 1
- IL- 6:
-
Interleukin 6
- LDL:
-
Low density lipoprotein
- SAA:
-
Serum amyloid A
- SNP:
-
Single nucleotide polymorphism
- SOS:
-
Swedish Obese Subjects
- VLDL:
-
Very low density lipoprotein
- ZNF610:
-
Zinc finger protein 610
References
Wilson PW, D’Agostino RB, Levy D, Belanger AM, Silbershatz H, Kannel WB. Prediction of coronary heart disease using risk factor categories. Circulation. 1998;97(18):1837-47. Epub 1998/05/29.
Prospective Studies Collaboration, Whitlock G, Lewington S, Sherliker P, Clarke R, Emberson J, et al. Body-mass index and cause-specific mortality in 900 000 adults: collaborative analyses of 57 prospective studies. Lancet. 2009;373(9669):1083–96. Epub 2009/03/18.
Tirosh A, Shai I, Afek A, Dubnov-Raz G, Ayalon N, Gordon B, et al. Adolescent BMI trajectory and risk of diabetes versus coronary disease. N Engl J Med. 2011;364(14):1315-25. Epub 2011/04/08.
Aday AW, Ridker PM. Targeting Residual Inflammatory Risk: A Shifting Paradigm for Atherosclerotic Disease. Front Cardiovasc Med. 2019;6:16. Epub 2019/03/16.
Fyfe AI, Rothenberg LS, DeBeer FC, Cantor RM, Rotter JI, Lusis AJ. Association between serum amyloid A proteins and coronary artery disease: evidence from two distinct arteriosclerotic processes. Circulation. 1997;4(9):2914–9. 96.
Ridker PM, Hennekens CH, Buring JE, Rifai N. C-reactive protein and other markers of inflammation in the prediction of cardiovascular disease in women. N Engl J Med. 2000;342(12):836–43. Epub 2000/03/25.
Sjoholm K, Lundgren M, Olsson M, Eriksson JW. Association of serum amyloid A levels with adipocyte size and serum levels of adipokines: differences between men and women. Cytokine. 2009;48(3):260–6. Epub 2009/09/18.
Johnson BD, Kip KE, Marroquin OC, Ridker PM, Kelsey SF, Shaw LJ, et al. Serum amyloid A as a predictor of coronary artery disease and cardiovascular outcome in women: the National Heart, Lung, and Blood Institute-Sponsored Women’s Ischemia Syndrome Evaluation (WISE). Circulation. 2004;17(6):726–32. 109. Epub 2004/02/19.
Jylhava J, Haarala A, Eklund C, Pertovaara M, Kahonen M, Hutri-Kahonen N, et al. Serum amyloid A is independently associated with metabolic risk factors but not with early atherosclerosis: the Cardiovascular Risk in Young Finns Study. J Intern Med. 2009;266(3):286–95. Epub 2009/08/26.
Ji A, Wang X, Noffsinger VP, Jennings D, de Beer MC, de Beer FC, et al. Serum amyloid A is not incorporated into HDL during HDL biogenesis. J Lipid Res. 2020;61(3):328–37. Epub 2020/01/10.
Wilson PG, Thompson JC, Shridas P, McNamara PJ, de Beer MC, de Beer FC, et al. Serum Amyloid A Is an Exchangeable Apolipoprotein. Arterioscler Thromb Vasc Biol. 2018;38(8):1890–900. Epub 2018/07/07.
Shah AS, Tan L, Long JL, Davidson WS. Proteomic diversity of high density lipoproteins: our emerging understanding of its importance in lipid transport and beyond. J Lipid Res. 2013;54(10):2575–85. Epub 2013/02/26.
Ahlin S, Olsson M, Wilhelmson AS, Skalen K, Boren J, Carlsson LM, et al. Adipose tissue-derived human serum amyloid a does not affect atherosclerotic lesion area in hSAA1+/-/ApoE-/- mice. PLoS ONE. 2014;9(4):e95468. Epub 2014/04/23.
Uhlar CM, Whitehead AS. Serum amyloid A, the major vertebrate acute-phase reactant. Eur J Biochem. 1999;265(2):501–23.
Sjoholm K, Palming J, Olofsson LE, Gummesson A, Svensson PA, Lystig TC, et al. A microarray search for genes predominantly expressed in human omental adipocytes: adipose tissue as a major production site of serum amyloid A. J Clin Endocrinol Metab. 2005;90(4):2233–9.
Poitou C, Viguerie N, Cancello R, De Matteis R, Cinti S, Stich V, et al. Serum amyloid A: production by human white adipocyte and regulation by obesity and nutrition. Diabetologia. 2005;48(3):519–28. Epub 2005/02/25.
Betts JC, Cheshire JK, Akira S, Kishimoto T, Woo P. The role of NF-kappa B and NF-IL6 transactivating factors in the synergistic activation of human serum amyloid A gene expression by interleukin-1 and interleukin-6. J Biol Chem. 1993;05(34):25624–31. 268.
Jensen LE, Whitehead AS. Regulation of serum amyloid A protein expression during the acute-phase response. Biochem J. 1998;334(Pt 3):489–503.
Edbrooke MR, Burt DW, Cheshire JK, Woo P. Identification of cis-acting sequences responsible for phorbol ester induction of human serum amyloid A gene expression via a nuclear factor kappaB-like transcription factor. Molecular and cellular biology. 1989;9(5):1908–16.
Ray A, Ray BK. Isolation and functional characterization of cDNA of serum amyloid A-activating factor that binds to the serum amyloid A promoter. Mol Cell biology. 1998;18(12):7327–35.
Moriguchi M, Terai C, Kaneko H, Koseki Y, Kajiyama H, Uesato M, et al. A novel single-nucleotide polymorphism at the 5’-flanking region of SAA1 associated with risk of type AA amyloidosis secondary to rheumatoid arthritis. Arthritis Rheum. 2001;44(6):1266–72.
Sjostrom L. Review of the key results from the Swedish Obese Subjects (SOS) trial - a prospective controlled intervention study of bariatric surgery. J Intern Med. 2013;273(3):219–34.
Ahlin S, Sjoholm K, Jacobson P, Andersson-Assarsson JC, Walley A, Tordjman J, et al. Macrophage Gene Expression in Adipose Tissue is Associated with Insulin Sensitivity and Serum Lipid Levels Independent of Obesity. Obesity (Silver Spring). 2013;21(12):E571-6. Epub 2013/06/13.
Castro-Mondragon JA, Riudavets-Puig R, Rauluseviciute I, Lemma RB, Turchi L, Blanc-Mathieu R, et al. JASPAR 2022: the 9th release of the open-access database of transcription factor binding profiles. Nucleic Acids Res. 2022;50(D1):D165-D73. Epub 2021/12/02.
Moriguchi M, Kaneko H, Terai C, Koseki Y, Kajiyama H, Inada S, et al. Relative transcriptional activities of SAA1 promoters polymorphic at position – 13(T/C): potential association between increased transcription and amyloidosis. Amyloid. 2005;12(1):26–32. Epub 2005/08/04.
Yamada T, Wada A. Slower clearance of human SAA1.5 in mice: implications for allele specific variation of SAA concentration in human. Amyloid. 2003;10(3):147-50. Epub 2003/12/03.
Emerging Risk Factors Collaboration, Di Angelantonio E, Sarwar N, Perry P, Kaptoge S, Ray KK, et al. Major lipids, apolipoproteins, and risk of vascular disease. JAMA. 2009;302(18):1993-2000. Epub 2009/11/12.
Birner-Gruenberger R, Schittmayer M, Holzer M, Marsche G. Understanding high-density lipoprotein function in disease: recent advances in proteomics unravel the complexity of its composition and biology. Prog Lipid Res. 2014;56:36–46. Epub 2014/08/12.
Rohatgi A, Khera A, Berry JD, Givens EG, Ayers CR, Wedin KE, et al. HDL cholesterol efflux capacity and incident cardiovascular events. N Engl J Med. 2014;18(25):2383–93. 371. Epub 2014/11/19.
Zewinger S, Drechsler C, Kleber ME, Dressel A, Riffel J, Triem S, et al. Serum amyloid A: high-density lipoproteins interaction and cardiovascular risk. Eur Heart J. 2015;36(43):3007-16. Epub 2015/08/08.
Getz GS, Reardon CA. Apoprotein E as a lipid transport and signaling protein in the blood, liver, and artery wall. J Lipid Res. 2009;50 Suppl:S156-61. Epub 2008/11/18.
Sorokin AV, Patel N, Abdelrahman KM, Ling C, Reimund M, Graziano G, et al. Complex association of apolipoprotein E-containing HDL with coronary artery disease burden in cardiovascular disease. JCI Insight. 2022. Epub 2022/04/08.
Qi Y, Liu J, Wang W, Wang M, Zhao F, Sun J, et al. Apolipoprotein E-containing high-density lipoprotein (HDL) modifies the impact of cholesterol-overloaded HDL on incident coronary heart disease risk: A community-based cohort study. J Clin Lipidol. 2018;12(1):89-98e2. Epub 2017/11/14.
Morton AM, Koch M, Mendivil CO, Furtado JD, Tjonneland A, Overvad K, et al. Apolipoproteins E and CIII interact to regulate HDL metabolism and coronary heart disease risk. JCI Insight. 2018;3(4). Epub 2018/02/23.
Xie X, Ma YT, Yang YN, Li XM, Zheng YY, Fu ZY, et al. SAA1 genetic polymorphisms are associated with plasma glucose concentration in non-diabetic subjects. Clin Chem Lab Med. 2013;51(12):2331–4. Epub 2013/08/14.
Park C, Guallar E, Linton JA, Lee DC, Jang Y, Son DK, et al. Fasting glucose level and the risk of incident atherosclerotic cardiovascular diseases. Diabetes care. 2013;36(7):1988–93. Epub 2013/02/14.
de Oliveira EM, Ascar TP, Silva JC, Sandri S, Migliorini S, Fock RA, et al. Serum amyloid A links endotoxaemia to weight gain and insulin resistance in mice. Diabetologia. 2016;59(8):1760-8. Epub 2016/04/30.
Scheja L, Heese B, Zitzer H, Michael MD, Siesky AM, Pospisil H, et al. Acute-phase serum amyloid A as a marker of insulin resistance in mice. Exp Diabetes Res. 2008;2008:230837. Epub 2008/06/28.
Ye XY, Xue YM, Sha JP, Li CZ, Zhen ZJ. Serum amyloid A attenuates cellular insulin sensitivity by increasing JNK activity in 3T3-L1 adipocytes. J Endocrinol Invest. 2009;32(7):568-75. Epub 2009/05/29.
Ahlin S, Olsson M, Olsson B, Svensson PA, Sjoholm K. No Evidence for a Role of Adipose Tissue-Derived Serum Amyloid A in the Development of Insulin Resistance or Obesity-Related Inflammation in hSAA1(+/-) Transgenic Mice. PLoS ONE. 2013;8(8):e72204.
Christenson K, Bjorkman L, Ahlin S, Olsson M, Sjoholm K, Karlsson A, et al. Endogenous Acute Phase Serum Amyloid A Lacks Pro-Inflammatory Activity, Contrasting the Two Recombinant Variants That Activate Human Neutrophils through Different Receptors. Front Immunol. 2013;4:92.
Xu Y, Yamada T, Satoh T, Okuda Y. Measurement of serum amyloid A1 (SAA1), a major isotype of acute phase SAA. Clin Chem Lab Med. 2006;44(1):59–63. Epub 2005/12/27.
Acknowledgements
We would like to thank all the study participants and the surgical departments and primary health care centers involved in the studies.
Funding
Open access funding provided by University of Gothenburg. The study was financed by grants from the Swedish state under the agreement between the Swedish government and the county councils, the ALF-agreement (ALFGBG-717891), the Health & Medical Care Committee of the Region Västra Götaland. (grant no. VGFOUREG-931560 and VGFOUREG-941125), the Swedish research council (grant no. 2016 − 00522), the Swedish Diabetes foundation (grant no. 2019 − 417) and the Magnus Bergvall Foundation (grant no 2019–03489). The funders had no role in study design, data collection and analysis, decision to publish, or preparation of the manuscript.
Author information
Authors and Affiliations
Contributions
KS, LMSC, ML and PJ designed the studies. SA, ML, MT, JCAA, KS and PAS performed the genotyping, gene expression analysis and analysis of serum levels of SAA. KS, SA, ML and MP performed the statistical analysis and contributed to the interpretation of the data. SA, ML and KS wrote the manuscript and LMSC, PJ, MT, JCAA, PAS and MP contributed substantially in revising the manuscript. All authors read and approved the final manuscript.
Corresponding author
Ethics declarations
Ethics approval and consent to participate
All study protocols were approved by seven regional ethics committees (Göteborg, Linköping, Lund/Malmö, Stockholm, Umeå, Uppsala and Örebro). All participants gave informed consent to participate. The studies were performed in accordance with the Declaration of Helsinki.
Consent for publication
Not applicable.
Competing interests
The authors declare no conflicts of interest.
Additional information
Publisher’s note
Springer Nature remains neutral with regard to jurisdictional claims in published maps and institutional affiliations.
Supplementary Information
Additional file 1.
Supplementary Material 1
Rights and permissions
Open Access This article is licensed under a Creative Commons Attribution 4.0 International License, which permits use, sharing, adaptation, distribution and reproduction in any medium or format, as long as you give appropriate credit to the original author(s) and the source, provide a link to the Creative Commons licence, and indicate if changes were made. The images or other third party material in this article are included in the article's Creative Commons licence, unless indicated otherwise in a credit line to the material. If material is not included in the article's Creative Commons licence and your intended use is not permitted by statutory regulation or exceeds the permitted use, you will need to obtain permission directly from the copyright holder. To view a copy of this licence, visit http://creativecommons.org/licenses/by/4.0/.
About this article
Cite this article
Ahlin, S., Löfgren, M., Taube, M. et al. A SNP in the 5’ flanking region of the SAA1 gene is associated with serum levels of serum amyloid A and cardiovascular risk factors. transl med commun 7, 12 (2022). https://doi.org/10.1186/s41231-022-00119-3
Received:
Accepted:
Published:
DOI: https://doi.org/10.1186/s41231-022-00119-3