Abstract
Background
Breast cancer patients undergoing chemotherapy treatment are at particular risk of experiencing acute cognitive impairment leading to daily challenges in decision-making and reduced quality of life and functional autonomy. The aim was to assess the relationship between clinical and genetic factors and cognitive function in a sample of patients with breast cancer undergoing chemotherapy.
Methods
A cross-sectional study was carried out between November 2017 and June 2019 on women (N = 112) treated for breast cancer by intravenous chemotherapy at the oncology outpatient unit of Hôtel-Dieu de France Hospital, Beirut. Patients were evaluated with the 37-item Functional Assessment of Cancer Therapy-Cognitive Function (FACT-Cog). Other validated scales were also used to assess depression, anxiety, sleep disorders, pain, and fatigue. DNA was obtained by a buccal swab (FTA®technology) for genotyping of different genes (ABCB1, COMT, DRD2, OPRM1, CLOCK, CRY2, and PER2) using the Lightcycler®(Roche).
Results
The mean age of participants was 56.04 years. Multivariable analysis, taking the four FACT-Cog subscores as the dependent variables, showed that the mean cognitive score decreased with higher depression, anxiety, and insomnia scores. Patients with university education levels had better perceived cognitive abilities than those with primary education. Moreover, carrying the G allele for the OPRM1 polymorphism (c.118A > G;rs197791) was significantly associated with a better cognitive function compared to AA patients (B = 2.05; p = 0.038).
Conclusions
A comprehensive oncological care plan should include a personalized assessment of all factors related to cognitive functioning in cancer patients, particularly anxiety and depression, to achieve an optimal patient outcome.
Similar content being viewed by others
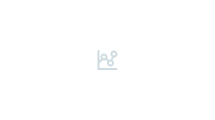
Background
Cancer-related cognitive impairment (CRCI) is defined as impaired memory, learning, concentration, executive function, visual-spatial abilities, and information processing [1,2,3]. Breast cancer patients undergoing chemotherapy treatment are at particular risk of experiencing acute cognitive impairment leading to daily challenges in decision-making, adherence to treatment, reduced quality of life and functional autonomy, and shorter survival rates [4, 5]. Hence, studies designed to evaluate cognition reported that up to 75% of women with breast cancer experience cognitive changes after a standard dose of chemotherapy [3, 6]. Several factors may account for the patients’ susceptibility to cognitive decline during a chemotherapy course, including number of therapy cycle [7], direct and indirect neurotoxicity due to treatment (progenitor cell and mitochondrial damage, immune system dysregulation) [8, 9], psychological disorders (anxiety, depression, sleep problems) [10,11,12], and genetic susceptibility [13,14,15]. Moreover, cancer-related fatigue, anxiety, depression, and insufficient sleep are associated with negative health outcomes, including impaired cognition [16,17,18]. As for the genetic aspect, most studies have focused on genes regulating neural repair/plasticity, such as the genes encoding the brain-derived neurotrophic factor (BDNF) [19] or apolipoprotein E (ApoE) [20]. However, other less frequently explored genetic factors might also contribute to chemotherapy-induced cognitive changes. These include the low efficiency of membrane efflux transporters at the blood–brain barrier (BBB) (such as P-glycoprotein or P-gp encoded by the ABCB1 gene) [21], impaired neurotransmission (via dopamine pathways, known for their implication in aging and neurocognition processing, especially in the prefrontal cortex [22]), dysregulation in the brain regions known to regulate learning and memory functions (opioid system) [23,24,25], or dysregulation in circadian rhythms [26].
The first selected single nucleotide polymorphism (SNP) in our study included the c.3435 T > C (rs1045642) in the ABCB1 gene encoding the P-gp. This substitution leads to a reduced P-pg expression and transport of xenobiotics to the central nervous system (CNS). Hence, patients with the T allele might have higher cognitive impairment due to a higher drug concentration in the brain, mainly because most breast cancer chemotherapy regimens have at least one cytotoxic drug that is a substrate of P-gp [27, 28]. Genes encoding the catechol-O-methyl transferase (COMT) and the dopamine receptor D2 (DRD2) were also explored. The SNP rs4680 in COMT is of particular interest: it causes a valine to methionine substitution (p.Val158 Met), leading to a 3- to fourfold reduced catabolic activity of COMT [29, 30]. Patients with the Met allele have a higher level of adrenaline in the prefrontal cortex (PFC) and increased dopamine binding to the D1 receptor, which enhances the stability of the dopaminergic PFC networks; these patients have a better performance in cognitive tasks (retaining information in the working memory) but a decreased cognitive flexibility while updating memory with new information [31]. As for DRD2, the SNP rs6277 (c.957C > T) causes a change in the mRNA folding, thus reducing the receptor stability and synthesis and the dopamine binding capacity in vivo and in vitro [32]. All these changes might lead to dysregulation in cognitive functioning in cancer patients. Despite the potential role of the opioid system in memory functions, the effects of SNPs in OPRM1 (gene encoding the Mu-opioid receptor) on cognitive function in breast cancer patients have been rarely explored. Our team was the first to describe in palliative cancer patients that homozygous AA patients for the SNP rs1799971 (c.118A > G) of OPRM1 had a significantly lower cognitive function than the AG patients [33]. Thus, it was decided to replicate this finding using a validated tool for cognitive function. Finally, several SNPs in genes of the circadian rhythm unexplored with cognition previously were evaluated in cancer patients: rs1801260 in CLOCK (Circadian Locomotor Output Cycles Kaput), rs934945 in PER2 (Period circadian regulator 2), and rs10838524 CRY2 (Cryptochrome circadian regulator 2). The hypothesis was that these SNPs would induce a loss of coherence between the components of this system, which might negatively affect cognitive function [26, 34, 35].
Therefore, this study aimed to explore cognitive impairment in a group of breast cancer patients undergoing a chemotherapy course (using a validated tool for cancer patients) and evaluate the contribution of clinical, psychological, and genetic factors underlying treatment-related cognitive decline.
Methods
Study design and patients
The CAGE-Cog is a cross-sectional study conducted at Hôtel-Dieu de France (HDF) Hospital between November 2017 and June 2019 to evaluate the impact of Clinical And Genetic factors on cognitive function in women with primary breast cancer treated by chemotherapy.
Inclusion criteria were: adult women (> 18 years old), primary diagnosis of breast cancer (Stage 0 to IV), to be scheduled to receive a course of chemotherapy (random cycle). Exclusion criteria were: patients who refused to participate in the study, concurrent radiation/adjuvant hormone therapy, relapsed breast cancer/other types of cancer, any surgical intervention/disorder of the central nervous system (dementia, multiple sclerosis, epilepsy, Parkinson's disease, intellectual disability, and neurosurgeries) that may affect cognitive function, brain metastasis (due to their known effect on the cognitive decline) [36,37,38].
Ethical approval
The ethics committee of HDF hospital approved this study (Reference: CEHDF1016, July 2017), and all patients gave their written consent prior to inclusion.
Sociodemographic and clinical information
Several demographic and clinical data were collected (using patient interview and medical records): age, gender, weight and height (to calculate the body mass index, BMI), Body Surface Area (BSA, calculated using the Mosteller formula) [39, 40], ethnicity/nationality, marital status, education level, socioeconomic level, presence of comorbidities (diabetes, hypertension, dyslipidemia, others), current alcohol consumption (self-reported), current tobacco smoking (self-reported), and medications (other than chemotherapy). Cancer-related clinical features were retrieved from the patients' medical records, including disease stage, location of metastases (if present), chemotherapy cycle number at the time of data collection, and chemotherapy regimen.
Cognitive function assessment and other assessments
Patients were interviewed on the day they were admitted to the oncology outpatient unit to receive chemotherapy (day 1 of chemotherapy, random cycle). The chemotherapy cycle number was recorded.
Cognitive function was assessed using the Functional Assessment of Cancer Therapy—Cognitive Function (FACT-Cog, version 3; Licensing agreement granted on November 2, 2017). This self-assessment scale consists of 37 questions divided into four subscales: 1) Perceived cognitive impairments subscale (CogPCI); 2) Perceived cognitive abilities subscale (CogPCA); 3) Comments from others subscale (CogOTH), referring to the comments of other people on cognitive function of the patient; and 4) Impact of perceived cognitive impairments on quality of life subscale (CogQOL), including disrupted normal daily functioning and working capacity. The higher the FACT-Cog score/subscore, the better the cognitive function of patients and the lower the impact on their quality of life [41].
Sleep disorders were evaluated using two screening tools, the Pittsburgh Sleep Quality Index (PSQI) and the insomnia severity index (ISI). The PSQI is a 19-item scale designed to measure sleep in seven domains during the past month: subjective quality of sleep, sleep latency, sleep duration, sleep efficiency, sleep disorders, sleep medication, and daytime dysfunction. The component scores range from 0 (no difficulty) to 3 (severe difficulty) and allow the calculation of an overall score ranging from 0 to 21 [42]. The ISI is a 7-item tool to assess the perceived severity of insomnia during the past two weeks. Questions are rated on a 5-point Likert scale from 0 (very satisfied) to 4 (not at all satisfied). Total scores range from 0 to 28, with the highest scores representing more severe insomnia [43].
Anxiety and depression were assessed using the Hospital Anxiety and Depression Scale (HADS). This self-report tool consists of two subscales designed to identify and quantify anxiety (HADS-A) and depression (HADS-D) in patients. Symptoms of the previous week are reported on a scale from 0 (not at all) to 3 (most of the time). Patients would be classified as “normal” (Score 0–7), having a “borderline anxiety/depression” (Score 8–10), or “clinical anxiety/depression” (Score 11–21) [44].
Fatigue was evaluated using three questions from the EORTC-QLQ C30 scale (European Organization for Research and Treatment of Cancer Quality of Life Questionnaire. QLQ C10: “Do you need rest?”; QLQ C12: “Did you feel weak?”; and QLQ C18: “Were you tired?”. A raw score was obtained by summing the responses to the three questions, then transformed into a score ranging from 0 to 100, according to the EORTC scoring manual. Higher scores indicated worse fatigue and a poorer QOL [45].
Finally, pain was estimated by the visual analogue scale (VAS), ranging from 0 (absence of pain) to 10 (maximum of pain) [46].
DNA Sampling and Genotyping
DNA was obtained by a buccal swab (Whatman® FTA® card technology-GE Healthcare) as recommended by the manufacturer. Genotyping for selected SNPs in ABCB1 (rs1045642), OPRM1 (rs1799971), COMT (rs4680), DRD2 (rs6277), CLOCK (rs1801260), PER2 (rs934945), and CRY2 (rs10838524) was performed using the Lightcycler® 2.0 (Roche Diagnostics GmbH-Mannheim-Germany).
Supplementary Table 1 shows the polymerase chain reaction (PCR) protocol and conditions. Positive (defined by direct sequencing) and negative controls (water) were systematically included in experiments.
Physicians and investigators at the hospital assessing cognitive function and other variables were not informed of the genotyping results prior to the end of the study to minimize potential bias.
Data and statistical analysis
The SPSS software version 25.0 was used for data analysis. Descriptive statistics were calculated for all variables in the study, including the mean and standard deviation for continuous measures and counts and percentages for categorical variables. Each of the cognitive sub-scores (CogPCI, CogPCA, CogOTH, CogQOL) was analyzed separately. The different cognitive scores did not follow a normal distribution (verified by the Kolmogorov–Smirnov test); therefore, nonparametric tests were used. The Kruskal–Wallis test was applied to compare several groups, the Mann–Whitney test to compare two groups, and the Spearman correlation test to compare two continuous variables. Multiple linear regressions were performed, taking each cognitive score as the dependent variable, respectively, and using variables that showed a p < 0.1 in the bivariate analysis as independent variables to reduce potential confounders. A value of p ≤ 0.05 was considered statistically significant.
Results
Sociodemographic and clinical characteristics
A total of 112 women with breast cancer were included in our study (mean age 56.04 ± 11.69 years and an average BMI of 25.9 ± 4.62 kg/m2). Among these patients, 8 had metastases and received palliative chemotherapy. Pain, as evaluated by the VAS, was relatively low, with a mean score of 1.68 ± 2.48 [0–9]. The average number of chemotherapy cycles was 4.45 ± 2.35 [1-12] (Table 1). More details are presented in Supplementary Table 2.
Bivariate analyses
Bivariate analyses were conducted with each FACT-Cog subscale score and the total instrument score as the dependent variable in the model (Table 2).
The results have shown that a significantly higher CogPCI score was found in patients who did not have diabetes compared to those who did. Patients taking adjuvant treatments (vs. palliative or neo-adjuvant regimens) had significantly higher CogOTH, QOL, and total scores. Patients who did not have metastases and those with the AG genotype for OPRM1 SNP (vs. AA) had a significantly higher CogQOL score (p = 0.005) (Table 2).
Higher insomnia severity, pain score, anxiety, and depression levels were significantly associated with lower CogPCI, whereas higher pain score was significantly associated with lower CogPCA scores. Higher anxiety and depression scores were significantly associated with higher CogOTH. Higher insomnia severity, worse sleep quality (higher PSQI scores), higher chemotherapy cycle number, higher pain scores, and higher anxiety and depression scores were significantly associated with lower Cog QOL and total FACT-Cog scores (Table 3).
Multivariable analysis
A first linear regression, taking the CogPCI score as the dependent variable, showed that participants with higher anxiety (B = -1.06) and those with diabetes (B = -8.94) had lower CogPCI scores (Table 4, Model 1).
A second forward linear regression, taking the CogPCA score as the dependent variable, showed that having a university education level (B = 2.75) was significantly associated with higher CogPCA scores (Table 4, Model 2).
A third linear regression, taking the CogOTH score as the dependent variable, showed that a higher depression level (B = -0.25) was significantly associated with lower CogOTH, whereas taking an adjuvant chemotherapeutic treatment (B = 1.65) vs. a palliative regimen was significantly associated with higher CogOTH scores (Table 4, Model 3).
A fourth linear regression, taking the CogQOL score as the dependent variable, showed that a higher depression (B = -0.24) and insomnia severity (ISI; B = -0.17) were significantly associated with lower CogQOL scores, whereas taking an adjuvant chemotherapeutic treatment (B = 3.21) vs. a palliative regimen and having the OPRM1 AG genotype vs. AA (B = 2.05) were significantly associated with higher CogQOL scores (Table 4, Model 4).
A fifth linear regression, taking the total FACT-Cog score as the dependent variable, showed that higher depression (B = -1.91) and taking a neo-adjuvant chemotherapeutic treatment (B = -13.80) vs. a palliative regimen were significantly associated with lower total FACT-Cog scores (Table 4, Model 5).
These results were adjusted over all the variables that showed a p-value of more than 0.1 in the bivariate analyses (including age and education usually controlled when considering cancer-related effects on cognition).
When forcing all the genes into each model, the results remained the same.
Discussion
Our study explored the clinical and genetic factors associated with cognitive impairment in patients with breast cancer. The total FACT-Cog and subdomain scores were similar to those reported in other studies among breast cancer patients [20, 47], but lower than those reported in other populations after the completion of chemotherapy [10], likely due to the relatively short period of evaluation of cognitive function since the beginning of chemotherapy treatment. Regarding the genetic factors, the rs1799971 of OPRM1 (c.118A > G) showed to be significantly associated with cognitive function; patients carrying the G variant had significantly higher CogQOL scores compared to AA patients. To the best of our knowledge, this study is the first to report such an association between the opioid system and cognitive function, using a validated tool for patient-reported (subjective) cognitive function in cancer patients. A similar finding had been previously reported in a palliative cancer group of patients but using a less specific tool for cognitive functioning (EORTC-QOL of the World Health Organization WHO) [33]. Previous studies have explored the association of dynorphin/κ-opioid receptor (κOR), but not the mu-opioid receptor, with cognitive function, showing its implication in emotion and cognition. Furthermore, it has been demonstrated that the pharmacological blockade of κOR prevented impairments of memory performance, whereas its activation was linked to cognitive decline in mice [24, 25]. Therefore, it was hypothesized that AA patients for OPRM1, exhibiting a greater activation of the mu-receptor, would have cognitive deficits as described with the activation of the κOR, explaining their lower cognitive performance and the subsequent impact on their quality of life. Future studies are necessary to validate these results in replication cohorts.
Besides the genetic factors, our study has shown that patients who have anxiety or depression report more cognitive impairments (lower CogPCI scores for anxiety. and lower CogOTH, CogQOL, and total FACT-Cog scores for depression). These results are in line with several previous reports showing a significant association between reported psychological distress and subjective/objective cognitive disturbances in cancer patients [10,11,12, 17, 48]. Indeed, psychological and emotional distress from cancer diagnosis and cancer-related treatments (chemotherapy, radiotherapy, or surgery) might cause changes in the hypothalamic-pituitary axis [49]. This hypothesis suggests that emotional states can trigger biological changes, such as the release of pro-inflammatory cytokines and biochemical alterations in neuronal plasticity, which leads to cognitive decline [8].
Another psychological factor that emerged in the multivariable analyses is the insomnia severity as evaluated by the insomnia severity index. The higher the ISI scores, the lower the CogQOL scores due to cognitive decline. This finding is similar to what was previously reported about the association between sleep disorders, cognitive dysfunction, and the detrimental impact on quality of life [50,51,52]. Interestingly, some researchers identified neuro-inflammatory processes [18, 53] and reduced hippocampal volumes in areas involved in working memory among patients with sleep disturbances [54].
Pain was significantly associated with cognition in the bivariate but not multivariable analyses. Previous studies exploring pain in cancer and non-cancer patients highlighted that patients reporting ongoing/persistent moderate to severe pain might be at risk for cognitive decline. A possible explanation for why this relevant factor did not remain significant in the multivariable analyses could be the relatively low pain levels reported in our study (mean VAS score of 1.68) or the relatively small sample size.
Hence, psychological disorders, pain, fatigue, sleep disorders, and cognitive dysfunction seem to be interrelated and might share a common pathophysiology, suggesting the concept of a “symptom cluster” [4, 16, 32, 43, 55].
Furthermore, patients with diabetes reported more cognitive impairment as described elsewhere [56, 57]. Several hypotheses have been suggested to explain the pathophysiology of diabetes-related cognitive dysfunction. In that context, it was found that insulin receptors are expressed in the neuronal soma and synaptic terminals, making them essential components for the preservation of memory in the hippocampus [56]. This metabolic disorder can cause a chronic inflammatory state and oxidative stress that affect neurons, inducing apoptosis and increasing cerebral damage [58]. These biochemical modifications could, therefore, potentially lead to cognitive impairments.
Having a university level of education was significantly associated with higher CogPCA scores. Several studies had previously reported the correlation between higher education and cognitive reserve [59,60,61]. Indeed, higher education is associated with better mental adaptation and superior coping skills following chemotherapy-induced brain damage, thus protecting against cognitive impairment [60]. A study about the impact of education level on cognitive function in breast cancer patients has reported that patients with higher education levels performed better on the verbal memory task than those who are less educated [61].
Finally, chemotherapy regimens were also associated with different subscores of the cognitive evaluation. Patients who were offered adjuvant chemotherapy following breast cancer surgery had better CogOTH/CogQOL scores than those who received a neoadjuvant or palliative regimen. A possible explanation for such a result could be the more severe/advanced cancer stages in patients who did not receive adjuvant chemotherapy.
Limitations and strengths
Our study has several limitations related to its design. In this cohort of patients, cognitive function was not evaluated at baseline before starting any initial treatment (chemotherapy/surgery). Therefore, it was not possible to detect changes over time, and the cognitive assessment could have been biased as some patients might exhibit cognitive impairment since the beginning of their treatment. Moreover, although a validated tool for cancer patients was used, cognition was still subjectively reported and not objectively measured using clinical batteries for objective neurocognitive functioning assessment. A selection bias is also possible since patients were recruited from one hospital. An information bias is also likely since symptoms of depression, anxiety, and insomnia might have been over or underestimated by patients. Residual confounding bias might also be present since not all factors associated with cognitive function were considered in this study.
Finally, the sample size is considered relatively small for genetic analyses. However, to the best of our knowledge, this study is the first to explore several clinical and genetic factors, including genes for the circadian rhythm, not previously evaluated with cognitive function. Furthermore, multivariable analyses were performed, taking into account all confounding factors.
Conclusion
Our findings highlight the importance of identifying clinical and genetic markers to improve the diagnosis of cognitive impairment and implement personalized medicine approaches to mitigate detrimental health outcomes in women with breast cancer. Patients with higher susceptibility to cognitive impairment could benefit from a personalized cognitive assessment as part of a comprehensive oncological supportive care plan. Management strategies, including physical activity/exercise, behavioral interventions, or other targeted pharmacological agents, that are still currently under investigation, would be interesting to implement once stronger evidence supporting their relevance is identified. Thus, further research on a larger sample is necessary to confirm our findings, allow generalization to the breast cancer population, and implement the adequate management plan.
Availability of data and materials
The datasets generated and/or analyzed during the current study are not publicly available due to the fact that the study is still ongoing on other cancer populations (other than breast cancer), but are available from the corresponding author on reasonable request.
Abbreviations
- ApoE:
-
Apolipoprotein E
- BBB:
-
Blood brain barrier
- BDNF:
-
Brain-derived neurotrophic factor
- BMI:
-
Body mass index
- BSA:
-
Body Surface Area
- CNS:
-
Central nervous system
- COMT:
-
Catechol-O-methyl transferase
- CogPCA:
-
Perceived cognitive abilities subscale
- CogPCI:
-
Perceived cognitive impairments subscale
- CogOTH:
-
Comments from others subscale
- CogQOL:
-
Impact of perceived cognitive impairments on quality of life
- EORTC-QLQ C30 scale:
-
European Organization for Research and Treatment of Cancer FACIT: Functional Assessment of Chronic Illness Therapy system of Quality of Life questionnaires
- FACT-Cog:
-
Functional Assessment of Cancer Therapy-Cognitive Function
- HADS-A:
-
Hospital Anxiety and Depression Scale; anxiety subscale
- HADS-D:
-
Hospital Anxiety and Depression Scale; depression subscale
- HDF:
-
Hôtel-Dieu de France
- ISI:
-
Insomnia severity index
- PCR:
-
Polymerase chain reaction
- PFC:
-
Prefrontal cortex
- P-gp:
-
P-glycoprotein
- PSQI:
-
Pittsburgh Sleep Quality Index
- SNP:
-
Single nucleotide polymorphism
- VAS:
-
Visual analogue scale
- WHO:
-
World Health Organization
References
Ahles TA, Root JC, Ryan EL. Cancer- and cancer treatment-associated cognitive change: an update on the state of the science. J Clin Oncol. 2012;30(30):3675–86.
Dietrich J, Prust M, Kaiser J. Chemotherapy, cognitive impairment and hippocampal toxicity. Neuroscience. 2015;309:224–32.
Vannorsdall TD. Cognitive Changes Related to Cancer Therapy. Med Clin North Am. 2017;101(6):1115–34.
Janz NK, Mujahid M, Chung LK, Lantz PM, Hawley ST, Morrow M, Schwartz K, Katz SJ. Symptom experience and quality of life of women following breast cancer treatment. J Womens Health (Larchmt). 2007;16(9):1348–61.
Alatawi Y, Hansen RA, Chou C, Qian J, Suppiramaniam V, Cao G. The impact of cognitive impairment on survival and medication adherence among older women with breast cancer. Breast Cancer. 2020;28(2):277–88.
Janelsins MC, Kesler SR, Ahles TA, Morrow GR. Prevalence, mechanisms, and management of cancer-related cognitive impairment. Int Rev Psychiatry. 2014;26(1):102–13.
Oh PJ. Predictors of cognitive decline in people with cancer undergoing chemotherapy. Eur J Oncol Nurs. 2017;27:53–9.
Cheung YT, Ng T, Shwe M, Ho HK, Foo KM, Cham MT, Lee JA, Fan G, Tan YP, Yong WS, et al. Association of proinflammatory cytokines and chemotherapy-associated cognitive impairment in breast cancer patients: a multi-centered, prospective, cohort study. Ann Oncol. 2015;26(7):1446–51.
Gaman AM, Uzoni A, Popa-Wagner A, Andrei A, Petcu EB. The Role of Oxidative Stress in Etiopathogenesis of Chemotherapy Induced Cognitive Impairment (CICI)-“Chemobrain.” Aging Dis. 2016;7(3):307.
Janelsins MC, Heckler CE, Peppone LJ, Kamen C, Mustian KM, Mohile SG, Magnuson A, Kleckner IR, Guido JJ, Young KL, et al. Cognitive Complaints in Survivors of Breast Cancer After Chemotherapy Compared With Age-Matched Controls: An Analysis From a Nationwide, Multicenter. Prospective Longitudinal Study J Clin Oncol. 2017;35(5):506–14.
Berman MG, Askren MK, Jung M, Therrien B, Peltier S, Noll DC, Zhang M, Ossher L, Hayes DF, Reuter-Lorenz PA, et al. Pretreatment worry and neurocognitive responses in women with breast cancer. Health Psychol. 2014;33(3):222–31.
Von Ah D, Tallman EF. Perceived Cognitive Function in Breast Cancer Survivors: Evaluating Relationships With Objective Cognitive Performance and Other Symptoms Using the Functional Assessment of Cancer Therapy—Cognitive Function Instrument. J Pain Symptom Manage. 2015;49(4):697–706.
Cheng H, Li W, Gan C, Zhang B, Jia Q, Wang K. The COMT (rs165599) gene polymorphism contributes to chemotherapy-induced cognitive impairment in breast cancer patients. Am J Transl Res. 2016;8(11):5087–97.
Ng T, Lee YY, Chae JW, Yeo AHL, Shwe M, Gan YX, Ng RCH, Chu PPY, Khor CC, Ho HK, et al. Evaluation of plasma brain-derived neurotrophic factor levels and self-perceived cognitive impairment post-chemotherapy: a longitudinal study. BMC Cancer. 2017;17(1):867.
Park JY, Lengacher CA, Reich RR, Alinat CB, Ramesar S, Le A, Paterson CL, Pleasant ML, Park HY, Kiluk J, et al. Translational genomic research: the role of genetic polymorphisms in MBSR program among breast cancer survivors (MBSR[BC]). Transl Behav Med. 2019;9(4):693–702.
Xu S, Thompson W, Ancoli-Israel S, Liu L, Palmer B, Natarajan L. Cognition, quality-of-life, and symptom clusters in breast cancer: Using Bayesian networks to elucidate complex relationships. Psychooncology. 2018;27(3):802–9.
Park JH, Jung YS, Jung YM, Bae SH. The role of depression in the relationship between cognitive decline and quality of life among breast cancer patients. Support Care Cancer. 2019;27(7):2707–14.
Zhu B, Dong Y, Xu Z, Gompf HS, Ward SA, Xue Z, Miao C, Zhang Y, Chamberlin NL, Xie Z. Sleep disturbance induces neuroinflammation and impairment of learning and memory. Neurobiol Dis. 2012;48(3):348–55.
Ng T, Teo SM, Yeo HL, Shwe M, Gan YX, Cheung YT, Foo KM, Cham MT, Lee JA, Tan YP, et al. Brain-derived neurotrophic factor genetic polymorphism (rs6265) is protective against chemotherapy-associated cognitive impairment in patients with early-stage breast cancer. Neuro Oncol. 2016;18(2):244–51.
Lange M, Heutte N, Rigal O, Noal S, Kurtz JE, Levy C, Allouache D, Rieux C, Lefel J, Clarisse B, et al. Decline in Cognitive Function in Older Adults With Early-Stage Breast Cancer After Adjuvant Treatment. Oncologist. 2016;21(11):1337–48.
Ahles TA, Saykin AJ. Candidate mechanisms for chemotherapy-induced cognitive changes. Nat Rev Cancer. 2007;7(3):192–201.
Savitz J, Solms M, Ramesar R. The molecular genetics of cognition: dopamine. COMT and BDNF Genes Brain Behav. 2006;5(4):311–28.
Jamot L, Matthes HW, Simonin F, Kieffer BL, Roder JC. Differential involvement of the mu and kappa opioid receptors in spatial learning. Genes Brain Behav. 2003;2(2):80–92.
Bilkei-Gorzo A, Mauer D, Michel K, Zimmer A. Dynorphins regulate the strength of social memory. Neuropharmacology. 2014;77:406–13.
Carey AN, Lyons AM, Shay CF, Dunton O, McLaughlin JP. Endogenous kappa opioid activation mediates stress-induced deficits in learning and memory. J Neurosci. 2009;29(13):4293–300.
Heyde I, Kiehn JT, Oster H. Mutual influence of sleep and circadian clocks on physiology and cognition. Free Radic Biol Med. 2018;119:8–16.
Clarke R, Leonessa F, Trock B. Multidrug resistance/P-glycoprotein and breast cancer: review and meta-analysis. Semin Oncol. 2005;32(6 Suppl 7):S9-15.
Zhou SF. Structure, function and regulation of P-glycoprotein and its clinical relevance in drug disposition. Xenobiotica. 2008;38(7–8):802–32.
Qin X, Peng Q, Qin A, Chen Z, Lin L, Deng Y, Xie L, Xu J, Li H, Li T, et al. Association of COMT Val158Met polymorphism and breast cancer risk: an updated meta-analysis. Diagn Pathol. 2012;7:136.
Small BJ, Rawson KS, Walsh E, Jim HS, Hughes TF, Iser L, Andrykowski MA, Jacobsen PB. Catechol-O-methyltransferase genotype modulates cancer treatment-related cognitive deficits in breast cancer survivors. Cancer. 2011;117(7):1369–76.
Schacht JP. COMT val158met moderation of dopaminergic drug effects on cognitive function: a critical review. Pharmacogenomics J. 2016;16(5):430–8.
Bradberry JC, Droege M, Evans RL, Guglielmo J, Knapp D, Knapp K, Meyer S, Poirier T, Plaza C. Curricula Then and Now—An Environmental Scan and Recommendations Since the Commission to Implement Change in Pharmaceutical Education: Report of the 2006–2007 Academic Affairs Committee. Am J Pharm Educ. 2007;71(Suppl):S10.
Hajj A, Halepian L, Osta NE, Chahine G, Kattan J, Rabbaa Khabbaz L. OPRM1 c.118A>G Polymorphism and Duration of Morphine Treatment Associated with Morphine Doses and Quality-of-Life in Palliative Cancer Pain Settings. Int J Mol Sci. 2017;18(4):669.
Valdez P. Circadian Rhythms in Attention. Yale J Biol Med. 2019;92(1):81–92.
Waterhouse J. Circadian rhythms and cognition. Prog Brain Res. 2010;185:131–53.
Noh T, Walbert T. Brain metastasis: clinical manifestations, symptom management, and palliative care. Handb Clin Neurol. 2018;149:75–88.
Lee PE, Tierney MC, Wu W, Pritchard KI, Rochon PA. Endocrine treatment-associated cognitive impairment in breast cancer survivors: evidence from published studies. Breast Cancer Res Treat. 2016;158(3):407–20.
Wagner LI, Gray RJ, Sparano JA, Whelan TJ, Garcia SF, Yanez B, Tevaarwerk AJ, Carlos RC, Albain KS, Olson JA Jr, et al. Patient-Reported Cognitive Impairment Among Women With Early Breast Cancer Randomly Assigned to Endocrine Therapy Alone Versus Chemoendocrine Therapy: Results From TAILORx. J Clin Oncol. 2020;38(17):1875–86.
Medscape, Body Surface Area Based Dosing, last assessed May 1, 2020 at: https://reference.medscape.com/calculator/bsa-dosing
Verbraecken J, Van de Heyning P, De Backer W, Van Gaal L. Body surface area in normal-weight, overweight, and obese adults. A comparison study Metabolism. 2006;55(4):515–24.
Lange M, Heutte N, Morel N, Eustache F, Joly F, Giffard B. Cognitive complaints in cancer: The French version of the Functional Assessment of Cancer Therapy-Cognitive Function (FACT-Cog), normative data from a healthy population. Neuropsychol Rehabil. 2016;26(3):392–409.
Akman T, Yavuzsen T, Sevgen Z, Ellidokuz H, Yilmaz AU. Evaluation of sleep disorders in cancer patients based on Pittsburgh Sleep Quality Index. Eur J Cancer Care (Engl). 2015;24(4):553–9.
Gehrman PR, Garland SN, Matura LA, Mao J. Insomnia in breast cancer: Independent symptom or symptom cluster? Palliat Support Care. 2017;15(3):369–75.
Hartung TJ, Friedrich M, Johansen C, Wittchen HU, Faller H, Koch U, Brahler E, Harter M, Keller M, Schulz H, et al. The Hospital Anxiety and Depression Scale (HADS) and the 9-item Patient Health Questionnaire (PHQ-9) as screening instruments for depression in patients with cancer. Cancer. 2017;123(21):4236–43.
Mierzynska J, Taye M, Pe M, Coens C, Martinelli F, Pogoda K, Velikova G, Bjelic-Radisic V, Cardoso F, Brain E, et al. Reference values for the EORTC QLQ-C30 in early and metastatic breast cancer. Eur J Cancer. 2020;125:69–82.
Ovayolu O, Ovayolu N, Aytac S, Serce S, Sevinc A. Pain in cancer patients: pain assessment by patients and family caregivers and problems experienced by caregivers. Support Care Cancer. 2015;23(7):1857–64.
Park JH, Bae SH, Jung YS, Jung YM. The psychometric properties of the Korean version of the functional assessment of cancer therapy-cognitive (FACT-Cog) in Korean patients with breast cancer. Support Care Cancer. 2015;23(9):2695–703.
Bedillion MF, Ansell EB, Thomas GA. Cancer treatment effects on cognition and depression: The moderating role of physical activity. Breast. 2019;44:73–80.
Young EA, Abelson JL, Cameron OG. Effect of comorbid anxiety disorders on the hypothalamic-pituitary-adrenal axis response to a social stressor in major depression. Biol Psychiatry. 2004;56(2):113–20.
Walker WH 2nd, Borniger JC. Molecular Mechanisms of Cancer-Induced Sleep Disruption. Int J Mol Sci. 2019;20(11):2780.
Palesh O, Aldridge-Gerry A, Ulusakarya A, Ortiz-Tudela E, Capuron L, Innominato PF. Sleep disruption in breast cancer patients and survivors. J Natl Compr Canc Netw. 2013;11(12):1523–30.
Liou KT, Ahles TA, Garland SN, Li QS, Bao T, Li Y, Root JC, Mao JJ: The Relationship Between Insomnia and Cognitive Impairment in Breast Cancer Survivors. JNCI Cancer Spectrum 2019, 3(3):pkz041.
van Dalfsen JH, Markus CR. The influence of sleep on human hypothalamic–pituitary–adrenal (HPA) axis reactivity: A systematic review. Sleep Med Rev. 2017;39:187–94.
Chee MW, Chuah LY, Venkatraman V, Chan WY, Philip P, Dinges DF. Functional imaging of working memory following normal sleep and after 24 and 35 h of sleep deprivation: Correlations of fronto-parietal activation with performance. Neuroimage. 2006;31(1):419–28.
Ho SY, Rohan KJ, Parent J, Tager FA, McKinley PS. A longitudinal study of depression, fatigue, and sleep disturbances as a symptom cluster in women with breast cancer. J Pain Symptom Manage. 2015;49(4):707–15.
Zilliox LA, Chadrasekaran K, Kwan JY, Russell JW. Diabetes and Cognitive Impairment. Curr Diab Rep. 2016;16(9):87.
Black S, Kraemer K, Shah A, Simpson G, Scogin F, Smith A. Diabetes, Depression, and Cognition: a Recursive Cycle of Cognitive Dysfunction and Glycemic Dysregulation. Curr Diab Rep. 2018;18(11):118.
Rojas-Carranza CA, Bustos-Cruz RH, Pino-Pinzon CJ, Ariza-Marquez YV, Gomez-Bello RM, Canadas-Garre M. Diabetes-Related Neurological Implications and Pharmacogenomics. Curr Pharm Des. 2018;24(15):1695–710.
Van Arsdale A, Rosenbaum D, Kaur G, Pinto P, Kuo DY, Barrera R, Goldberg GL, Nevadunsky NS. Prevalence and factors associated with cognitive deficit in women with gynecologic malignancies. Gynecol Oncol. 2016;141(2):323–8.
Rimkus CM, Avolio IMB, Miotto EC, Pereira SA, Mendes MF, Callegaro D, Leite CDC. The protective effects of high-education levels on cognition in different stages of multiple sclerosis. Mult Scler Relat Disord. 2018;22:41–8.
Perrier J, Viard A, Levy C, Morel N, Allouache D, Noal S, Joly F, Eustache F, Giffard B. Longitudinal investigation of cognitive deficits in breast cancer patients and their gray matter correlates: impact of education level. Brain Imaging Behav. 2020;14(1):226–41.
Acknowledgements
The authors would like to thank all physicians and students (namely Tamara Nehmé, Bashar ElJebbawi, Christina Chemaly) who helped recruit patients at Hôtel-Dieu de France Hospital (Beirut, Lebanon), and Dr. David Cella for granting permission to use the FACT-Cog (Licensing agreement granted on November 2, 2017). This work was supported by the financial support of the Saint-Joseph University (Conseil de la recherche: FPH71).
Funding
This work was supported by the “Conseil de la recherche” of the Saint-Joseph University of Beirut (FPH71).
Author information
Authors and Affiliations
Contributions
Aline HAJJ: Conceptualization, Data curation, Funding acquisition, Investigation, Methodology, Project administration, Writing—original draft. Rita KHOURY: Data curation, Investigation, Methodology, Writing—original draft. Roula HACHEM: Data curation, Investigation, Methodology, Writing—original draft. Aya AWAD: Investigation. Souheil HALLIT: Formal analysis, Software, Writing—review & editing. Hala SACRE: Writing—review & editing. Fady NASR: Investigation. Fadi El KARAK: Investigation. Georges CHAHINE: Investigation. Joseph KATTAN: Supervision, Investigation, Writing—review & editing. Lydia RABBAA KHABBAZ: Supervision, Writing—review & editing. The author(s) read and approved the final manuscript.
Corresponding author
Ethics declarations
Ethics approval and consent to participate
All experimental protocols were approved by Hôtel-Dieu de France Hospital ethical committee (HDF, CEHDF1016, July 2017). All methods were carried out in accordance with relevant guidelines and regulations. Participants were fully informed of the purpose and procedures of the study and had the adequate time to ask questions and ponder about their voluntary participation. A written informed consent was obtained from all patients before enrollment.
Consent for publication
Not applicable.
Competing interests
The authors have no conflicts of interest to disclose.
Additional information
Publisher’s Note
Springer Nature remains neutral with regard to jurisdictional claims in published maps and institutional affiliations.
Supplementary Information
Additional file 1:
Supplementary Table 1. The PCR protocol and conditions for CLOCK, PER2, CRY2, COMT, DRD2, OPRM1, and ABCB1 genotyping. Supplementary Table 2. Patients’ demographics and clinical characteristics (N = 112)*.
Rights and permissions
Open Access This article is licensed under a Creative Commons Attribution 4.0 International License, which permits use, sharing, adaptation, distribution and reproduction in any medium or format, as long as you give appropriate credit to the original author(s) and the source, provide a link to the Creative Commons licence, and indicate if changes were made. The images or other third party material in this article are included in the article's Creative Commons licence, unless indicated otherwise in a credit line to the material. If material is not included in the article's Creative Commons licence and your intended use is not permitted by statutory regulation or exceeds the permitted use, you will need to obtain permission directly from the copyright holder. To view a copy of this licence, visit http://creativecommons.org/licenses/by/4.0/. The Creative Commons Public Domain Dedication waiver (http://creativecommons.org/publicdomain/zero/1.0/) applies to the data made available in this article, unless otherwise stated in a credit line to the data.
About this article
Cite this article
Hajj, A., Khoury, R., Hachem, R. et al. Clinical and genetic factors associated with self-reported cognitive deficits in women with breast cancer: the “CAGE-Cog” study. BMC Cancer 22, 996 (2022). https://doi.org/10.1186/s12885-022-10077-6
Received:
Accepted:
Published:
DOI: https://doi.org/10.1186/s12885-022-10077-6