Abstract
Background
Stone pine (Pinus pinea), a drought-resistant species, has significant socio-economic benefits and increasing interest for the establishment of productive plantations in several countries, especially in a climate change context. Monitoring hourly stem diameter variations contributes to the understanding of the tree-growth response to changes in environmental conditions and management. By monitoring the diameter expansion of tree stems, high-resolution band dendrometers were used to study the development of adult trees growing in a semi-arid coastal environment of central Chile under fertilized and non-fertilized soil conditions through the span of a year.
Results
Short cycles (< 21 h) were few in fertilized and non-fertilized trees (6 and 4, respectively), whereas long cycles (> 28 h) occurred at a higher frequency in fertilized trees (16 vs 6). Most of the circadian cycles were regular (24 ± 3 h). The longest cycle duration (59 h) was observed in fertilized trees during spring. In all seasons, each phase of the circadian cycle, especially during the stem diameter increment phase (P3, irreversible growth), started earlier in fertilized than in control trees. P3 duration was significantly longer in fertilized than in control trees in springtime. The maximum shrinkage (P1) was observed in summer for both treatments. Stem diameter increased faster in fertilized than in control trees throughout the year, with the highest accumulation occurring in spring and the lowest in autumn. The daily variability pattern showed lower growth under high temperature across seasons.
Conclusions
This study highlights the importance of fertilization in enhancing stone pine diameter growth. This cultural practice should be further explored to contribute to the mitigation of climate change effects in semi-arid environments.
Similar content being viewed by others
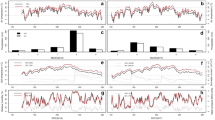
Background
Stone pine (Pinus pinea L.) is a drought-tolerant Mediterranean species whose valued seeds, the pine nuts, have been harvested from wild and cultivated trees for thousands of years (Lim 2012). Significant socio-economic benefits of its cultivation have been reported for Turkey (Eker and Laz 2018), Tunisia (Schröder et al. 2014), Lebanon (Sattout and Faour 2017) and Europe (Awan and Pettenella 2017). The species shows an interesting growth and fruiting potential in Chile (Loewe et al. 2016) and other non-native countries, where there is an increasing interest in establishing productive plantations.
Fertilization has well-known benefits on crop yield and fruit quality of nut-bearing species (Wani et al. 2016; Kumar et al. 2017). In stone pine, fertilization has been found to have positive effects on seedling growth in nursery (Montero et al. 2004) and initial growth after planting (Toca et al. 2014), and in cone production and quality (Calama et al. 2007; Kilci 2011; Freire et al. 2019). Fertilization also improves growth (Freire et al. 2019) and canopy efficiency (Correia et al. 2016). The species is sensitive to deficiency of micronutrients such as boron (Bento and Coutinho 2011) and iron (Malchi and Shenker 2011). Diameter growth was found to be stimulated by fertilization (Loewe-Muñoz et al. 2020); this effect is important due to the correlation between diameter at breast height (DBH, 1.3 m) and cone production (Freire et al. 2019).
However, little is known about stem diameter variability as a response to fertilizer supply at a fine temporal scale in the circadian cycle of stone pine. This information could be useful to provide cropping guidelines, since knowledge on the seasonal circadian cycle dynamics might be important to determine when fertilization will have a more positive effect on enhancing the recovery from the stem shrinkage induced by water deficit, and on boosting irreversible growth.
Stem diameter variations monitored by dendrometers have great potential to capture in situ forest productivity and sensitivity to environmental stress and are useful as early warning to detect stress thresholds in tree vitality and growth (Salomón et al. 2022). The daily dynamics of stem diameter fluctuations involve tree-related properties and external environmental conditions that influence the flow of water within the soil–plant–atmosphere continuum. In fact, an accurate measurement of stem diameter variations (SDVs) could be used to estimate sap flow (Sevanto et al. 2008), especially the stem shrinkage phase (Tian et al. 2019). SDVs result in reversible depletion and replenishment (Steppe et al. 2006) and irreversible diameter growth, with tree water status playing a key role (Zweifel et al. 2006), as observed in Pinus hartwegii Lindl. (Biondi et al. 2005) and in P. sylvestris L. (Zweifel et al. 2006). Thus, hourly stem diameter variation monitoring throughout the day during 1 year would help identify reversible shrinkage and swelling (Downes et al. 1999), and understand the tree’s response to changes in environmental conditions (Deslauriers et al. 2007a; Drew and Downes 2009) and management. Understanding those responses is relevant since drought-exposed forests may be at risk of mortality. Therefore, there is a need to know tree susceptibility to environmental changes to contribute to the species performance in a context of climate change (Schäfer et al. 2019). The species adopts a strategy of drought tolerance and drought avoidance to adapt to semi-arid coastal environment. Balekoglu et al. (2023a) explored the drought stress strategy of P. pinea seedlings, reporting that some provenances hold an isohydric (avoidance) while others an anisohydric (tolerance) behavior.
The information obtained using high-resolution dendrometers is useful for irrigation scheduling (Espadafor et al. 2017), crop growth monitoring (Steppe et al. 2015), site quality assessment and identification of main drivers of stem diameter growth at sub-diurnal resolution (Drew and Downes 2009). However, to date, no information is available on the intra-daily stem diameter variations in stone pine subjected to different management practices that would affect the circadian cycle (consecutive occurrence of shrinkage, recovery and increment phases). This approach may contribute to the understanding of the stone pine circadian clock seasonal variability and the impact of fertilization on this variability. Circadian clocks include self-sustained rhythms that persist despite a lack of external time cues and that remain largely constant under changing temperature conditions, being coupled to photoperiodic responses (McClung 2006), important for several development processes including growth control (Gyllenstrand et al. 2014).
The effect of fertilization on diameter growth was previously reported for horticultural crops (Brun and Tournier 1992) and stone pine (Loewe-Muñoz et al. 2020). Measuring short-term stem diameter changes and coupling them to environmental conditions is a practical approach to the understanding of stem sensitivity to management interventions and of the species-specific patterns (McMahon and Parker 2015; Cocozza et al. 2018; Zalloni et al. 2018).
We explored the scientific question of how fertilization affects the stone pine stem circadian cycle. The objective of the study was to characterize the stem circadian cycle (composed of contraction, expansion and irreversible growth), and to quantify stem cycle classes frequency across seasons in fertilized and non-fertilized adult stone pine trees in a semi-arid coastal environment. Our working hypothesis postulates that nutrient supply increases irreversible diameter growth, contributing to the species performance.
Methods
Study area and experimental trial
Data were collected from a 31-year-old stone pine plantation in Cáhuil, Chile (34° 30′ SL, 71° 59′ WL) established on smooth hills with a sandy-loam and non-salty soil (E.C. 0.06 mmho cm−1), slightly acid (pH = 6.2), with low organic matter content (1.7%), at 125 m a.s.l. The genetic material of measured trees is a mix from Portugal and local sources. The plantation was thinned 20 years after establishment, with 50% of trees being cut; 10 years later, the plantation was pruned and thinned, with 65% being removed and an average density of 285 trees ha−1 being left. At the start of the trial, average size of trees was 5.7 m in height, 16 cm DBH and 3.6 m of crown diameter, reflecting a lower growth than in the dry coast macrozone (Loewe et al. 2015).
The climate in the study area is Mediterranean, characterized by long dry summers and short intense winter precipitation ( Table 1), with Pacific Ocean influence. Dry months, expressed as the sum of all months of the year for which the ratio between rainfall and evapotranspiration is below 0.5, are 8.6 per year. During the study period, negligible amount of rainfall was detected in November, February and May.
The trial to assess the fertilization effects was set up in 2013 and consisted of two treatments, control and fertilization. Plots (25 trees each) were arranged in a randomized complete block design with three replicates. One tree from the center of the plots was selected for hourly monitoring of stem diameter variations, accounting for six trees in total. Average DBH of sampled trees was 16.3 ± 1.2 cm.
Doses and fertilizers were determined according to soil deficiencies identified through soil analyses. Fertilizers were locally applied in two lateral strips at 5 cm depth. In spring 2013, fertilized trees received Novatec N-max (1500 g plant−1), zinc sulfate (20 g plant−1) and borax (60 g plant−1). In the following autumn (2014), and in order to induce nutrient load (Li et al. 2016), trees received a second fertilization with triple super phosphate (350 g plant−1) and carbamide (200 g plant−1). In the studied growing season 2014/2015, spring and autumn fertilizations were repeated with the same products and doses, including macronutrients (nitrogen, phosphorus, potassium, calcium, magnesium and sulphur) and micronutrients (boron, iron and zinc).
Data collection
High-resolution band dendrometers (EMS DLR26A) were used to monitor tree growth continuously. Dendrometers were installed at a height of 1.3 m perpendicular to the slope, in three healthy trees for each treatment. Bark outermost loose layers (periderm) were slightly removed to allow proper mounting and to ensure close contact with the stem. The temperature-compensated electronic band dendrometers have a resolution of < 1 μm (Environmental Measuring Systems, Brno, Czech Republic). Raw data were taken every hour, stored into a data logger memory and downloaded using a converting cable with built-in infrared transceiver USB EMS/IrDA. Dendrometer temperature sensor operates on the range from − 30 to 60 °C.
Stem circumference variations were recorded daily at 1-h intervals (00:00 to 24:00) from June 2014 to May 2015 using the software EMS Mini32. Changes in stem circumferences were converted to stem diameter variations assuming a circular cross-section (Vospernik and Nothdurf 2018). From dendrometers, surrounding air temperature was obtained at 1-h intervals. Main daily air temperatures, precipitation, and potential evapotranspiration were obtained and summarized by season ( Table 1). The distribution of daily mean air temperature and annual precipitation is presented in Fig. 1.
Circadian cycle characterization
To study the stem circadian cycle, the hourly SDVs were accumulated and split into three different phases covering approximately 24 h (Deslauriers et al. 2007b). The contraction phase (phase 1, P1) corresponds to the stem shrinkage and is calculated as the difference between the maximum stem diameter value of the previous cycle and the minimum stem diameter value of the current cycle. The expansion phase (phase 2, P2) represents the stem swelling, and involves the period from the minimum to the maximum previous cycle stem diameter value. The stem diameter increment (phase 3, P3) starts when the accumulated SDV exceeds the previous cycle maximum and lasts until the next maximum (Fig. 2). The maximum cycle stem expansion (TE) corresponds to the difference between the minimum and the maximum stem diameter value of the cycle (sum of P2 and P3).
Circadian cycle of stem growth studied from hourly SDVs in stone pine by phase. Each cycle is divided into three phases: contraction (P1, black), expansion (P2, grey) and stem diameter increment (P3, white). Total expansion (TE) corresponds to the maximum stem expansion of the cycle (P2 + P3). A short cycle lasts 18 h, a regular cycle lasts close to 24 h, and a long cycle up to 2.5 days
Time of onset was determined as the start of each phase for all circadian cycles by using treatment average SDV series. The mean phase duration was calculated after classifying the cycles into short, regular and long duration: cycles lasting less than 21 h, 24 ± 3 h, and more than 28 h, respectively (Deslauriers et al. 2007a). To obtain the representation of SDVs throughout the year, a window of 1 month was studied in each season; seasonal mean values were calculated using all days of August (winter), November (spring), February (summer) and May (autumn). The monthly frequency of cycle types was analyzed to understand cycle distribution.
Data analyses
Both components of the circadian stem cycle study (phase duration and SDVs) were analyzed through mixed ANOVA models using the stem cycle as the unit of analysis. To compare phase duration between treatments, the ANOVA model included fixed effects of treatment, phase, season and their interactions, and a random cycle effect. Residuals from the phase duration model were normally distributed. Differences in the frequency distribution of the cycle class (short, regular and long) were χ2 tested using marginal tables (or across seasons). All seasons were considered together in the χ2 analysis, since the number of non-regular cycles was low.
For the second circadian stem cycle component, SDVs were studied in cycles of regular duration. The ANOVA models used to analyze the phases of contraction, expansion and stem diameter increment involved fixed effects of treatment, season and their interactions, and a random cycle effect. These parameter distributions were asymmetric; thus, data were transformed by the square root of the value + 1.5, as in Deslauriers et al. (2007a). The LSD test (α = 0.05) was used for mean comparisons.
The empirical distribution function of SDV of cycles with data from all dendrometers during the 365 days was constructed to provide a proxy of how stem diameter accumulated throughout the year by treatment. Then, the cycle’s SDVs of fertilized and control trees were correlated with hourly air temperature using the Spearman rank correlation coefficient. Statistical analyses were performed using the software Infostat (Di Rienzo et al. 2023) and its interface with the software R (R Core Team 2020).
Results
Circadian cycle timing and duration
The time of onset and duration of each phase are presented in Table 2; this table also includes the number of cycles in each circadian cycle class (short, regular and long) in 1 month by season. The number of short cycles (< 21 h) was low in both fertilized and non-fertilized trees (in total, 6 and 4 in the four studied months, respectively). Long cycles occurred at a higher frequency in fertilized than in control trees (16 vs. 6 during the four studied months). Most of the circadian cycles were regular (105 for control trees and 85 for fertilized trees during the four studied months) and lasted on average 23:57 h. The average longest cycle duration (59:00 h) was observed in spring for fertilized trees (Table 2). In all seasons, on average onset occurred earlier in fertilized trees than in the control ones, especially for P3.
Treatment × season × phase interactions were non-significant (p = 0.08) for phase length. Second-order interactions of season × phase and treatment × phase were statistically significant (p < 0.01), but treatment × season was non-significant (p = 0.22). The duration of the phases of the regular circadian cycles is presented in Table 3. The contraction phase was not affected by fertilization, being similar in both treatments (p > 0.05) in all seasons. Fertilization showed a significant effect on the expansion phase duration, which was shorter than in control trees except in autumn. In all seasons, on average, the stem diameter increment phase lasted longer in fertilized than in control trees, with significant differences only in spring.
Stem variations during a circadian cycle
At a month scale, the number of short and long cycles in stone pine was low, whereas between 19 and 28 regular cycles per month were observed; therefore, only regular cycles were used to study stem hourly variations during a circadian cycle. Table 4 shows the mean SDVs of each cycle phase by treatment and season. For the contraction phase (P1), the treatment × season interaction was non-significant (p = 0.43); the treatment effect was non-significant (p = 0.53), and the season effect was statistically significant (p < 0.01). For the stem diameter increment phase (P3), the treatment × season interaction was significant (p = 0.01), as well as the treatment effect (p < 0.01) and the season effect (p < 0.01). For the total expansion (P2 + P3), the treatment × season interaction was significant (p < 0.01), as well as the treatment effect (p < 0.01) and the season effect (p < 0.01).
For P1, the maximum shrinkage (highest contraction of the cycle) was observed in summer for both treatments (Table 4). The total expansion mean was statistically different between treatments in winter and spring (4.2 and 2.3 times, respectively; Table 4). P3 showed statistically higher mean values in fertilized trees than in control ones in winter and spring (Table 4). The smallest SDVs of P3 occurred in fertilized trees in autumn and in control trees in winter. The circadian cycle class frequency was statistically significant between treatments across seasons (χ2 = 6.77, p = 0.03) (Fig. 3).
The empirical distribution function from raw SDV data shows that stem diameter accumulated faster in fertilized than in control trees throughout the year (Fig. 4). Accumulated diameter growth during the study year was on average 14.9 mm in fertilized trees and 5.3 mm in control trees (Fig. 4). Time series of SDVs of the mean circadian cycle during 1 month of spring, summer, autumn and winter are shown for each treatment in Fig. 5, where P3 is highlighted. The highest and the lowest accumulated SDVs were recorded in spring and autumn, respectively, for both fertilized and control trees.
Time series of circadian cycles by season and treatment (Fertilization, upper line; control, bottom line). The stem cycle is divided into three phases: contraction (solid black), expansion (grey), and diameter increment (red). One month of winter (August), spring (November), summer (February) and autumn (May) was studied
Figure 6 presents the hourly SDV, which shows the intra-cycle stem variation, and air temperature, which was simultaneously recorded by dendrometers during 1 month in each season. A Spearman correlation was found between treatments and air temperature (ρ = − 0.60, p < 0.01 for fertilized trees and ρ = − 0.43, p < 0.01 for control trees) throughout the year and in every season (Fig. 6). A significant correlation between series of control and fertilized trees was also observed across seasons (ρ = 0.74, p < 0.01). The observed temporal variability pattern shows low growth under high daily temperatures in all seasons. During the fastest growth period (spring), the highest SDV values of the circadian cycle were found under fertilization treatment. Growth of fertilized trees stopped at noon and resumed at 16:00 pm, whereas in control trees, growth also stopped at noon but resumed at 20:00 pm. Irreversible growth on average started with air temperature over 11.8 °C in control trees and 10.9 °C in fertilized trees.
Time series of hourly diameter variations and concomitant air temperature (red) by season and treatment. Fertilized trees (F): solid, control trees (C): dashed. Correlation values among SDVs and temperature series are reported in the embedded tables. One month of winter (August), spring (November), summer (February) and autumn (May) was studied
Discussion
Characterizing stem growth at an intra-daily scale is useful to understand the effects of management on growth. Indeed, the SDV distribution and frequency should be interpreted as a function of the physiological status of trees, which changes over the seasons (Deslauriers et al. 2007a). Seasonal SDVs can help elucidate the relationship between tree growth and seasonal climatic conditions.
The importance of characterizing the duration of the circadian cycle phases has been highlighted for other species (Deslauriers et al. 2007b). In our study, in both treatments most of the circadian cycles lasted around 24 h (regular), meaning that circadian rhythm, temperature and light are intertwined in trees, whereas the number of short and long cycles was low. Long cycles were 2.7 times more frequent in fertilized than in control trees, indicating a longer duration of irreversible growth in managed trees; long cycle duration indicates a decoupling of growth with daily changes in temperature or light. P1, the daily shrinkage related to changes in tree moisture content, typically occurred in the afternoon in both treatments, when transpiration is higher than root water uptake (Tian et al. 2019) and stems lose water from elastic tissues (bark, cambium and immature xylem) (Zweifel et al. 2014). P1 also included the night in control trees in winter, and mornings in fertilized trees in summer. The longest P1 lasted 17 h in control trees and occurred in winter, whereas in fertilized trees it lasted 18 h and occurred in summer and autumn; these maximum durations are much shorter than the maximum P1 duration reported for Cedrus libani A. Rich. and Juniperus excelsa M. Bieb., which ranged from single days to several weeks (Güney et al. 2020). Juniperus species (Douaihy et al. 2013) and Cedrus libani can adapt to extremely harsh environmental conditions, including drought (Messinger et al. 2015). P. pinea, an isohydric species able to tolerate drought through different physiological mechanisms, including root mortality, stomata control and biomass allocation (Oliveras et al. 2003), showed a best performance under more intense drought that these well-known drought-resistant species. Swelling (P2), which reflects the daily water uptake that takes place after P1, occurred in nights and mornings in winter and autumn, and mostly in nights of spring and summer, both in fertilized and control trees.
The onset of the phases of the circadian cycle differed between treatments, occurring earlier in fertilized trees than in the control ones, especially for P3 (over 2.5 h earlier). Thus, fertilization reduced the influence of environmental control on the phase onset reported by Oberhuber et al. (2014), in particular by air temperature, throughout the year. In a study on Pinus cembra, the onset of P1 and P2 in non-fertilized trees was similar to the one found in this work, and onset of P3 in summer was earlier than our values, with longer duration of the cycles and of each of the three phases (Deslauriers et al. 2007b). The asynchronous end of P3 observed between treatments suggests dependence on climatic variables (Swidrak et al. 2011), as well as an environmental control.
Phase duration could be highly impacted by climate variability, since shrinking and swelling are caused by the tree transpiration and sap flow (Perämäki et al. 2001; Tian et al. 2019). Regarding the seasonal variations in SDV patterns, fertilization reduced P1 length in summer by over 2 h and P2 length in all seasons, except in autumn. Fertilization increased the duration of P3 in spring, influencing the effective irreversible growth duration. These positive effects of fertilization on stem diameter growth are consistent with previous results on the effect of P and P + N supply on P. sylvestris and Picea abies (Niederberger et al. 2019); in adult stone pine plantations, N was found to be the most limiting element (Rapp et al. 1979). Different species show varied responses to water deficit. In fact, Picea abies and P. cembra show a water saving behavior while Larix decidua maintain a relatively high transpiration even under moderate water deficit (Anfodillo et al. 1998). In particular, P. abies was reported to have a limited capacity to restore internal water reserves (Knüver et al. 2022). In our study, in untreated trees, P2 lasted 11:14 h in summer, a similar duration to the one reported for L. decidua, P. abies, Pinus cembra (Deslauriers et al. 2007b), and Abies balsamea (Deslauriers et al. 2003) in wet spring–summer, indicating a remarkable recovery of stone pine even under arid conditions.
Despite rainfall and air temperature seasonal variations, interestingly, our study showed from winter to summer that fertilization contributed to a faster recovery from the stem shrinkage induced by water deficit than observed in control trees (gains of 4:29 in winter, 3:12 h in spring and 2:40 h in summer); in spring, the fertilization impact led to a recovery phase shorter than the previous shrinkage. These results are in agreement with Balekoglu et al. (2023b) who found that in stone pine seedlings, a low C/N ratio in stems have superior resistance and recovery in summer. In the same season, in P. taiwanensis under high temperatures and low rainfall, the strong transpiration amplified the magnitude of contraction within tree stems; thus, the refill of the stem hydraulic capacitance was restricted due to the low soil water availability, resulting in the shrinkage of stems and lower growth and shorter duration of stem increments (Liu et al. 2018). The maximum duration of P2 indicates that fertilization induced a faster recovery (18.2% in winter, 25.0% in spring, and 6.7% in summer). This valuable finding agrees with results obtained by several authors that point out that a sufficient nutrient supply can increase the water-use efficiency during droughts and enable a faster recovery afterwards (Gessler et al. 2017). In particular, Balekoglu et al. (2023b) found that N availability increased stone pine recovery in summer; Chambi Legoas et al. (2023) reported positive effects of K fertilization on Eucalyptus grandis for growth recovery following drought; and Wang et al. (2022) concluded that fertilization can alleviate the negative effects of extreme drought on survival and recovery growth of Quercus pubescens saplings.
In conifers, P3 is determined by soil water availability and atmospheric vapor pressure deficit (VPD) (Zweifel et al. 2005). In summer and autumn, P3 occurred mostly during the mornings in control trees, and during the nights and mornings in fertilized trees. In winter, in control trees P3 started in the morning and ended at night, whereas in fertilized trees it started during the night and finished the next afternoon and lasted three more hours. In spring, the fastest growth period when temperature is favorable for growth, P3 occurred during nights and mornings in control trees, a longer period than reported for P. palustris, P. radiata and P. resinosa (Mencuccini et al. 2017), and during night, morning and afternoon in fertilized trees, a longer period than in the control trees. Thus, fertilization increased P3 duration, in agreement with our hypothesis.
The fastest stem diameter growth was observed in spring, followed by winter; in summer growth was very low, as reported for most species (Sánchez-Costa et al. 2015), due to the harsh environmental conditions during the summer drought (Liu et al. 2018). In autumn, it was negligible, probably due to low temperatures and water deficit (Aldea et al. 2021), and to the photoperiod impact, with shorter days inducing growth cessation (Gyllenstrand et al. 2014). During dry periods, even a low rainfall event (< 15 mm day−1) can alleviate water deficit, inducing an enlargement of the xylem cells (Zweifel et al. 2006). Mediterranean climate usually leads to a bimodal growth pattern with peaks before and after summer (Aldea et al. 2018), but in our study, trees grew before and during the dry period, probably supported by the humidity from the Pacific Ocean. In fact, the wetting of leaves with fog and dew positively affects plant water balance, without significantly increasing soil wetness (Limm et al. 2009). The studied stone pine trees grew all year round, except in autumn; thus, the growth period was much longer than that reported for P. sylvestris trees growing at a xeric site with higher annual rainfall, which only grew during a short period in spring (Oberhuber 2017).
Fertilization effect on irreversible growth was greatest in spring. Stem growth has been enhanced by macronutrients (Ferrer et al. 2004) and micronutrients (Schlatter and Gerding 1984a, b) availability in several pines. Species-specific responses to the nitrogen supplementation on xylem growth have been reported in Quercus species (Yu et al. 2019). The strongest effect of fertilization was observed in spring, when growth rates are highest (Gruber et al. 2010); this result indicates that fertilization is a useful silvicultural tool to cope with the limiting environmental conditions prevailing in the study area, e.g., drought in spring combined with a limited soil water holding capacity and nutrient deficiency. The occurrence of most irreversible growth during dry periods could be attributed to water reserves stored in the trees (Oberhuber 2017; Szymczak et al. 2020) or to the ability of stone pine to tap water from deep soil layers (Brito et al. 2017); if this ability is limited, severe drought may reduce growth and cause mortality. In fact, Schönbeck (2019) concluded that a low-intensity drought stress could be offset by increased nutrient availability, with fertilization improving the metabolism and functioning of the roots, and C allocation to belowground tissues, as also reported by Balekoglu et al. (2023b).
Understanding how daily stem growth is affected by environmental conditions provides a useful approach to the study of the variations in broadleaves (Deslauriers at el. 2007a) and Pinus sylvestris (Thaabet et al. 2009) tree growth. In fact, an increase in air temperature shortened both duration and SDVs in both treatments and all seasons. This result partially agrees with findings of Deslauriers et al. (2007b), who reported its effect only on shortening duration, and disagrees with those of Oberhuber and Gruber (2010), who stated that air temperature had no influence on stem increment. The observed negative effect found in this study is probably due to the impact of transpiration on diameter growth (Tardif et al. 2001); however, contrasting effects of temperature on SDVs may occur in the circadian cycle phases, possibly associated with optimum temperature (Oladi et al. 2017) for the different physiological processes.
Temperature is a determinant of growth that was found to influence cell production in several European conifers (Rossi 2003), a positive correlation with stem diameter contraction was reported in the native Fitzroya cupressoides (Urrutia-Jalabert et al. 2015). Thus, growth–temperature relationships are interesting to understand growth processes and environmental drivers. Negative correlations were found in our study between SDVs and air temperature, with high temperatures reducing stem growth duration and magnitude, probably through a metabolic alteration, especially changes in leaf transpiration (Oberhuber et al. 2014). In fact, temperature above a certain threshold (16 to 21 °C) (Beedlow et al. 2013) increases photorespiration (Lewis et al. 1999), inducing a reduction in net photosynthesis, and consequently resulting in a negative effect on stem diameter growth.
The growth–air temperature correlations found at an intra-daily scale highlight the relevance of water availability on circadian cycles. The negative correlations evidenced between stem diameter increments and mean air temperature in both treatments and all seasons, except in winter, for control trees, disagree with results reported by Akkemik (2000). These negative correlations can be attributed to the water deficit caused by warm temperatures (Bachtobji-Bouachir et al. 2017), which drives changes in leaf transpiration (Oberhuber et al. 2014); transpiration is lower in P. pinea than in Citrus sinensis and Olea europaea (Rana and Ferrara 2019).
The effect of rainfall on stem growth and circadian cycles has been repeatedly reported as important (Tardif et al. 2001; Deslauriers et al. 2003, 2007b; Oberhuber and Gruber 2010; Oberhuber 2017; Camarero and Rubio-Cuadrado 2020; Ma et al. 2021). However, we did not study this variable because no rainfall occurred in the studied months of spring, summer and autumn, and only few events were recorded in winter (see Fig. 1). In fact, the observed ability to grow during dry periods is an indication of the species’ drought tolerance (Güney et al. 2020).
Since stem growth is influenced by climatic characteristics across years (Oberhuber and Gruber 2010; Liu et al. 2018), long-term studies including the combined action of air temperature and other environmental factors should be conducted to understand the stone pine circadian cycles inter-annual variability.
Overall, the results suggest that stone pine has seasonal growth, which would be driven mainly by temperature. Interestingly, stone pine maintained a low continuous diametric growth even during the dry summer period, confirming its exceptional drought tolerance. Our results indicate a high adaption of stone pine to current climate conditions in coastal central Chile, tolerating prolonged droughts during spring and summer. Therefore, the species would hold a promising potential for establishing productive plantations under a changing climate, which should be confirmed in future studies. This study provides additional evidence about the effects of climatic factors on stem growth, and on the importance of the availability of nutrients in the potential enhancement of irreversible growth, the most important phase of the circadian cycle from a productive point of view. Differences in nutrient acquisition may drive differential physiological processes that modify the circadian cycle of stone pine throughout the seasons. The capacity of stone pine to adjust cambial activity to environmental conditions represents an interesting strategy under climate change.
Considering the projected effects of climate change, especially in Mediterranean regions, which may reduce the species’ survival capacity, studies including tree mortality are needed (Güney et al. 2020) especially under drought conditions. This knowledge is important to understand the vulnerability of productive plantations and to estimate their carbon sequestration capacity. Long-term monitoring of the fertilization effects on stem growth can contribute to the definition of climate-adaptive silvicultural strategies to promote stone pine adaptation to challenging environmental conditions, addressing limitations of this study, such as the inclusion of only one plantation, and evaluating more trees and during a longer span time to determine long-term treatment and environmental effects, such as concurrent drought.
Conclusions
The intra-daily monitoring of stem diameter variations allowed us to explore the stone pine stem circadian cycle in detail and to quantify the impact of fertilization on diameter growth. Thus, we were able to elucidate tree responses to environmental conditions. Fertilized trees exhibited a faster recovery from the shrinkage phase and a higher irreversible stem diameter growth than control trees. SDVs can be used to assess the environmental sensitivity of forest trees to climate and management practices; this information is useful to make climate-adapted forest management decisions. Data obtained on a daily basis and with a high temporal resolution revealed a positive significant silvicultural effect of fertilization on stem diameter growth and its distribution and duration. Fertilization changed the diel pattern of growth. Indeed, in control trees irreversible growth was concentrated in nights and mornings of the fastest growth period (spring), whereas in fertilized trees, growth also occurred during the day. Thus, fertilization extended the growth period.
Irreversible diameter growth was limited by climatic stress (dry winter and hot summer), and absent in autumn, probably due to temperature above an optimum threshold and drought stress in mid-late growing season. Superior irreversible stem diameter growth was observed in spring, when temperature is favorable for growth, being fertilization effective to further increase it. The seasonal shifts detected in the stone pine circadian cycle suggest the importance of long-term monitoring growth at a daily scale, to forecast growth responses to climate variability that could be useful to make climate-adapted forest management decisions.
Availability of data and materials
The datasets supporting the conclusions of this article are available from the corresponding author on reasonable request.
Abbreviations
- P1:
-
Stem diameter contraction phase, phase 1
- P2:
-
Stem diameter expansion phase, phase 2
- P3:
-
Stem diameter increment phase, phase 3
- TE:
-
The maximum cycle stem expansion
- DBH:
-
Diameter at breast height (1.3 m)
- SDV:
-
Stem diameter variation
- VPD:
-
Vapor pressure deficit
- PET:
-
Potential evapotranspiration
References
Akkemik Ü (2000) Dendroclimatology of umbrella pine (Pinus pinea L.) in Istanbul, Turkey. Tree-Ring Bull 56:17–20
Aldea J, Bravo F, Vázquez-Piqué J, Rubio-Cuadrado A, del Río M (2018) Species-specific weather response in the daily stem variation cycles of Mediterranean pine-oak mixed stands. Agric For Meteorol 256–257:220–230. https://doi.org/10.1016/j.agrformet.2018.03.013
Aldea J, Bravo F, Vázquez-Piqué J, Ruíz-Peinado R, del Río M (2021) Differences in stem radial variation between Pinus pinaster Ait. and Quercus pyrenaica Willd. may release inter-specific competition. For Ecol Manage 481:e118779. https://doi.org/10.1016/j.foreco.2020.118779
Anfodillo T, Rento S, Carraro V, Furlanetto L, Urbinati C, Carrer M (1998) Tree water relations and climatic variations at the alpine timberline: seasonal changes of sap flux and xylem water potential in Larix decidua Miller, Picea abies (L.) Karst. and Pinus cembra L. Ann Des Sci For 55:159–172. https://doi.org/10.1051/forest:19980110
Awan H, Pettenella D (2017) Pine nuts: a review of recent sanitary conditions and market development. Forests 8(10):e367. https://doi.org/10.3390/f8100367
Bachtobji-Bouachir B, Khorchani A, Guibal F, El Aouni MH, Khaldi A (2017) Dendroecological study of Pinus halepensis and Pinus pinea in northeast coastal dunes in Tunisia according to distance from the shoreline and dieback intensity. Dendrochronologia 45:62–72. https://doi.org/10.1016/J.DENDRO.2017.06.008
Balekoglu S, Caliskan S, Dirik H, Rosner S (2023a) Response to drought stress differs among Pinus pinea provenances. For Ecol Manage 531:e120779. https://doi.org/10.1016/j.foreco.2023.120779
Balekoglu S, Caliskan S, Makineci E, Dirik H (2023b) An experimental assessment of carbon and nitrogen allocation in Pinus pinea populations under drought stress and rewatering treatment. Environ Exp Bot 210:e105334. https://doi.org/10.1016/j.envexpbot.2023.105334
Beedlow PA, Lee EH, Tingey DT, Waschmann RS, Burdick CA (2013) The importance of seasonal temperature and moisture patterns on growth of Douglas-fir in western Oregon, USA. Agric For Meteorol 169:174–185. https://doi.org/10.1016/j.agrformet.2012.10.010
Bento J, Coutinho J (2011) Boron deficiency in Stone pine. In: Mutke S (ed) Proceedings of the International Meeting on Mediterranean Stone Pine for Agroforestry - AGROPINE 2011. Valladolid, Spain, 17–19 November
Biondi F, Hartsough P, Galindo I (2005) Daily weather and tree growth at the tropical treeline of North America. Antarct Alp Res 37(1):16–24. https://doi.org/10.1657/1523-0430(2005)037[0016:DWATGA]2.0.CO;2
Brito P, Wieser G, Oberhuber W, Gruber A, Lorenzo JR, González-Rodríguez ÁM, Jiménez MS (2017) Water availability drives stem growth and stem water deficit of Pinus canariensis in a drought-induced tree line in Tenerife. Plant Ecol 218(3):277–290. https://doi.org/10.1007/s11258-016-0686-6
Brun R, Tournier JP (1992) Micrometric measurement of stem diameter changes as a means to detect nutritional stress of tomato plants. Acta Hortic 304:265–272. https://doi.org/10.17660/ActaHortic.1992.304.30
Calama R, Madrigal G, Candela J, Montero G (2007) Effects of fertilization on the production of an edible forest fruit: Stone pine (Pinus pinea L.) nuts in the south-west of Andalusia. For Syst 16(3):241–252. https://doi.org/10.5424/srf/2007163-01013
Camarero J, Rubio-Cuadrado Á (2020) Relating climate, drought and radial growth in broadleaf Mediterranean tree and shrub species: a new approach to quantify climate-growth relationships. Forests 11(12):e1250. https://doi.org/10.3390/f11121250
Chambi Legoas R, Tomazello Filho M, Laclau JP, Chaix G (2023) Potassium fertilization enhances xylem plasticity and growth recovery of Eucalyptus grandis trees in response to drastic changes in water availability. For Ecol Manage 528:e120656. https://doi.org/10.1016/j.foreco.2022.120656
Cocozza C, Tognetti R, Giovannelli A (2018) High-resolution analytical approach to describe the sensitivity of tree–environment dependences through stem radial variation. Forests 9(3):e134. https://doi.org/10.3390/f9030134
Correia A, Faias S, Fontes L, Freire J, Mutke S, Silva J, Soares P, Tome M, Pereira J (2016) The needles as drivers of tree productivity: relationship with growth, vitality and cone production in Pinus pinea. In: S. CM, A.C. C, Vila Verde (eds) Proceedings of 2nd International Meeting on Mediterranean Stone Pine for Agroforestry - AGROPINE 2016. INIAV, Oeiras, Portugal, 18–20 May
Deslauriers A, Morin H, Urbinati C, Carrer M (2003) Daily weather response of balsam fir (Abies balsamea (L.) Mill.) stem radius increment from dendrometer analysis in the boreal forests of Québec (Canada). Trees Struct Funct 17(6):477–484. https://doi.org/10.1007/s00468-003-0260-4
Deslauriers A, Anfodillo T, Rossi S, Carraro V (2007a) Using simple causal modeling to understand how water and temperature affect daily stem radial variation in trees. Tree Physiol 27(8):1125–1136. https://doi.org/10.1093/treephys/27.8.1125
Deslauriers A, Rossi S, Anfodillo T (2007b) Dendrometer and intra-annual tree growth: what kind of information can be inferred? Dendrochronologia 25(2):113–124. https://doi.org/10.1016/j.dendro.2007.05.003
Di Rienzo J, Casanoves F, Balzarini M, Gonzalez L, Tablada M, Robledo C (2023). InfoStat version 2023. Grupo InfoStat, FCA, Universidad Nacional de Córdoba, Argentina
Douaihy B, Restoux G, Machon N, Dagher-Kharrat MB (2013) Ecological characterization of the Juniperus excelsa stands in Lebanon. Ecol Mediter 39(1):169–180. https://doi.org/10.3406/ecmed.2013.1303
Downes G, Beadle C, Worledge D (1999) Daily stem growth patterns in irrigated Eucalyptus globulus and E. nitens in relation to climate. Trees 14(2):102–111. https://doi.org/10.1007/PL00009752
Drew DM, Downes GM (2009) The use of precision dendrometers in research on daily stem size and wood property variation: a review. Dendrochronologia 27(2):159–172. https://doi.org/10.1016/j.dendro.2009.06.008
Eker Ö, Laz B (2018) Examining stone pine (Pinus pinea) from ecological and socio-economic perspective in Kahramanmaras. In: Yilmaz M, Gönültas O, Yildirim N, Tas I (eds) Proceedings of the 4th International Non-Wood Forest Products Symposium. Bursa, Turkey, 4–6 October
Espadafor M, Orgaz F, Testi L, Lorite IJ, González-Dugo V, Fereres E (2017) Responses of transpiration and transpiration efficiency of almond trees to moderate water deficits. Sci Hortic 225:6–14. https://doi.org/10.1016/j.scienta.2017.06.028
Ferrer A, Herrero G, Milián C, Aguirre B (2004) Carencias nutrimentales en coníferas cubanas. I. Pinus tropicalis Morelet. Rev for Baracoa 23:23–28
Freire J, Rodrigues G, Tomé M (2019) Climate change impacts on Pinus pinea L. silvicultural system for cone production and ways to contour those impacts: a review complemented with data from permanent plots. Forests 10(2):e169. https://doi.org/10.3390/f10020169
Gessler A, Schaub M, McDowell NG (2017) The role of nutrients in drought-induced tree mortality and recovery. New Phytol 214:513–520. https://doi.org/10.1111/nph.14340
Gruber A, Strobl S, Veit B, Oberhuber W (2010) Impact of drought on the temporal dynamics of wood formation in Pinus sylvestris. Tree Physiol 30(4):490–501. https://doi.org/10.1093/treephys/tpq003
Güney A, Zweifel R, Türkan S, Zimmermann R, Wachendorf M, Güney CO (2020) Drought responses and their effects on radial stem growth of two co-occurring conifer species in the Mediterranean mountain range. Ann For Sci 77(4):e105. https://doi.org/10.1007/s13595-020-01007-2
Gyllenstrand N, Karlgren A, Clapham D, Holm K, Hall A, Gould PD, Kallman T, Lagercrantz U (2014) No time for spruce: rapid dampening of circadian rhythms in Picea abies (L. Karst). Plant Cell Physiol 55:535–550. https://doi.org/10.1093/pcp/pct199
Kilci M (2011) Effects of nutrients on conelet losses of stone pine (Pinus pinea L.) in Kozak province. In: Mutke S (ed) Proceedings of the International Meeting on Mediterranean Stone Pine for Agroforestry - AGROPINE 2011. Valladolid, Spain, 17–19 November
Knüver T, Bär A, Ganthaler A et al (2022) Recovery after long-term summer drought: hydraulic measurements reveal legacy effects in trunks of Picea abies but not in Fagus sylvatica. Plant Biol 24:1240–1253. https://doi.org/10.1111/plb.13444
Kumar J, Raja N, Kumar V (2017) Improving productivity of groundnut (Arachis hypogaea L.) under drip and micro fertigation system. Legum Res 42(1):90–95. https://doi.org/10.18805/LR-3851
Lewis JD, Olszyk D, Tingey DT (1999) Seasonal patterns of photosynthetic light response in Douglas-fir seedlings subjected to elevated atmospheric CO2 and temperature. Tree Physiol 19(4–5):243–252. https://doi.org/10.1093/treephys/19.4-5.243
Li G, Wang J, Oliet J, Jacobs D (2016) Combined pre-hardening and fall fertilization facilitates N storage and field performance of Pinus tabulaeformis seedlings. iForest 9:483–489. https://doi.org/10.3832/ifor1708-008
Lim TK (2012) Pinus pinea. In: Lim TK (ed) Edible medicinal and non-medicinal plants, vol 4. Springer, Dordrecht, pp 304–310. https://doi.org/10.1007/978-94-007-4053-2_39
Limm EB, Simonin KA, Bothman AG, Dawson TE (2009) Foliar water uptake: a common water acquisition strategy for plants of the redwood forest. Oecologia 161(3):449–459. https://doi.org/10.1007/s00442-009-1400-3
Liu X, Nie Y, Wen F (2018) Seasonal dynamics of stem radial increment of Pinus taiwanensis Hayata and its response to environmental factors in the Lushan Mountains, Southeastern China. Forests 9(7):e387. https://doi.org/10.3390/f9070387
Loewe V, Delard C, Balzarini M et al (2015) Impact of climate and management variables on stone pine (Pinus pinea L.) growing in Chile. Agric For Meteorol 214–215:106–116. https://doi.org/10.1016/j.agrformet.2015.08.248
Loewe V, Balzarini M, Álvarez A, Delard C, Navarro-Cerrillo R (2016) Fruit productivity of Stone pine (Pinus pinea L.) along a climatic gradient in Chile. Agric For Meteorol 223(15):203–216. https://doi.org/10.1016/j.agrformet.2016.04.011
Loewe-Muñoz V, Delard C, Del Río R, Balzarini M (2020) Long-term effect of fertilization on stone pine growth and cone production. Ann For Sci 77(3):e69. https://doi.org/10.1007/s13595-020-00978-6
Ma J, Guo J, Wang Y, Liu Z, Gao D, Hong L, Zhang Z (2021) Variations in stem radii of Larix principis-rupprechtii to environmental factors at two slope locations in the Liupan Mountains, northwest China. J For Res 32(2):513–527. https://doi.org/10.1007/s11676-020-01114-w
Malchi T, Shenker M (2011) Iron deficiency of Pinus pinea: evaluation of iron uptake mechanisms and comparison of different genetic lines. Israel: The Hebrew University of Jerusalem. Department of Soil and Water Sciences, The Robert H. Smith Faculty of Agriculture, Food and Environment. 41 p
McClung CR (2006) Plant circadian rhythms. Plant Cell 18:792–803. https://doi.org/10.1105/tpc.106.040980
McMahon S, Parker G (2015) A general model of intra-annual tree growth using dendrometer bands. Ecol Evol 5(2):243–254. https://doi.org/10.1002/ece3.1117
Mencuccini M, Salmon Y, Mitchell P, Hölttä T, Choat B, Meir P, O’Grady A, Tissue D, Zweifel R, Sevanto S, Pfautsch S (2017) An empirical method that separates irreversible stem radial growth from bark water content changes in trees: theory and case studies. Plant Cell Environ 40:290–303. https://doi.org/10.1111/pce.12863
Messinger J, Güney A, Zimmermann R, Ganser B, Bachmann M, Remmele S, Aas G (2015) Cedrus libani: a promising tree species for Central European forestry facing climate change? Eur J For Res 134:1005–1017. https://doi.org/10.1007/s10342-015-0905-z
Montero G, Candela J, Rodríguez (2004) El pino piñonero (Pinus pinea L.) en Andalucía: Ecología, distribución y selvicultura. Consejería de Medio Ambiente, Junta de Andalucía, Sevilla, Spain, 261 p
Niederberger J, Kohler M, Bauhus J (2019) Immobilization of P into wood and bark following fertilization. In: Battipaglia G, Marzaioli R (eds) Proceedings of the Tree Rings in Archaeology, Climatology and Ecology – TRACE 2019. San Leucio, Italy, 7–11 May
Oberhuber W (2017) Soil water availability and evaporative demand affect seasonal growth dynamics and use of stored water in co-occurring saplings and mature conifers under drought. Trees 31(2):467–478. https://doi.org/10.1007/s00468-016-1468-4
Oberhuber W, Gruber A (2010) Climatic influences on intra-annual stem radial increment of Pinus sylvestris (L.) exposed to drought. Trees 24(5):887–898. https://doi.org/10.1007/s00468-010-0458-1
Oberhuber W, Gruber A, Kofler W, Swidrak I (2014) Radial stem growth in response to microclimate and soil moisture in a drought-prone mixed coniferous forest at an inner Alpine site. Eur J For Res 133(3):467–479. https://doi.org/10.1007/s10342-013-0777-z
Oladi R, Elzami E, Pourtahmasi K, Bräuning A (2017) Weather factors controlling growth of Oriental beech are on the turn over the growing season. Eur J For Res 136(2):345–356. https://doi.org/10.1007/s10342-017-1036-5
Oliveras I, Martinez Vilalta J, Jimenez Ortiz T, Lledó MJ, Escarré A, Piñol J (2003) Hydraulic properties of Pinus halepensis, Pinus pinea and Tetraclinis articulata in a dune ecosystem of Eastern Spain. Plant Ecol 169:131–141. https://doi.org/10.1023/A:1026223516580
Perämäki M, Nikinmaa E, Sevanto S, Ilvesniemi H, Siivola E, Hari P, Vesala T (2001) Tree stem diameter variations and transpiration in Scots pine: an analysis using a dynamic sap flow model. Tree Physiol 21(12–13):889–897. https://doi.org/10.1093/treephys/21.12-13.889
R Core Team (2020) R: A language and environment for statistical computing. Foundation for Statistical Computing, Vienna, Austria
Rana G, Ferrara RM (2019) Air cooling by tree transpiration: a case study of, Citrus sinensis and Pinus pinea in Mediterranean town. Urban Clim 29:e100507. https://doi.org/10.1016/j.uclim.2019.100507
Rapp M, Leclerc M, Lossaint P (1979) The nitrogen economy in a Pinus pinea L. stand. For Ecol Manage 2(3):221–231. https://doi.org/10.1016/0378-1127(79)90048-3
Rossi S (2003) Intra-annual dynamics of tree-ring formation and effects of the environmental factors at the timberline in the Eastern Alps. PhD thesis, University of Padova, Padova, Italy
Salomón RL, Peters RL, Zweifel R, Sass-Klaassen UGW, Stegehuis AI, Smiljanic M, Poyatos R, Babst F, Cienciala E, Fonti P, Lerink BJW, Lindner M, Martinez-Vilalta J, Mencuccini M, Nabuurs G-J, van der Maaten E, von Arx G, Bär A, Akhmetzyanov L, Balanzategui D, Bellan M, Bendix J, Berveiller D, Blaženec M, Čada V, Carraro V, Cecchini S, Chan T, Conedera M, Delpierre N, Delzon S, Ditmarová Ľ, Dolezal J, Dufrêne E, Edvardsson J, Ehekircher S, Forner A, Frouz J, Ganthaler A, Gryc V, Güney A, Heinrich I, Hentschel R, Janda P, Ježík M, Kahle H-P, Knüsel S, Krejza J, Kuberski Ł, Kučera J, Lebourgeois F, Mikoláš M, Matula R, Mayr S, Oberhuber W, Obojes N, Osborne B, Paljakka T, Plichta R, Rabbel I, Rathgeber CBK, Salmon Y, Saunders M, Scharnweber T, Sitková Z, Stangler DF, Stereńczak K, Stojanović M, Střelcová K, Světlík J, Svoboda M, Tobin B, Trotsiuk V, Urban J, Valladares F, Vavrčík H, Vejpustková M, Walthert L, Wilmking M, Zin E, Zou J, Steppe K (2022) The 2018 European heatwave led to stem dehydration but not to consistent growth reductions in forests. Nat Commun 13(1):e28. https://doi.org/10.1038/s41467-021-27579-9
Sánchez-Costa E, Poyatos R, Sabaté S (2015) Contrasting growth and water use strategies in four co-occurring Mediterranean tree species revealed by concurrent measurements of sap flow and stem diameter variations. Agric For Meteorol 207:24–37. https://doi.org/10.1016/j.agrformet.2015.03.012
Sattout E, Faour G (2017) Insights on the value chain and management practices of stone pine forests in Lebanon. In: Carrasquinho I, Correia A, Mutke S (eds) Mediterranean pine nuts from forests and plantations. Options Méditerranéennes: Série A. Séminaires Méditerranéens, vol 122. CICHEAM, Zaragoza, Spain, pp 119–124
Schäfer C, Rötzer T, Thurm EA, Biber P, Kallenbach C, Pretzsch H, Schäfer C, Rötzer T, Thurm EA, Biber P, Kallenbach C, Pretzsch H (2019) Growth and tree water deficit of mixed Norway spruce and European beech at different heights in a tree and under heavy drought. Forests 10(7):e577. https://doi.org/10.3390/f10070577
Schlatter J, Gerding V (1984a) Deficiencia de Boro en Plantaciones de Pinus radiata D. Don en Chile I. Distribución y Origen Bosque 6:24–31. https://doi.org/10.4206/bosque.1985.v6n1-03
Schlatter J, Gerding V (1984b) Deficiencia de boro en plantaciones de Pinus radiata D. Don en Chile II. Principales Causas y Corrección Bosque 6:32–43. https://doi.org/10.4206/bosque.1985.v6n1-04
Schönbeck L (2019) Drought effects on carbon and nutrient dynamics in Scots pine. Homeostasis, thresholds and tipping points. Doctoral thesis. Switzerland: University of Basel, 131 p.
Schröder K, Khaldi A, Hasnaoui A (2014) Analyse de la chaîne de valeur “Pignons de pin” en Tunisie, Étude établie par ordre et pour le compte du projet: Adaptation au changement climatique des politiques forestières dans la región MENA. German Agency for International Cooperation (GIZ) and Tunisian General Direction of Forests (DGF). 50 p
Sevanto S, Nikinmaa E, Riikonen A, Daley M, Pettijohn JC, Mikkelsen TN, Phillips N, Holbrook NM (2008) Linking xylem diameter variations with sap flow measurements. Plant Soil 305(1–2):77–90. https://doi.org/10.1007/s11104-008-9566-8
Steppe K, De Pauw DJW, Lemeur R, Vanrolleghem PA (2006) A mathematical model linking tree sap flow dynamics to daily stem diameter fluctuations and radial stem growth. Tree Physiol 26(3):257–273. https://doi.org/10.1093/treephys/26.3.257
Steppe K, Sterck F, Deslauriers A (2015) Diel growth dynamics in tree stems: linking anatomy and ecophysiology. Trends Plant Sci 20(6):335–343. https://doi.org/10.1016/j.tplants.2015.03.015
Swidrak I, Gruber A, Kofler W, Oberhuber W (2011) Effects of environmental conditions on onset of xylem growth in Pinus sylvestris under drought. Tree Physiol 31(5):483–493. https://doi.org/10.1093/treephys/tpr034
Szymczak S, Häusser M, Garel E, Santoni S, Huneau F, Knerr I, Trachte K, Bendix J, Bräuning A (2020) How do Mediterranean pine trees respond to drought and precipitation events along an elevation gradient? Forests 11(7):e758. https://doi.org/10.3390/f11070758
Tardif J, Flannigan M, Bergeron Y (2001) An analysis of the daily radial activity of 7 boreal tree species, northwestern Quebec. Environ Monit Assess 67:141–160. https://doi.org/10.1023/A:1006430422061
Thabeet A, Vennetier M, Gadbin-Henry C, Denelle N, Roux M, Caraglio Y, Vila B (2009) Response of Pinus sylvestris L. to recent climatic events in the French Mediterranean region. Trees 23(4):843–853. https://doi.org/10.1007/s00468-009-0326-z
Tian Y, Zhang Q, Liu X, Meng M, Wang B (2019) The relationship between stem diameter shrinkage and tree bole moisture loss due to transpiration. Forests 10(3):290. https://doi.org/10.3390/f10030290
Toca A, Maroto J, Oliet J, Villar-Salvador P, Martínez-Catalán R (2014) Relation between nitrogen fertilization and the frost resistance of four pine species. In: Proceedings of the 5th International Conference on Mediterranean Pines (Medpine5). Solsona, Spain, 24–26 September
Urrutia-Jalabert R, Rossi S, Deslauriers A, Malhi Y, Lara A (2015) Environmental correlates of stem radius change in the endangered Fitzroya cupressoides forests of southern Chile. Agric For Meteorol 200:209–221. https://doi.org/10.1016/j.agrformet.2014.10.001
Vospernik S, Nothdurft A (2018) Can trees at high elevations compensate for growth reductions at low elevations due to climate warming? Can J For Res 48(6):650–662. https://doi.org/10.1139/cjfr-2017-0326
Wang X, Schönbeck L, Gessler A, Yang Y, Rigling A, Yu D, He P, Li M (2022) The effects of previous summer drought and fertilization on winter non-structural carbon reserves and spring leaf development of downy oak saplings. Front Plant Sci 13:e1035191. https://doi.org/10.3389/fpls.2022.1035191
Wani I, Bhat M, Dar M, Mehraj S, Bisati I, Wani S, Khanday M-U-D (2016) Response of different walnut (Juglans regia L.) selection to combined application of inorganic fertilizers and organic manures. Am J Exp Agric 12(6):1–10. https://doi.org/10.9734/AJEA/2016/24695
Yu B, Huang J-G, Ma Q, Guo X, Liang H, Zhang S, Fu S, Wan S, Yan J, Zhang W (2019) Comparison of the effects of canopy and understory nitrogen addition on xylem growth of two dominant species in a warm temperate forest, China. Dendrochronologia 56:e125604. https://doi.org/10.1016/J.DENDRO.2019.125604
Zalloni E, Battipaglia G, Cherubini P, Saurer M, De Micco V (2018) Contrasting physiological responses to Mediterranean climate variability are revealed by intra-annual density fluctuations in tree rings of Quercus ilex L. and Pinus pinea L. Tree Physiol 38(8):1213–1224. https://doi.org/10.1093/treephys/tpy061
Zweifel R, Zimmermann L, Newbery DM (2005) Modeling tree water deficit from microclimate: an approach to quantifying drought stress. Tree Physiol 25(2):147–156. https://doi.org/10.1093/treephys/25.2.147
Zweifel R, Zimmermann L, Zeugin F, Newbery DM (2006) Intra-annual radial growth and water relations of trees: implications towards a growth mechanism. J Exp Bot 57(6):1445–1459. https://doi.org/10.1093/jxb/erj125
Zweifel R, Drew DM, Schweingruber F, Downes GM (2014) Xylem as the main origin of stem radius changes in Eucalyptus. Funct Plant Biol 41(5):e520. https://doi.org/10.1071/FP13240
Acknowledgements
The trial was established as part of the project “Technique development for producing Stone pine (Pinus pinea) pine nuts, an attractive commercial option for Chile”, funded by FONDEF, CONICYT (2012–2016) (Code D11I1134). The authors thank the Araneda family for providing the plantation, and support for the trial establishment.
Funding
Trial establishment and management during the first four years were funded by FONDEF, ANID (Grant Number D11I1134). Subsequent management, measurements and analyses were funded by the Chilean Ministry of Agriculture and by ANID BASAL FB210015 (CENAMAD).
Author information
Authors and Affiliations
Contributions
VL, CDR and MB contributed to the study conception and design. Material preparation and data collection were performed by RDM. MB directed the statistical analyses. CDR assisted in bibliography revision. VL wrote the first draft of the manuscript. All authors read and approved the final manuscript.
Corresponding author
Ethics declarations
Ethics approval and consent to participate
Not applicable.
Consent for publication
Not applicable.
Competing interests
The authors declare that they have no competing interests.
Additional information
Publisher's Note
Springer Nature remains neutral with regard to jurisdictional claims in published maps and institutional affiliations.
Rights and permissions
Open Access This article is licensed under a Creative Commons Attribution 4.0 International License, which permits use, sharing, adaptation, distribution and reproduction in any medium or format, as long as you give appropriate credit to the original author(s) and the source, provide a link to the Creative Commons licence, and indicate if changes were made. The images or other third party material in this article are included in the article's Creative Commons licence, unless indicated otherwise in a credit line to the material. If material is not included in the article's Creative Commons licence and your intended use is not permitted by statutory regulation or exceeds the permitted use, you will need to obtain permission directly from the copyright holder. To view a copy of this licence, visit http://creativecommons.org/licenses/by/4.0/.
About this article
Cite this article
Loewe-Muñoz, V., del Río Millar, R., Delard Rodriguez, C. et al. Effects of fertilization on radial growth of Pinus pinea explored hourly using dendrometers. Ecol Process 13, 2 (2024). https://doi.org/10.1186/s13717-023-00479-0
Received:
Accepted:
Published:
DOI: https://doi.org/10.1186/s13717-023-00479-0