Abstract
Objective
Adropin, a newly identified regulatory protein has garnered attention given its potential role in metabolism regulation, especially glucose metabolism and insulin resistance. However, studies on the association between adropin and type 2 diabetes mellitus (T2DM) are equivocal. The aim of this study is to assess the association between serum adropin levels and T2DM using a systematic review and meta-analysis of observational studies.
Methods
PubMed, Scopus, ISI Web of science, and Google Scholar were searched, up to August 2022, for studies that reported the association between serum levels of adropin in adults with T2DM compared to a control group without diabetes. A random-effect model was used to compute the pooled weighted mean difference (WMD) with 95% confidence intervals (CI).
Results
Meta-analysis of 15 studies (n = 2813 participants) revealed that the serum adropin concentrations were significantly lower in patients with T2DM compared with the control group (WMD= -0.60 ng/mL, 95% CI: -0.70 to -0.49; I2 = 99.5%). Subgroup analysis also found lower concentration of adropin in patients with T2DM who were otherwise healthy compared to a control group (n = 9; WMD=-0.04 ng/ml, 95% CI= -0.06 to -0.01, p = 0.002; I2 = 96.4).
Conclusions
Our study showed adropin levels are lower in patients with diabetes compared to a control group without diabetes. However, the limitations of observational studies challenge the validity of the results, and further investigations are needed to confirm the veracity of these findings and additionally explore possible mechanisms.
Similar content being viewed by others
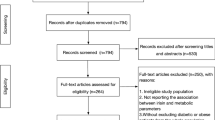
Introduction
Type 2 diabetes mellitus (T2DM) is a major global public health concern. Worldwide, the total number of people with T2DM has inexorably grown, and is estimated that, by 2040, its’ prevalence will reach approximately 570 million patients [1,2,3]. Patients with diabetes experience a wide range of complications, including micro and macro-vascular damages, leading to cardio-vascular diseases, neuropathy, retinopathy, or nephropathy [2, 4]. Given the progressive nature of the diabetes and no definitive cure for it, improved knowledge about the pathogenic pathways could lead to finding new biomarkers for early diagnostic and treatment development of diabetes.
Although the mechanisms underlying diabetes progression are not fully understood, it has been proposed that signaling molecules secreted in multiple tissues (e.g., hepatokines, myokines, adipokines) are involved [5]. One of these molecules, adropin, has recently attracted much attention. Adropin is a polypeptide of 76 amino acids encoded by the Energy Homeostasis Associated (Enho) gene, and Kumar et al., [6] initially identified its’ presence in the liver and brain of mouse models. Interestingly, in humans, adropin is also produced in the same amino acid sequence as mice, in the postrema and dorsal vagal complex areas in the brain and hepatocytes, which are involved in regulation of metabolism and energy hemostasis [6, 7]. Further, adropin has also observed in other areas of the central nervous system and other tissues, including kidney, lung, testes, gut, peripheral blood mononuclear cells, skeletal muscle, endothelial cells, and also is present in the circulatory system [6,7,8,9].
Although studies on adropin are still in their infancy, it has been proposed that adropin produces its multiple functions through interaction with the G protein-coupled receptor 19 (GPR19) [10], mainly in the brain, cardiac, some cancerous cells [10,11,12], and with Nb-3/Notch1 in the brain [13]. Adropin also can improve endothelial cell survival, and neovascularization in human umbilical vein and coronary artery endothelial cells, through modulation of vascular endothelial growth factor receptor-2 (VEGFR2), activation of the PI3K-Akt and ERK1/2 pathways, increased endothelial nitric oxide synthase bioactivity, and nitric oxide-dependent signaling pathways [14].
It is also proposed that adropin-deficient status may play a role in the pathogenesis of diabetes. Studies have reported that adropin-knockout mice showed an increase in adipose tissue and impaired glucose metabolism [15], and adropin treatment of diet-induced obese mice improved glucose metabolism and insulin signaling [6, 16, 17]. Although the exact mechanism has remained poorly defined, one of the underlying mechanisms appears to be associated with downregulation of pyruvate dehydrogenase kinase-4 (PDH kinase-4) expression, which leads to inhibition of fatty acid oxidation and higher glucose oxidation, due to increased activity of pyruvate dehydrogenase, an enzymatic complex involved in acetyl-coenzyme A formation; so it can enter into the citric acid cycle [18]. Moreover, Gao et al. suggested that insulin-induced cell surface expression of glucose transpoter-4 (GLUT4) gene expression is increased by adropin, especially in muscle cells, and is related to improved glucose uptake [16]. Concomitantly, carnitine palmitoyltransferase-1B and CD36, the two critical enzymes in fatty acid metabolism, are inhibited by adropin, reducing fatty acid uptake, and β-oxidation in mouse muscle cells [18]. As fatty acid over load is associated with insulin resistance [19], the regulatory role of adropin in switching the energy substrate from fatty acid to glucose may be contributory to the improvement insulin resistance. Further evidence indicates that adropin can suppress adipogenesis by inhibiting differentiation of 3T3-L1 cells to the mature adipocytes through GPR19 receptor, and ERK1/2 and AKT dependent mechanisms [20, 21]. Moreover, it is well known that obesity is related to increased cellular inflammation. The endoplasmic reticulum is one of the organelles affected by stress, and its homeostasis is disturbed, and, in turn, hepatic c-Jun N-terminal kinase activity increases, leading to phosphorylation of insulin receptor, and suppression of insulin signaling [22]. A recent study found that adropin treatment reduced endoplasmic reticulum stress responses and reduced hepatic c-Jun N-terminal kinase activity, which improved insulin signaling pathways in the liver, consequently [23].
There are a few observational studies showing that adropin levels are reduced in patients with T2DM [15, 24], and women with polycystic ovary syndrome (PCOS) [25, 26]. By contrast, some other studies have reported that serum levels of adropin may increase in patients with T2DM [27, 28]. Given the previous contradictory results, we aimed to investigate the association between adropin levels in patients with T2DM, compared with a control group without diabetes, by performing a systematic review and meta-analysis of observational studies.
Methods
This systematic review and meta-analysis was conducted based on the PRISMA statement (Preferred Reporting Items for Systematic Reviews and Meta-Analyses) [29].
Search strategy
Articles were obtained through a comprehensive systematic electronic search of PubMed, Scopus, Web of Science, and Google Scholar databases, up to 13th August 2022, using the term “adropin”. “Adropin” was searched in all fields of the electronic databases, without any date and language restriction, to comprehensively retrieve all the potentially relevant papers. Moreover, the reference lists of included studies were checked to find additional related articles. Two independent investigators screened articles (SS and MM), and any disagreement was resolved by consultation with a third investigator (SA).
Eligibility criteria
Observational studies that reported the mean and corresponding variance of adropin serum concentrations in adults with T2DM in comparison with a reference group (without diabetes) were eligible for inclusion in this systematic review. The exclusion criteria were as follows: studies which were (a) case reports, editorials, reviews, and conference abstract; (b) conducted in children, pregnant/ lactating women; (c) carried out on cell lines or animal models; (d) enrolled non-diabetic participants, and/or gestational diabetes; and (e) without a reference group for comparison.
Data extraction
Two investigators (SB, MM) extracted data from eligible studies, independently, and any discrepancy was resolved by discussion with the corresponding author. The following data were extracted from each eligible study: first author’s name, year of publication, country of origin, characteristics and sex of studied population, diagnostic criteria for diabetes, sample size, diabetes-related complications, comorbid diseases, methods of adropin measurement, mean and corresponding variance of adropin levels, and potential confounders.
Quality assessment of articles
Quality of the studies was assessed by independent investigators according to the Newcastle-Ottawa Scale (NOS). The NOS tool evaluates the quality of included studies across seven items categorized in three domains: selection, comparability, and outcome with a maximum score of 10 [30]. Studies with a score ≥ 7 were assigned high quality, whereas those with score ≤ 5 were considered low quality.
Statistical analysis
We used the means and their corresponding SD of serum adropin concentrations in cases with T2DM and their controls to calculate the effect size. The weighted mean differences (WMD), with the corresponding 95% CI, were pooled using a random effects model to take into account the variability between true study effects [31]. Cochran’s Q test and I2 were used to assess statistical heterogeneity [32]. Subgroup analyses for study location, diabetes-related complications, and adjustment of confounders were performed to identify the possible sources of heterogeneity. Sensitivity analysis was conducted to describe the effect of individual studies on pooled analysis. Funnel plots and Egger’s tests were used to examine the publication bias [33]. Statistical analyses were performed by STATA version 17 (STATA Corp., College Station, TX, USA), and P values less than 0.05 were considered statistically significant.
Results
Of the 671 retrieved studies, 261 remained after duplicates removed, and 72 remained after title/abstract screening. A total of 62 studies were excluded after screening the full-texts for the following reasons: non-diabetic patients (n = 32) [25, 34,35,36,37,38,39,40,41,42,43,44,45,46,47,48,49,50,51,52,53,54,55,56,57,58,59,60,61,62,63,64], without reference group (n = 1) [17], animal model (n = 19) [7, 23, 65,66,67,68,69,70,71,72,73,74,75,76,77,78,79,80,81], cell studies (n = 4) [12, 82,83,84], and randomized trials (n = 6) [85,86,87,88,89,90]. Update search on the 13th of August 2022, yielded additional five eligible studies. Finally, 15 relevant articles, involving 2813 subjects, were included in the current meta-analysis (Fig. 1).
Study characteristics
Table 1 summarizes the characteristics of the 15 eligible studies [24, 27, 91,92,93,94,95,96,97,98,99,100,101,102,103]. These studies were published between 2014 and 2022, and conducted in China (n = 7) [24, 91, 93, 94, 98, 101, 103], Iran (n = 3) [27, 92, 100], Turkey (n = 2) [95, 99], Iraq (n = 1) [96], India (n = 1) [102], and Ukraine (n = 1) [97]. Of all included studies, four studies were performed using a case-control design [27, 92, 95, 96], and eleven had a cross-sectional design [24, 91, 93, 94, 97,98,99,100,101,102,103]. Thirteen studies enrolled both male and female participants [24, 91,92,93,94,95, 97,98,99,100,101,102,103]; while two studies exclusively included females [96] or males [27]. The participants of four studies had diabetes-related complications, including albuminuria [93, 94], retinopathy [91, 93], and hypertension [97]. The method of serum adropin measurement was ELIZA in all the included studies [24, 27, 91,92,93,94,95,96,97,98,99,100,101,102,103]. The diagnostic criteria for T2DM was based on the American Diabetes Association (ADA) in eight studies [24, 27, 91,92,93,94, 98, 102], European Association for the Study of Diabetes (EASD) in one study [97], HOMA-IR cut-off in one study [101], and World Health Organization (WHO) in another study [93]. The remaining five studies did not provide information about the diagnosis criteria [95, 96, 99, 100, 103]. The confounding factors that were examined in the analyses included age and sex [24, 91, 93, 94, 98], BMI [24, 93, 98], lipid profile [27, 93, 98], and blood pressure values [93, 98]. However, nine studies reported non-adjusted association [92, 95,96,97, 99,100,101,102,103].
The results of quality assessment, based on the Newcastle-Ottawa scale, suggested that the six studies had good methodological quality [24, 27, 91, 93, 94, 98], whilst others were of moderate [92, 97, 99,100,101,102,103], and low quality [95, 96], respectively (Table 2). Except for four studies [96, 97, 99, 101], all the included studies used a sample that was representative of the average of our interested population. However, no description was provided about the characteristics of the responders and the non-responders across all studies. Another source of bias was related to the confounding factors, which were not controlled for [92, 95,96,97, 99,100,101,102,103], or a few number of possible confounding factors were considered, in the analyses [27]. Finally, no details were provided for the assessment of outcome in two studies [95, 96], and no score was assigned for this domain.
Meta-analysis
Overall, meta-analysis of 15 studies [24, 27, 91,92,93,94,95,96,97,98,99,100,101,102,103] (n = 2,813 participants) revealed that the serum levels of adropin were significantly lower in patients with T2DM compared with non-diabetic participants (WMD= -0.60 ng/mL, 95% CI: -0.70 to -0.49, P < 0.001; I2 = 99.48%, P-heterogeneity < 0.001) (Fig. 2). A high between-study heterogeneity was observed, of unknown origin, even after subgroup analysis. The subgroup analysis indicated lower levels of adropin in patients with T2DM otherwise healthy (n = 9; WMD= -0.60 ng/mL, 95% CI: -0.70 to -0.49; I2 = 99.5%). Moreover, a lower adropin levels was observed in patients with T2DM in sex and age-adjusted studies (n = 5; WMD=-0.90 ng/ml, 95% CI= -0.70 to -0.49, p < 0.001; I2 = 97.2), compared to the studies did not adjust for these confounders (n = 10; WMD= -0.58 ng/ml, 95% CI= -0.71 to -0.45, p < 0.001; I2 = 99.55). Moreover, pooling data of BMI-adjusted studies failed to show a significant association between adropin levels and T2DM (n = 3; WMD= -0.69 ng/ml, 95% CI= -1.45 to 0.06, p < 0.001; I2 = 94.8), this is while, non-adjusted associations showed a significant lower level of adropin in patients with T2DM compared to the control group (n = 12; WMD= -0.67 ng/ml, 95% CI= -0.79 to -0.54, p < 0.001; I2 = 99.5) (Table 3).
Sensitivity analysis and publication bias
Findings from sensitivity analysis indicated results remained stable after removal of each study, individually, from the analysis.
As assessed by visual inspection, no asymmetry in the funnel plots was identified. Likewise, no significant publication bias was detected using asymmetry tests for meta-analysis (Begg’s test, P = 0.839; Egger’s test, P = 0.217).
Discussion
The main finding of this meta-analysis indicated a significantly lower adropin concentration in patients with T2DM compared with a non-diabetic control group. This association was more pronounced when the analysis was adjusted for age and sex in original studies.
In support of our finding, evidence from animal models demonstrated a decrease in hepatic gluconeogenic regulatory enzymes, blood glucose levels, HOMA-IR, and a marked increase in serum insulin levels and insulin sensitivity index following adropin treatment [7, 18, 104, 105].
These beneficial effects of adropin have been explained via sensitization of insulin signaling pathways, and improved glucose oxidation. Adropin seems to increase insulin-mediated AKT phosphorylation and augments GLUT4 cell-surface expression in muscle cells, leading to glucose oxidation in these cells [16]. Furthermore, adropin is proposed to downregulate pyruvate dehydrogenase kinase gene expression (PDH kinase-4), and subsequently reduce its inhibitory effect on pyruvate dehydrogenase complex, and increased glycolysis [16]. Another recent study reported enhanced AKT signaling pathway, and suppression activity of FoxO1 and GSK3 by adropin treatment, resulting to a reduction in hepatic glucose production, and increased glycogen synthesis [23]. However, in the present study, we found significantly lower levels of adropin in patients with diabetes. As a possible justification, some studies reported increased adropin levels following hyperglycemia [7, 81, 105, 106], and since none of the included studies examined newly diagnosed patients, the observed decreased adropin levels may be due to damage to the organs responsible for the production of adropin, which is also targeted for diabetes, such as the pancreas or liver.
A reduction in adropin levels in obesity may also have contributed to the observed results [26, 98, 101, 107]. Although not all studies reported the body weight of participants, this justification is not implausible, given the high-prevalence of obesity in patients with T2DM. In support of this hypothesis, the association between lower levels of adropin and T2DM disappeared when our analysis was restricted to BMI-adjusted associations. Although it is not yet well understood how adropin levels decrease in the presence of obesity, it has been shown that adropin-knockout mice exhibit development of insulin resistance and metabolic dysregulation, and treatment with exogenous adropin, may improve these conditions [15]. Ghoshal et al., [108] also suggested a complex age-dependent association between adropin levels and obesity indices. Another study also found lower levels of adropin concentrations in obese patients compared to non-obese healthy individuals [109]. However, it must be acknowledged that some studies did not observe any significant association between obesity and adropin levels [107, 110]. Our recent meta-analysis on the adropin levels and obesity found a mean value of adropin levels in obese patients 2.01 ± 1.29 ng/mL, which was significantly lower compared to non-obese participants (WMD = − 0.96 ng/ml, 95% CI = − 1.72 to − 0.19, P = 0.01) [111]. However, in the current study, we found a relatively higher concentration of adropin in patients with T2DM (2.58 ± 0.8 ng/mL); which may be contributory to the low number of included studies in our previous study (n = 5), and a wide interval of reported values, which may not be a true representation of the obese population.
As another explanation, some studies have shown an inverse association between inflammation and adropin levels [112, 113]. As a chronic low-grade inflammation has been observed in diabetes, it may reduce the expression of the Enho gene in this situation. Akcilar et al. [104] also confirm this hypothesis, and showed an increase in TNF-α and IL-6 in diabetes-induced rats, which decreased after intraperitoneal adropin application. Additionally, Wu et al. [24] found a significant increase of hs-CRP in patients with diabetes in comparison to control group, and also an inverse association between adropin and hs-CRP levels.
Our results also showed that age and sex may affect adropin levels, which was in line with some previous studies [9, 108]. Although the mechanisms are not completely clear, it may be that sex-dependent hormonal differences and age-related metabolism changes contributed. Moreover, this can indirectly indicate an association between adiposity and adropin levels, which is affected by age and sex. Further studies evaluating these confounding factors are required to discern the precise mechanisms for this interaction.
The current systematic review and meta-analysis is, to date, the first comprehensive analysis to evaluate the association between serum adropin concentration and T2DM. Nevertheless, there are some potential limitations. Our meta-analysis is based on observational studies, which questions the validity of the results. Observational evidence is known to be to prone confounding bias, as several important characteristics may not be adjusted in the studies; for instance, in the present study weight of participants, medicines and treatment approaches, dietary intake, physical activity, duration of T2DM history, body composition etc., were not considered in original studies. Moreover, adropin gene expression in liver is thought to be affected by circadian rhythm, macronutrient intake, and weight changes. However, it is not clear whether studies took these confounding issues into account, which may bias our results. In addition, included studies did not provide sufficient data to perform additional analysis for the association between serum adropin and indices of glucose homeostasis. On the other hand, different populations, settings, and adjustment methods between studies may produce a high heterogeneity when pooling observational evidence, as we also observed an unknown source of heterogeneity between studies. It should also be noted we pooled together results of adropin that were measured either in serum or plasma, which may be the source of heterogeneity. Inclusion criteria, and matching process are also often less restrictive in observational studies than randomized trials [114]. Furthermore, observational evidence is prone to presence of selection bias since no randomization is performed, and therefore they cannot provide any casual inferences. Despite the aforementioned limitations, meta-analysis of observational analysis can be useful when clinical data is not sufficient. Finally, all included studies, except one, were conducted in Asian nations, which may have reduced the generalizability of our results, rendering them non-applicable, or of limited applicability, to other populations. Overall, the interpretation made from the findings of this meta-analysis should be taken cautiously.
Conclusion
In conclusion, our meta-analysis demonstrated that there is an inverse association between adropin levels and T2DM; it seems that adropin might be a viable candidate for eliciting a protective effect against T2DM. However, this finding was manifest from a low number of studies which could decrease the robustness of our findings. More investigations are still needed to clarify the molecular mechanisms that justify this association in different geographical zones.
Data Availability
The datasets used and/or analysed during the current study are available from the corresponding author on reasonable request.
References
DeFronzo RA, Ferrannini E, Groop L, Henry RR, Herman WH, Holst JJ, et al. Type 2 diabetes mellitus. Nat reviews Disease primers. 2015;1:1–22.
Zheng Y, Ley SH, Hu FB. Global aetiology and epidemiology of type 2 diabetes mellitus and its complications. Nat Reviews Endocrinol. 2018;14:88.
Chatterjee S, Khunti K, Davies MJ. Type 2 diabetes. The Lancet. 2017;389:2239–51.
Saran R, Robinson B, Abbott KC, Agodoa LY, Albertus P, Ayanian J, et al. US renal data system 2016 annual data report: epidemiology of kidney disease in the United States. Am J Kidney Dis. 2017;69:A7–A8.
Rabe K, Lehrke M, Parhofer KG, Broedl UC. Adipokines and insulin resistance. Mol Med. 2008;14:741–51.
Kumar KG, Trevaskis JL, Lam DD, Sutton GM, Koza RA, Chouljenko VN, et al. Identification of adropin as a secreted factor linking dietary macronutrient intake with energy homeostasis and lipid metabolism. Cell Metabol. 2008;8:468–81.
Butler AA, Zhang J, Price CA, Stevens JR, Graham JL, Stanhope KL, et al. Low plasma adropin concentrations increase risks of weight gain and metabolic dysregulation in response to a high-sugar diet in male nonhuman primates. J Biol Chem. 2019;294:9706–19.
Yolbaş S, Kara M, Kalaycı M, Yıldırım A, Gündoğdu B, Aydın S et al. ENHO gene expression and serum adropin level in rheumatoid arthritis and systemic lupus erythematosus. 2018.
Butler AA, Tam CS, Stanhope KL, Wolfe BM, Ali MR, O’Keeffe M, et al. Low circulating adropin concentrations with obesity and aging correlate with risk factors for metabolic disease and increase after gastric bypass surgery in humans. J Clin Endocrinol Metabolism. 2012;97:3783–91.
Stein LM, Yosten GL, Samson WK. Adropin acts in brain to inhibit water drinking: potential interaction with the orphan G protein-coupled receptor, GPR19. Am J Physiology-Regulatory Integr Comp Physiol. 2016;310:R476–R80.
Rao A, Herr DR. G protein-coupled receptor GPR19 regulates E-cadherin expression and invasion of breast cancer cells. Biochim et Biophys Acta (BBA)-Molecular Cell Res. 2017;1864:1318–27.
Thapa D, Stoner MW, Zhang ML, Xie BX, Manning JR, Guimaraes D, et al. Adropin regulates pyruvate dehydrogenase in cardiac cells via a novel GPCR-MAPK-PDK4 signaling pathway. Redox Biol. 2018;18:25–32.
Wong C-M, Wang Y, Lee JTH, Huang Z, Wu D, Xu A et al. Adropin is a brain membrane-bound protein regulating physical activity via the NB-3/Notch signaling pathway in mice. 2014;289:25976–86.
Lovren F, Pan Y, Quan A, Singh KK, Shukla PC, Gupta M et al. Adropin is a novel regulator of endothelial function. 2010;122:S185-S92.
Ganesh-Kumar K, Zhang J, Gao S, Rossi J, McGuinness OP, Halem HH, et al. Adropin deficiency is associated with increased adiposity and insulin resistance. Obesity. 2012;20:1394–402.
Gao S, McMillan RP, Zhu Q, Lopaschuk GD, Hulver MW, Butler AA. Therapeutic effects of adropin on glucose tolerance and substrate utilization in diet-induced obese mice with insulin resistance. Mol metabolism. 2015;4:310–24.
Choi HN, Yim JE. Plasma adropin as a potential marker Predicting obesity and obesity-associated Cancer in korean patients with type 2 diabetes Mellitus. J Cancer Prev. 2018;23:191–6.
Gao S, McMillan RP, Jacas J, Zhu Q, Li X, Kumar GK, et al. Regulation of substrate oxidation preferences in muscle by the peptide hormone adropin. Diabetes. 2014;63:3242–52.
Koves TR, Ussher JR, Noland RC, Slentz D, Mosedale M, Ilkayeva O, et al. Mitochondrial overload and incomplete fatty acid oxidation contribute to skeletal muscle insulin resistance. Cell Metabol. 2008;7:45–56.
Jasaszwili M, Billert M, Strowski MZ, Nowak KW, Skrzypski M. Adropin as a Fat-Burning hormone with multiple functions—review of a Decade of Research. Molecules. 2020;25:549.
Jasaszwili M, Wojciechowicz T, Billert M, Strowski MZ, Nowak KW, Skrzypski MJM et al. Effects of adropin on proliferation and differentiation of 3T3-L1 cells and rat primary preadipocytes. 2019;496:110532.
Ozcan U, Cao Q, Yilmaz E, Lee A-H, Iwakoshi NN, Ozdelen E et al. Endoplasmic reticulum stress links obesity, insulin action, and type 2 diabetes. 2004;306:457–61.
Gao S, Ghoshal S, Zhang L, Stevens JR, McCommis KS, Finck BN, et al. The peptide hormone adropin regulates signal transduction pathways controlling hepatic glucose metabolism in a mouse model of diet-induced obesity. J Biol Chem. 2019;294:13366–77.
Wu L, Fang J, Chen L, Zhao Z, Luo Y, Lin C, et al. Low serum adropin is associated with coronary atherosclerosis in type 2 diabetic and non-diabetic patients. Clin Chem Lab Med. 2014;52:751–8.
Kume T, Calan M, Yilmaz O, Kocabas G, Yesil P, Temur M, et al. A possible connection between tumor necrosis factor alpha and adropin levels in polycystic ovary syndrome. J Endocrinol Investig. 2016;39:747–54.
Bousmpoula A, Kouskouni E, Benidis E, Demeridou S, Kapeta-Kourkouli R, Chasiakou A, et al. Adropin levels in women with polycystic ovaries undergoing ovarian stimulation: correlation with lipoprotein lipid profiles. Gynecol Endocrinol. 2018;34:153–6.
Hosseini A, Shanaki M, Emamgholipour S, Nakhjavani M, Razi F, Golmohammadi T. Elevated serum levels of adropin in patients with type 2 diabetes mellitus and its association with insulin resistance. J Biology Today’s World. 2016;5:44–9.
Ugur K, Oz B, Ozkan Y, Sener SY, Orhan B, Aydin S, editors. Microalbuminuria by concentration serum and urine levels of adropin in patients with type 2 diabetes mellitus. Endocrine Abstracts; 2015.
Moher D, Shamseer L, Clarke M, Ghersi D, Liberati A, Petticrew M, et al. Preferred reporting items for systematic review and meta-analysis protocols (PRISMA-P) 2015 statement. Syst reviews. 2015;4:1.
Lo CK-L, Mertz D, Loeb M. Newcastle-Ottawa Scale: comparing reviewers’ to authors’ assessments. BMC Med Res Methodol. 2014;14:45.
Egger M, Davey-Smith G, Altman D. Systematic reviews in health care: meta-analysis in context. John Wiley & Sons; 2008.
Higgins JP, Thompson SG. Quantifying heterogeneity in a meta-analysis. Stat Med. 2002;21:1539–58.
Egger M, Smith GD, Schneider M, Minder C. Bias in meta-analysis detected by a simple, graphical test. BMJ. 1997;315:629–34.
Alaaraji SFT. Correlation of calprotectin with galectin-3, adropin and CTLA-4 in iraqi rheumatoid arthritis patients. Int J Pharm Res. 2020;12:932–9.
Bolayir HA, Kivrak T, Gunes H, Bolayir A, Karaca I. Adropin and circadian variation of blood pressure. Kardiologia Polska. 2018;76:776–82.
Caf F, Algul S, Koyun M. Investigation of the irisin, preptin and adropin levels in the blood serum of Alburnus tarichi. Cell Mol Biol. 2017;63:95–9.
Cao JF, Wang MS, Bian CY, Tao YF, You T, Xu WT, et al. Association between serum adropin and subclinical atherosclerosis in patients with hyperlipidemia. Int J Clin Exp Med. 2019;12:9351–8.
Conte D, Nordio M, Ronnanelli F, Manganelli F, Giovenco P, Dondero F, et al. Role of seminal prostaglandins in male fertility. II. Effects of prostaglandin synthesis inhibition on spermatogenesis in man. J Endocrinological Investigation: Official J Italian Soc Endocrinol. 1985;8:289–91.
Eraslan E, Topdagi O, Tanyeli A, Guler MC, Sebin E, Sebin SO, et al. Can insulin-like Protein-3 and Adropin be a new marker in Hepatocellular Carcinoma? Acta Physiol. 2018;225:34.
Eser Karlidag G, Arslan Solmaz O. Are adropin, apelin, elabela, asprosin and betatrophin biomarkers for chronic hepatitis and staging of fibrosis? Biotech Histochem. 2020;95:152–9.
Grzegorzewska AE, Niepolski L, Mostowska A, Warchoł W, Jagodziński PP. Involvement of adropin and adropin-associated genes in metabolic abnormalities of hemodialysis patients. Life Sci. 2016;160:41–6.
Gu XS, Li H, Zhu XY, Gu HB, Chen JC, Wang LC et al. Inverse Correlation Between Plasma Adropin and ET-1 Levels in Essential Hypertension A Cross-Sectional Study.Medicine. 2015;94.
Gulen B, Eken C, Kucukdagli OT, Serinken M, Kocyigit A, Kılıc E, et al. Adropin levels and target organ damage secondary to high blood pressure in the ED. Am J Emerg Med. 2016;34:2061–4.
Gundogar H, Ozsoy B, Uzunkaya M, Senyurt SZ, Erciyas K. Effect of Peri-Implant Disease on Adropin levels: a cross-sectional pilot study. Eur J Ther. 2020;26:61–5.
Kaluzna M, Hoppe K, Schwermer K, Ibrahim AY, Pawlaczyk K, Ziemnicka K. Adropin and irisin levels in relation to nutrition, body composition, and insulin resistance in patients with end-stage renal disease on chronic hemodialysis and peritoneal dialysis. Polskie Arch Medycyny Wewnetrznej-Polish Archives Intern Med. 2016;126:474–82.
Lu Z, Chen X, Wang S. Associations of serum adropin level with severity of coronary artery disease and slow Flow in patients with Non-ST-elevation ACS. Chin Gen Pract. 2020;23:2895–900.
Niu YX, Wang XL, Wang DQ, Jiang XF. Changes of serum vitamin D levels in infertile patients with polycystic ovarian syndrome and its significance. Int J Clin Exp Med. 2020;13:3442–8.
Oruc CU, Akpinar YE, Dervisoglu E, Amikishiyev S, Salmaslioglu A, Gurdol F, et al. Low concentrations of adropin are associated with endothelial dysfunction as assessed by flow-mediated dilatation in patients with metabolic syndrome. Clin Chem Lab Med. 2017;55:139–44.
Rao A, Herr DR. G protein-coupled receptor GPR19 regulates E-cadherin expression and invasion of breast cancer cells. Biochim et Biophys Acta - Mol Cell Res. 2017;1864:1318–27.
Sanchis-Gomar F, Alis R, Rampinini E, Bosio A, Ferioli D, La Torre A, et al. Adropin and apelin fluctuations throughout a season in professional soccer players: are they related with performance? Peptides. 2015;70:32–6.
Tuna BG, Atalay PB, Altunbek M, Kalkan BM, Dogan S. Effects of chronic and intermittent calorie restriction on adropin levels in breast Cancer. Nutr Cancer. 2017;69:1003–10.
Wang HD, Wang XJ, Cao Y, Han WX, Guo YJ, Yang GS et al. Association of polymorphisms of preptin, irisin and adropin genes with susceptibility to coronary artery disease and hypertension.Medicine. 2020;99.
Zhang C, Zhao L, Xu W, Li J, Wang B, Gu X, et al. Correlation of serum adropin level with coronary artery disease. Natl Med J China. 2014;94:1255–7.
Grzegorzewska AE, Niepolski L, Swiderska MK, Mostowska A, Stolarek I, Warchol W, et al. ENHO, RXRA, and LXRA polymorphisms and dyslipidaemia, related comorbidities and survival in haemodialysis patients. BMC Med Genet. 2018;19:194.
Yosaee S, Khodadost M, Esteghamati A, Speakman JR, Shidfar F, Nazari MN, et al. Metabolic syndrome patients have lower levels of Adropin when compared with healthy Overweight/Obese and lean subjects. Am J Men’s Health. 2017;11:426–34.
Ogurel T, Ogurel R, Topuz M, Ornek N, Ornek K. Plasma adropin level in patients with pseudoexfoliation. Int Ophthalmol. 2016;36:737–42.
Sen H, Erbag G, Binnetoglu E, Eroglu M, Turkon H, Tekin SZ, et al. Adropin levels in polycystic ovary syndrome patients. J Clin Anal Med. 2017;8:23–6.
Kuliczkowska-Płaksej J, Mierzwicka A, Jończyk M, Stachowska B, Urbanovych A, Bolanowski M. Adropin in women with polycystic ovary syndrome. Endokrynologia Polska. 2019;70:151–6.
Bozic J, Borovac JA, Galic T, Kurir TT, Supe-Domic D, Dogas Z. Adropin and inflammation biomarker levels in male patients with obstructive sleep apnea: a link with glucose metabolism and sleep parameters. J Clin Sleep Med. 2018;14:1109–18.
Wang B, Xue Y, Shang F, Ni S, Liu X, Fan B et al. Association of serum adropin with the presence of atrial fibrillation and atrial remodeling.Journal of Clinical Laboratory Analysis. 2019;33.
Hamdi RA, Abas HN, Alsaeed FAA. Role of adropin in women with polycystic ovary syndrome. Indian J Public Health Res Dev. 2019;10:809–14.
Zhao LP, You T, Chan SP, Chen JC, Xu WT. Adropin is associated with hyperhomocysteine and coronary atherosclerosis. Experimental and Therapeutic Medicine. 2016;11:1065–70.
Korkmaz S, Sayılan Özgün G. Serum adropin levels in psoriasis vulgaris and its relation with metabolic parameters. Turk J Med Sci. 2019;49:110–5.
Demircelik B, Kurtul A, Ocek H, Cakmak M, Cetin M, Ureyen C, et al. The relationship between adropin levels and the slow coronary Flow Phenomenon. Indian J Clin Biochem. 2015;30:412–7.
Gheit AE, Atef RE, El Deeb MM, Badawi OS, Alshenawy GA, Elwan HA. WM, et al. Unique novel role of Adropin in the gastric Ulcer in a rotenone-induced rat model of Parkinson’s disease. ACS chemical neuroscience; 2020.
Akcilar R, Emel Kocak F, Simsek H, Akcilar A, Bayat Z, Ece E, et al. The effect of adropin on lipid and glucose metabolism in rats with hyperlipidemia. Iran J basic Med Sci. 2016;19:245–51.
Altintas O, Kumas M, Altintas MO. Neuroprotective effect of ischemic preconditioning via modulating the expression of adropin and oxidative markers against transient cerebral ischemia in diabetic rats. Peptides. 2016;79:31–8.
Aydin MA, Aydogdu N, Tastekin E, Kandemir N, Yavuz OY, Kudaibergenova A. Investigation of the relation of Nesfatin-1 and adropin with blood pressure in experimental hypertension model. Neuroendocrinology. 2018;107:32.
Aydin S, Aydin S, Kuloglu T, Kalayci M, Yilmaz M, Çakmak T, et al. Elevated adropin: a candidate diagnostic marker for myocardial infarction in conjunction with troponin-I. Peptides. 2014;58:91–7.
Aydin S, Eren MN, Kuloglu T, Aydin S, Yilmaz M, Gul E, et al. Alteration of serum and cardiac tissue adropin, copeptin, irisin and TRPM2 expressions in DOX treated male rats. Biotech Histochem. 2015;90:197–205.
Billert M, Jasaszwili M, Strowski M, Nowak KW, Skrzypski M. Adropin suppresses insulin expression and secretion in INS-1E cells and rat pancreatic islets.Journal of physiology and pharmacology: an official journal of the Polish Physiological Society. 2020;71.
Bremer AA, Stanhope KL, Graham JL, Cummings BP, Ampah SB, Saville BR, et al. Fish oil supplementation ameliorates fructose-induced hypertriglyceridemia and insulin resistance in adult male rhesus macaques. J Nutr. 2014;144:5–11.
Chao HC, Tsai PF, Lee SC, Lin YS, Wu MC. Effects of Myricetin-Containing ethanol solution on High-Fat Diet Induced obese rats. J Food Sci. 2017;82:1947–52.
Chen X, Sun X, Shen T, Chen Q, Chen S, Pang J, et al. Lower adropin expression is associated with oxidative stress and severity of nonalcoholic fatty liver disease. Free Radic Biol Med. 2020;160:191–8.
Gulmez A, Celiker H, Bicim S, Aydin S, Kuloglu T. The investigation of the Therapeutic Effect of Adropin on Gentamicin-Induced Experimental Nephropathy. Turkish Nephrol Dialysis Transplantation J. 2018;27:240–8.
Kedzierski W, Cywniska A, Wawak T, Janczarek I, Wilk I, Kowalik S. Plasma apelin concentration in exercised horses: preliminary study. J Equine Veterinary Sci. 2019;80:16–9.
Mohamed FH, Ozer C. The effect of systemic Adropin treatment on levels of oxidant and antioxidant of Cardiac muscle in Diabetic rats. Acta Physiol. 2019;227:60.
Pawlowska-Olszewska M, Puzio I, Tymicki G, Kalisz G, Sroka-Bartnicka A, Blicharz-Kania A et al. Adropin, nesfatin-1 and angiotensin ii receptor expression in the abdominal aorta in ovariectomized rats after nesfatin-1 treatment.Journal of Physiology and Pharmacology. 2019;70.
Skugor A, Kjos NP, Sundaram AYM, Mydland LT, Anestad R, Tauson AH, et al. Effects of long-term feeding of rapeseed meal on skeletal muscle transcriptome, production efficiency and meat quality traits in norwegian landrace growing-finishing pigs. PLoS ONE. 2019;14:e0220441.
Thapa D, Xie B, Manning JR, Zhang M, Stoner MW, Huckestein BR et al. Adropin reduces blood glucose levels in mice by limiting hepatic glucose production.Physiological Reports. 2019;7.
Kuloglu T, Aydin S. Immunohistochemical expressions of adropin and ınducible nitric oxide synthase in renal tissues of rats with streptozotocin-ınduced experimental diabetes. Biotech Histochem. 2014;89:104–10.
Wu LZ, Fang J, Yuan X, Xiong C, Chen LL. Adropin reduces hypoxia/reoxygenation-induced myocardial injury via the reperfusion injury salvage kinase pathway. Experimental and Therapeutic Medicine. 2019;18:3307–14.
Muter J, Alam MT, Vrljicak P, Barros FSV, Ruane PT, Ewington LJ, et al. The glycosyltransferase EOGT regulates adropin expression in decidualizing human endometrium. Endocrinology. 2018;159:994–1004.
Sato K, Yamashita T, Shirai R, Shibata K, Okano T, Yamaguchi M et al. Adropin contributes to anti-atherosclerosis by suppressing monocyte-endothelial cell adhesion and smooth muscle cell proliferation.International Journal of Molecular Sciences. 2018;19.
Alizadeh R, Golestani N, Moradi L, Rezaeinezhad N. Effect of aerobic exercise with maximal fat oxidation intensity, on adropin and insulin resistance among overweight women.Iranian Journal of Endocrinology and Metabolism. 2018;20.
Celik HT, Akkaya N, Erdamar H, Gok S, Kazanci F, Demircelik B, et al. The Effects of Valsartan and Amlodipine on the levels of Irisin, Adropin, and Perilipin. Clin Lab. 2015;61:1889–95.
Wei S, Gao Q, Wang E. Effects of high- intensity interval training on plasma adropin concentration and endothelial function in male obese youth. Chin J Rehabilitation Med. 2019;34:1058–64.
Stevens JR, Kearney ML, St-Onge MP, Stanhope KL, Havel PJ, Kanaley JA, et al. Inverse association between carbohydrate consumption and plasma adropin concentrations in humans. Obesity. 2016;24:1731–40.
St-Onge MP, Shechter A, Shlisky J, Tam CS, Gao S, Ravussin E, et al. Fasting plasma adropin concentrations correlate with fat consumption in human females. Obesity. 2014;22:1056–63.
Zarrati M, Raji Lahiji M, Salehi E, Yazdani B, Razmpoosh E, Shokouhi Shoormasti R, et al. Effects of Probiotic Yogurt on serum Omentin-1, Adropin, and Nesfatin-1 concentrations in overweight and obese participants under low-calorie Diet. Probiotics and Antimicrobial Proteins; 2018.
Li S, Sun J, Hu W, Liu Y, Lin D, Duan H, et al. The association of serum and vitreous adropin concentrations with diabetic retinopathy. Ann Clin Biochem. 2019;56:253–8.
Palizban A-A, Yazdani A-H, Jahanbani-Ardakani H. Role of rs7903146 polymorphism and adropin serum level in patients with diabetes mellitus; a case–control study from Isfahan,Iran. Archives of Physiology and Biochemistry. 2019:1–4.
Li B, Li N, Guo S, Zhang M, Li J, Zhai N, et al. The changing features of serum adropin, copeptin, neprilysin and chitotriosidase which are associated with vascular endothelial function in type 2 diabetic retinopathy patients. J Diabetes Complicat. 2020;34:107686.
Hu W, Chen L. Association of serum adropin concentrations with diabetic nephropathy. Mediators of inflammation. 2016;2016.
Kutlu O, Altun Ö, Dikker O, Aktaş Ş, Özsoy N, Arman Y, et al. Serum adropin levels are reduced in adult patients with nonalcoholic fatty liver disease. Med Principles Pract. 2019;28:463–9.
Ali HA, Abbas HJ, Naser NA. Preptin and adropin levels as new predictor in women with polycystic ovary syndrome. J Pharm Sci Res. 2018;10:3005–8.
Shelest B. Correlation of adropin, chemerin and inflammation in hypertensive patients with type 2 diabetes mellitus and obesity. Kardiologija v Belarusi. 2018;10:766–74.
Zang H, Jiang F, Cheng X, Xu H, Hu X. Serum adropin levels are decreased in chinese type 2 diabetic patients and negatively correlated with body mass index. Endocr J. 2018;65:685–91.
Aydin P, Uzuncakmak SK, Tor IH, Bilen A, Ozden A. Comparison of serum adropin levels in patients with diabetes Mellitus, COVID-19, and COVID-19 with diabetes Mellitus. Eurasian J Med. 2022;54:197–201.
Es-Haghi A, Al-Abyadh T, Mehrad-Majd H. The clinical value of serum Adropin Level in Early Detection of Diabetic Nephropathy. Kidney Blood Press Res. 2021;46:734–40.
Li N, Xie GM, Zhou BA, Qu AJ, Meng H, Liu J et al. Serum Adropin as a Potential Biomarker for Predicting the Development of Type 2 Diabetes Mellitus in Individuals With Metabolic Dysfunction-Associated Fatty Liver Disease.Frontiers in Physiology. 2021;12.
Shah N, Anand P, Kumar S, Kulkarni P. Evaluation of serum adropin levels in type 2 diabetic patients and its correlation with insulin resistance: a tertiary care teaching hospital-based study. J Datta Meghe Inst Med Sci Univ. 2021;16:658–61.
Zhang XK, Li BX, Li N, Zhai N, Zhang YL, Liu LF, et al. Changes of serum adropin protein and neprilysin levels in patients with type 2 diabetic retinopathy and analysis of influening factors. Int Eye Sci. 2020;20:504–8.
Akcilar R, Kocak F, Simsek H, Akcilar A, Bayat Z, Ece E, et al. Antidiabetic and hypolipidemic effects of adropinin streoptozotocin-induced type 2 diabetic rats. Bratisl Lek Listy. 2016;117:100.
Kuo FY, Cheng K-C, Li Y, Cheng J-T, Tsai C-C. Promotion of Adropin Expression by Hyperglycemia Is Associated with STAT3 Activation in Diabetic Rats. Diabetes, Metabolic Syndrome and Obesity: Targets and Therapy. 2020;13:2269.
Aydin S, Kuloglu T, Aydin S, Eren MN, Yilmaz M, Kalayci M, et al. Expression of adropin in rat brain, cerebellum, kidneys, heart, liver, and pancreas in streptozotocin-induced diabetes. Mol Cell Biochem. 2013;380:73–81.
Inal ZO, Erdem S, Gederet Y, Duran C, Kucukaydin Z, Kurku H, et al. The impact of serum adropin and ischemia modified albumin levels based on BMI in PCOS. Endokrynologia Polska. 2018;69:135–41.
Ghoshal S, Stevens JR, Billon C, Girardet C, Sitaula S, Leon AS, et al. Adropin: an endocrine link between the biological clock and cholesterol homeostasis. Mol metabolism. 2018;8:51–64.
Erman H, Ozdemir A, Sitar ME, Cetin SI, Boyuk BJJoIM. Role of serum adropin measurement in the assessment of insulin resistance in obesity. 2021;69:1318–23.
Topuz M, Celik A, Aslantas T, Demir AK, Aydin S, Aydin S. Plasma adropin levels predict endothelial dysfunction like flow-mediated dilatation in patients with type 2 diabetes mellitus. J Investig Med. 2013;61:1161–4.
Soltani S, Kolahdouz-Mohammadi R, Aydin S, Yosaee S, Clark CC, Abdollahi SJH. Circulating levels of adropin and overweight/obesity: a systematic review and meta-analysis of observational studies. 2021:1–8.
Celik E, Yilmaz E, Celik O, Ulas M, Turkcuoglu I, Karaer A, et al. Maternal and fetal adropin levels in gestational diabetes mellitus. J Perinat Med. 2013;41:375–80.
Kheirandish-Gozal L, Gileles-Hillel A, Alonso-Álvarez ML, Peris E, Bhattacharjee R, Terán-Santos J, et al. Effects of adenotonsillectomy on plasma inflammatory biomarkers in obese children with obstructive sleep apnea: a community-based study. Int J Obes. 2015;39:1094–100.
Metelli S, Chaimani AJE. -bmh. Challenges in meta-analyses with observational studies. 2020;23:83 – 7.
Acknowledgements
We sincerely thank Dr. Andrew Butler for all valuable comments and suggestions, which helped us to improve the quality of the article.
Authors would like to thank the electronic library of Shahid Sadoughi University of Medical Sciences to allow our group for performing more comprehensive search.
Funding
This work was funded by the North Khorasan University of Medical Sciences. The financial source had no role in the design of the study, described data management, analysis, reporting of study results, or decision to submit the manuscript for publication.
Author information
Authors and Affiliations
Contributions
SS conceived the study. SS and MM contributed in defining the search strategy. SS, SB, MM conducted the search, study selection and data extraction. SS conducted the statistical analyses. SB, SS, SA, AND CC wrote the first draft of the manuscript. SA critically revised the manuscript. All authors read and approved the final version of the manuscript. All authors reviewed the manuscript and provided final approval of the version to be published.
Corresponding author
Ethics declarations
Ethics approval and consent to participate
Not applicable.
Consent for publication
Not applicable.
Competing interests
The authors declare no competing interests.
Additional information
Publisher’s Note
Springer Nature remains neutral with regard to jurisdictional claims in published maps and institutional affiliations.
Rights and permissions
Open Access This article is licensed under a Creative Commons Attribution 4.0 International License, which permits use, sharing, adaptation, distribution and reproduction in any medium or format, as long as you give appropriate credit to the original author(s) and the source, provide a link to the Creative Commons licence, and indicate if changes were made. The images or other third party material in this article are included in the article's Creative Commons licence, unless indicated otherwise in a credit line to the material. If material is not included in the article's Creative Commons licence and your intended use is not permitted by statutory regulation or exceeds the permitted use, you will need to obtain permission directly from the copyright holder. To view a copy of this licence, visit http://creativecommons.org/licenses/by/4.0/. The Creative Commons Public Domain Dedication waiver (http://creativecommons.org/publicdomain/zero/1.0/) applies to the data made available in this article, unless otherwise stated in a credit line to the data.
About this article
Cite this article
Soltani, S., Beigrezaei, S., Malekahmadi, M. et al. Circulating levels of adropin and diabetes: a systematic review and meta-analysis of observational studies. BMC Endocr Disord 23, 73 (2023). https://doi.org/10.1186/s12902-023-01327-0
Received:
Accepted:
Published:
DOI: https://doi.org/10.1186/s12902-023-01327-0