Abstract
To adapt to each other, plants and insect herbivores have developed sophisticated molecular interactions. Here, we summarize current knowledge about such molecular interactions between rice, a globally important food crop, and insect herbivores. When infested by insect herbivores, rice perceives herbivore- and/or damage-associated molecular patterns (HAMPs/DAMPs) via receptors that activate early signaling events such as the influx of Ca2+, the burst of reactive oxygen species, and the activation of MPK cascades. These changes result in specific rice defenses via signaling networks that mainly include phytohormones (jasmonic acid, salicylic acid, ethylene, and abscisic acid) and transcription factors. Some compounds, including flavonoids, phenolamides, defensive proteins, and herbivore-induced rice volatiles, have been reported to be used by rice against insects. Insect herbivores can deliver effectors or factors to inhibit rice defenses or enhance rice susceptibility. Although the number of HAMPs and defense-suppressing effectors from rice piercing-sucking insects has increased rapidly, none from rice chewing insects has been identified. Moreover, herbivore effectors or factors that induce rice susceptibility, and rice immune receptors recognizing HAMPs or effectors, are not well characterized. We point out future research directions in this area and highlight the importance of elucidating the mechanisms for rice sensing of insect herbivores and for insect counter-defenses against plants.
Similar content being viewed by others
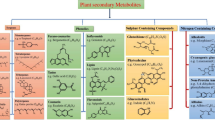
Avoid common mistakes on your manuscript.
Introduction
Over millions of years of coevolution, plants and herbivorous insects have engaged in an ongoing battle involving diverse mechanisms. Plants employ constitutive defenses to prevent herbivore infestation; when attacked by insect herbivores, plants activate induced defenses by perceiving herbivore- and/or damage-associated molecular patterns (HAMPs/DAMPs) [1, 2]. These induced defenses are produced locally and systemically, and may influence the performance of conspecific and non-conspecific herbivores that share the same host plant, either simultaneously or successively, directly and/or indirectly, by attracting the natural enemies of insect herbivores [3,4,5]. In response to plants, insect herbivores have evolved various counter-defense strategies, such as secreting effectors or factors to inhibit plant defenses or enhance plant susceptibility, and detoxifying or accommodating plant defensive compounds [4, 6, 7]. These molecular interactions between plants and insect herbivores largely determine the consequences of plant-herbivore interactions that are observable at the macroscopic level: the plant either resists or is susceptible to the herbivore, which in turn affects the population density and diversity of both plants and insect herbivores. Therefore, elucidating the molecular interactions between plants and insect herbivores will deepen understanding of their interactions and also provide both a theoretical and a technical basis for new ways of managing insect pests.
Rice (Oryza sativa) is a crucial food crop and the primary food source for more than 50% of the world’s population. However, yield is severely threatened by various insect pests; there are three main groups of these insect pests: rice planthoppers – the brown planthopper (BPH, Nilaparvata lugens), white-backed planthopper (WBPH, Sogatella furcifera), and small brown planthopper (SBPH, Laodelphax striatellus); rice borers – the striped stem borer (SSB, Chilo suppressalis) and yellow stem borer (Scircophaga incertulas); and the rice leaf folder (LF, Cnaphalocrocis medinalis) [8, 9]. Thus far, the molecular interactions of rice with insect herbivores, especially BPH and SSB, have been extensively studied; elicitors and effectors derived from herbivores have been identified, as have signaling networks related to rice defenses and their roles, and rice defensive compounds and the mechanisms underlying their biosynthesis. In this paper, we will summarize the latest literature and identify future research directions, especially those that promote environmentally friendly pest control techniques.
The defenses of rice in response to insect-herbivore infestation
When infested by herbivores, rice plants, like many other plant species, rapidly employ specific immune receptors—pattern recognition receptors (PRRs)—to perceive HAMPs/DAMPs and then initiate early signaling events [9, 10]. These events include the influx of calcium ions (Ca2+), a burst of reactive oxygen species (ROS), and the activation of mitogen-activated protein kinase (MPK) cascades [10,11,12,13]. Early events trigger the activation of transcription factors such as WRKYs and signaling pathways mediated by phytohormones, primarily jasmonic acid (JA), salicylic acid (SA), abscisic acid (ABA), and ethylene (ET) [14,15,16,17,18,19]. Subsequently, extensive reprogramming of the plant transcriptome, proteome, and metabolome occurs; both direct and indirect resistance of rice to herbivores results from this reprogramming [20,21,22,23,24,25,26] (Fig. 1).
Current model of the molecular interactions between rice and herbivores. When infested by herbivores, rice plants rapidly and specifically employ pattern recognition receptors (PRRs) to perceive herbivore/damage-associated molecular patterns (HAMPs/DAMPs), initiating early signaling events. These early signaling events include the influx of calcium ions (Ca2+), the burst of reactive oxygen species (ROS), and the activation of mitogen-activated protein kinase (MPK) cascades. Additionally, they trigger the activation of transcription factors such as WRKYs and signaling pathways mediated by phytohormones, including jasmonic acid (JA), salicylic acid (SA), abscisic acid (ABA), ethylene (ET), gibberellins (GAs), and nitric oxide (NO). Subsequently, extensive reprogramming of the plant transcriptome, proteome, and metabolome occurs, leading to both direct and indirect resistance to rice insect pests. To cope with plant defense, adapted herbivores also secrete a repertoire of effectors or factors to suppress plant defenses or enhance the susceptibility of rice plants. However, some plants with specific resistance genes may be able to activate effector-triggered immunity by recognizing effectors, which in turn improves the ability of rice to resist herbivores. Details of the known immune receptors, HAMPs, DAMPs, effectors and signaling components are provided in the text. LRR-RLK, leucine-rich repeat receptor-like kinase; LecRK, lectin receptor-like kinase; PEPR, plant elicitor peptide (Pep) receptor; RLCK, receptor-like cytoplasmic kinase; BSR1, broad-spectrum resistance 1; RBOH, respiratory burst oxidase homologous protein; MKK, MPK kinase; MKKK, MKK kinase; PLCP, papain-like cysteine protease; NBR1, a selective autophagy cargo receptor; ATG8, autophagy associated gene 8; TrypPI, trypsin proteinase inhibitor; VgN,N-terminal subunit of vitellogenin; MLP, mucin-like protein; PDI, disulfide isomerase; BISP, BROWN PLANTHOPPER 14-interacting salivary protein; VgC, C-terminal subunit of vitellogenin; EG1, endo-β-1,4-glucanase; SEF1, salivary EF-hand calcium-binding protein1; ECP1, EF-hand calcium-binding protein 1; SP84, salivary protein 84; Os, Oryza sativa; Nl, Nilaparvata lugens; Ls, Laodelphax striatellus; Nc, Nephotettix cincticeps
HAMPs and DAMPs
The initiation of an effective plant defense response relies on the plant’s ability to accurately recognize signals related to herbivores [7, 27]. These signals include DAMPs and HAMPs. DAMPs are compounds derived from plants when they are mechanically damaged or infested by insect herbivores; these compounds may be plant cell wall fragments, phytocytokines or small secondary metabolite derivatives [7, 27, 28]. HAMPs, also referred to as elicitors, are compounds derived from insect herbivores that enter plant tissues and induce defenses in plants [1, 6, 7]. Unlike elicitors, effectors are compounds secreted by insect herbivores to suppress plant defenses [2]. However, some plants with specific resistance genes may be able to activate effector-triggered immunity by recognizing effectors in turn, leading to plant resistance to herbivores [2, 29]. Therefore, effectors can also be considered as elicitors [2].
Thus far, scientists have identified various HAMPs from oral secretions (saliva and regurgitant fluids), excreta (frass or honeydew), eggs, and oviposition-associated secretions of insect herbivores, such as fatty acid‒amino acid conjugates (FACs) [30], caeliferins [31], inceptins [32], bruchins [33], benzyl cyanide [34], phosphatidylcholines [35] and an annexin-like protein [36]. These elicitors, which are mostly herbivore-specific, tend to induce specific defense responses in plants [37]. In rice herbivores, all identified HAMPs are from rice planthoppers (Table 1). By activating defense-related signaling pathways and inducing the accumulation of defensive compounds in plants, these HAMPs enhance plant herbivore resistance. The four salivary proteins of planthoppers—NlG14 [38], a mucin-like protein (NlMLP) [39], a DNAJ protein (NlDNAJB9) [40], and a disulfide isomerase (LsPDI1) [41], for example—may lead to any of the following: the activation of calcium signaling; MPK cascades and signaling pathways mediated by ROS and JA signaling; the deposition of callose; and the death of cells in the leaves of tobacco plants (Nicotiana benthamiana) when plants express one of these elicitor genes. Additionally, four phospholipids released by gravid WBPH females can induce the production of the ovicidal compound benzyl benzoate in rice plants; this compound eliminates WBPH eggs [42]. A recent study found that the N-terminal subunit of vitellogenins (VgN) of rice planthoppers, which is present in saliva and on the egg surface, is able to reach rice tissues, where it triggers Ca2+ influx and enhances the levels of hydrogen peroxide (H2O2), JA, and JA-isoleucine (JA-Ile) when the insects are feeding or during oviposition; this ability suggests that VgN reliably elicits rice defenses [17].
Interestingly, some microbes in BPH honeydews also induce the accumulation of phytoalexins and the release of volatiles from plants, thereby enhancing the resistance of rice to the insect [43]. These results imply that, in addition to perceiving HAMPs directly from insect herbivores, plants can also perceive these compounds from herbivore-associated microbes and, in response, initiate defenses. Other rice insect herbivores, such as SSB, Parnara guttata, Mythimna loreyi, Spodoptera frugiperda, Spodoptera mauritia, and Nephotettix bipunctatus, have also been reported to activate strong defense responses in rice [44, 59,60,61,62]. However, no HAMPs from these insect herbivores have been identified.
In addition to HAMPs, DAMPs also play an important role in regulating plant defenses [28, 63]. Thus far, many DAMPs have been identified from host plants, and their roles in mediating plant-herbivore interactions have also been extensively studied, especially those of phytocytokines, systemin [64] and plant elicitor peptides (Peps) [65]. Systemin, an 18-amino acid peptide that is cleaved from precursor prosystemins, specifically activates local and systemic defense responses in plants of the Solanaceae by regulating the JA-signaling pathway and the biosynthesis of trypsin proteinase inhibitors (TrypPIs) [28]. Peps, cleaved from the precursor PROPEPs, were initially thought to be defense signals that amplify immunity in Arabidopsis. Further studies found that peps are also present in Gramineous, Solanaceous, and Fabaceous plants, such as rice, Zea mays, Solanum lycopersicum, and Glycine max [45, 65,66,67]. In rice, two DAMPs, OsPep3 [45, 67] and a class of oligosaccharides [46], have been identified (Table 1). Both treatment with M. loreyi oral secretions and BPH infestation significantly induced the expression of the OsPep3 precursor gene OsPROPEP3 in rice; moreover, the exogenous application of OsPep3 amplified herbivore-induced rice defense responses, including the activation of OsMPK3/6 and the production of ROS, JA-Ile, and defensive compounds (p-coumaroylputrescine, feruloyl putrescine, momilactone A and momilactone B). Overall, the exogenous application of OsPep3 increases plant resistance to both insect pests and pathogens [45, 67]. Recently, oligosaccharides—the hydrolysates of the Poaceae-specific secondary metabolite mixed-β-1,3;1,4-D-glucans (MLGs)—have been found to contribute to the ability of rice to resist BPH; the infestation of rice by this species not only promotes the hydrolysis of MLGs into oligosaccharides but also induces the binding of OsMYC2, a key transcription factor of JA signaling, to the promoter of the MLG biosynthesis-related gene OsCsIF6, thereby increasing the production of MLGs and extending rice defense responses [46].
Plant immune receptors
Plants use different immune receptors to monitor infection/infestation signals in both extracellular and intracellular compartments. These immune receptors include plasma membrane-localized PRRs, which mainly perceive extracellular signals, such as HAMPs, and intracellular resistance (R) proteins, which mainly perceive intracellular signals, such as effectors [2, 29, 68, 69] (Fig. 1).
Plasma membrane-localized PRRs
Plasma membrane-localized PRRs are categorized into receptor-like kinases (RLKs) or receptor-like proteins (RLPs) [2, 28, 29, 68]. RLKs typically comprise a ligand-binding extracellular domain, a transmembrane domain, and a cytoplasmic kinase domain, whereas RLPs lack a cytoplasmic kinase domain [29, 68]. After PRRs perceive a ligand, they associate with co-receptors, such as somatic embryogenesis receptor kinases (SERKs), and activate downstream signaling events [7, 27, 29, 68]. To date, only one leucine-rich repeat receptor-like protein (LRR-RLP) in plants has been identified as a PRR that perceives HAMPs. This PRR, known as inceptin receptor (INR), is present in plants of the common bean, Vigna unguiculata, and related legumes, and recognizes inceptin, a HAMP from the oral secretions of Lepidopteran caterpillars [70]. INR constitutively interacts with coreceptors, such as the brassinosteroid insensitive 1-associated kinase 1 (BAK1) (one of the SERKs), and binds to the adaptor RLK, suppressor of BAK1-interacting receptor-like kinase 1 (SOBIR1); subsequently, the INR-containing complex activates the following defense responses, such as enhancing the levels of ROS, ET, peroxidases, and TrypPIs, and then confers the ability to resist insect herbivores on plants [70]. In rice, a leucine-rich repeat receptor-like kinase (OsLRR-RLK1) has been identified as a putative plant PRR that might perceive HAMPs from SSB larvae. Remarkably, silencing OsLRR-RLK1 has been shown to impair the level of SSB-induced OsMPK3/6 phosphorylation and decrease SSB-elicited, but not wounding-elicited, levels of JA, ET, and TrypPI activity and the resistance of rice to SSB [10]. Notably, using multiple forward genetics approaches, an LRR-RLK ZmFACS (a homolog of OsLRR-RLK1) in Z. mays was identified as a candidate PRR responding to Gln-18:3, a typical FAC isolated from Lepidoptera larval oral secretions [71]. Considering that ZmFACS-mediated plant antiherbivore defenses are similar to the defenses mediated by OsLRR-RLK1 in rice, it is possible that both ZmFACS and OsLRR-RLK1 function as PRRs, sensing specific FACs. The association of direct receptors with FACs has not yet been demonstrated.
Unlike the study of HAMP-recognizing PRRs, which is quite new, the study of DAMP-recognizing PRRs has made great progress. Thus far, several DAMP-recognizing PRRs, such as those that recognize systemin [72] and those that recognize Peps [73,74,75], and their underlying mechanisms have been reported. AtPEPR1 in Arabidopsis thaliana, for instance, interacts with AtPep1 ~ 6 and mainly controls the activation of foliar AtPep signaling, whereas AtPEPR2 binds AtPep1/2 and modulates AtPep signaling in plant roots [73,74,75]. Although infestation by S. littoralis larvae strongly enhances the transcript levels of AtPEPR1, AtPEPR2, and AtPEOPEP3 in Arabidopsis, pepr1 pepr2 double mutant plants are highly insensitive to Peps and display a reduced defense response and less resistance to S. littoralis [75]. In rice, the overexpression of OsPEPR1 further enhances rice defenses against M. loreyi induced by wounding and treatment with OsPep3 [67]. In contrast, OsPep3-elicited rice defenses against BPH are abolished when OsPEPR1/2 is knocked out [45]. This research suggested that the Pep-PEPR ligand-receptor signal module is conserved across different plant species and imparts plant resistance when DAMPs are sensed. Additionally, Bph3, a gene cluster comprising three genes encoding lectin receptor-like kinases (OsLecRK1/2/3), was identified as the first potential RLK gene contributing to rice defense against BPH [76]. A recent study revealed that MLG-derived oligosaccharides directly bind to OsLecRK1 and OsLecRK3, thereby enhancing OsLecRK activity [46]; in contrast, oligosaccharide-induced rice resistance to BPH in the MLG biosynthesis gene (OsCsIF6)-overexpressing rice plants is nullified when OsLecRK1/3 is knocked out [46]. This finding suggested that OsLecRK1/3 functions similarly to PRRs in rice plants: it perceives MLG-derived oligosaccharides and initiates oligosaccharides-triggered rice defenses.
Intracellular R proteins
When plants defend against HAMPs, adapted insect herbivores can employ effectors to overcome these defenses (see section ‘Regulation of rice defenses and susceptibility by insect herbivores’). Furthermore, some plants develop intracellular immune receptors, also known as R proteins, to detect these effectors and thus initiate effector-triggered defenses [2, 29, 68, 69]. The largest group of intracellular R proteins belong to the nucleotide-binding site leucine-rich repeat proteins (NB-LRRs, also termed NLRs) [2, 69]. NLRs contain three distinct domains: a nucleotide-binding (NB-ARC) domain, a C-terminal leucine-rich repeat (LRR) domain, and an N-terminal extension domain [69]. Several R genes encoding intracellular NLRs conferring plant resistance to root-knot nematodes, such as PcMa from Prunus cerasifera [77], PsoRPM2 from Prunus sogdiana [78], AtDSC1 from Arabidopsis [79], and SlMi from Solanum lycopersicum [80], have been cloned.
In rice, two intracellular R proteins that confer the ability to resist BPH infection in rice have been cloned and characterized; these R genes include Bph14 and Bph9 (with alleles Bph1, Bph2, Bph7, Bph10, Bph18, Bph21, and Bph26) [81,82,83,84,85,86]. Among these R proteins, BPH14 activates ROS and SA-mediated signaling pathways, leading to an increase in the production of TrypPIs; moreover, the coiled-coil (CC) and NB-ARC domains of BPH14 interact with and stabilize OsWRKY46 and OsWRKY72, promoting the expression of callose synthase genes, enhancing callose deposition, and eventually reinforcing rice resistance [81, 84]. Significantly, a recent study reported a vital role of BPH14 in sensing BPH effectors and mediating trade-offs between rice growth and defense [85]. BPH secretes the effector BPH14-interacting salivary protein (BISP) into rice plants during feeding. In susceptible plants, BISP targets a receptor-like cytoplasmic kinase (RLCK), OsRLCK185, and inhibits the autophosphorylation of OsRLCK185, thereby decreasing basal defenses in rice. In Bph14-carrying resistant plants, BPH14 directly binds to BISP, activating effector-triggered rice defenses but inhibiting rice growth. Notably, the BISP-BPH14 module also binds to the selective autophagic cargo receptor OsNBR1. As insects cease feeding and depart from these sites, the activated autophagy pathway rapidly degrades BISP, thereby maintaining an appropriate level of BISP-BPH14 module-triggered rice defenses and restoring rice growth [85]. This study elucidates the molecular mechanism underlying the trade-offs in rice between defense and growth in the BISP-BPH14-OsNBR1 module. Additionally, BPH9 confers resistance to BPH in rice by modulating the SA-, JA-, and ET-signaling pathways [82]. However, which effectors are perceived by BPH9 cells remains unknown.
Early signaling events
Following ligand perception, plant immune receptors activate multiple kinases, mainly including RLCKs; activated RLCKs phosphorylate downstream factors, such as Ca2+ channel proteins, respiratory burst oxidase homologous (RBOH) proteins and MPK kinase kinases (MKKKs), thereby activating a series of follow-up responses, such as Ca2+ influx, an ROS bursts, and MPK cascades [2, 7, 29] (Fig. 1). These early signaling events play critical roles in plant defense responses to different stimuli, including herbivore infestation [87,88,89].
In rice, herbivore infestation also activates various early signaling events (Fig. 1). Rice planthopper infestation, for instance, induces bursts of cytosolic Ca2+ and ROS (predominantly H2O2) and activates MPK cascades [13, 17, 81]. SSB infestation also quickly and strongly activates MPKs [10, 90]. Moreover, RLCKs also play an important role in regulating these early signaling events in rice. After chitin or peptidoglycan (PGN) treatment, rice chitin and PGN receptors, chitin oligosaccharide elicitor-binding protein (OsCEBiP), and lysin motif (LysM)-containing proteins (OsLYP4 and OsLYP6) form a complex by interacting with the co-receptor chitin elicitor receptor kinase 1 (OsCERK1). Then, the complex triggers the activation of OsRLCK185 and OsRLCK176 [91]. Subsequently, activated OsRLCK185 not only directly phosphorylates the cyclic nucleotide gated channel (OsCNGC9), facilitating channel opening and inducing Ca2+ influx but also activates the OsMKKK18/24-OsMKK4-OsMPK3/6 cascade [91, 92]. Moreover, OsRLCK185 regulates the activation of RBOHs, leading to the ROS bursts [91, 92]. Like OsRLCK185, OsRLCK176 also positively regulates chitin/PGN-induced ROS bursts, Ca2+ influxes, and OsMPK3/6 activities [93]. Another RLCK, BSR1, positively regulates M. loreyi- or OsPep3-elicited rice defenses, including the expression of defense genes, and the production of H2O2 and diterpenoid phytoalexins, thus enhancing the ability of rice to resist feeding on M. loreyi larvae [94].
MPK cascades, one of the most highly conserved signaling modules downstream of PRR complexes, have also been reported to play an important role in regulating defense-related phytohormone signaling pathways [95, 96]. In rice, several MPK members have been revealed to participate in herbivore-induced defenses (Fig. 1). For instance, OsMPK3 and OsMPK4 contribute to rice defenses against SSB by positively regulating the JA-, SA-, and ET-signaling pathways and the production of TrypPIs [90, 97]. The OsMEK4-OsMPK3/6 module functions downstream of OsLRR-RLK1 and OsLRR-RLK2; silencing OsLRR-RLK1 or OsLRR-RLK2 significantly decreases the basal and herbivore-induced activation of OsMPK3/6 and induces JA and ET in rice, making rice susceptibility to SSB larvae [10, 98]. In addition to their role in regulating the ability of rice to resist SSB, some MPKs also play a role in rice defenses against rice planthoppers. The overexpression of OsMKK3 enhances BPH-induced JA, JA-Ile, and ABA levels and improves the resistance of rice to BPH [99]. The infection of gravid BPH females quickly induces significant levels of OsMAPK20-5 transcripts, but infestation by BPH nymphs has little effect; silencing OsMAPK20-5 enhances BPH oviposition but not feeding-induced nitric oxide (NO) and ET accumulation, which in turn reduces the hatching rate of BPH eggs and rice tolerance to infestation by gravid BPH females [100]. These results indicate that OsMAPK20-5 protects plants from autotoxicity by suppressing herbivore-induced defense signaling pathways, which are mainly mediated by NO and ET.
Phytohormone-mediated signaling pathways
Phytohormone-mediated signaling pathways function downstream of early signaling events and control plant defense outputs, including the reconfiguration of the transcriptome and metabolome, and plant resistance to insect herbivores. In rice, herbivore infestation can induce changes in the profile of phytohormones, such as JAs, SA, ET, and ABA, all of which jointly regulate the ability of rice to resist insect herbivores [10, 18, 24, 60, 101] (Fig. 1).
As reported in many plant species, the JA-signaling pathway also plays an important role in regulating the resistance of rice to insect herbivores. In general, the JA-signaling pathway positively regulates the resistance of rice to chewing insects, such as SSB and LF [12, 102,103,104,105,106,107,108], whereas its role in the resistance of rice to piercing-sucking insects, such as rice planthoppers, varies. For instance, overexpression of genes related to JA biosynthesis, such as an allene oxide cyclase gene (OsAOC) or a cis-12-oxo-phytodienoic acid (OPDA) reductase 3 (OsOPR3), in rice enhances the resistance of plants to SSB larvae [102], whereas silencing or knocking out genes related to JA biosynthesis, such as a lipoxygenase gene (OsHI-LOX) [103], phospholipase D genes (OsPLDα4 and OsPLDα5) [104], and allene oxide synthase genes (OsAOS1 and OsAOS2) [12], decreases resistance to SSB. The knockout of OsAOC in rice decreases the levels of herbivore-induced defensive compounds, including some phenolamines, flavonoids, and herbivore-induced plant volatiles (HIPVs); lower levels of these compounds increase the growth of rice LF larvae [105]. Additionally, the JA receptor coronatine insensitive 1a (OsCOI1a) [106] and the core transcription factor of the JA-signaling pathway (OsMYC2) [105] have been shown to positively regulate rice defenses against rice LF larvae. Both positive and negative regulation of the JA-signaling pathway in rice planthopper resistance have been reported. Knocking out OsAOC [24], JA receptors (OsCOI1a and OsCOI2) [106, 109] or the OsMYC2 transcription factor of the JA-signaling pathway [24] in rice, for instance, decreases the hatching rate of BPH eggs; moreover, silencing OsMYC3 (the homolog of OsMYC2) in rice facilitates the feeding of WBPH and SBPH nymphs [110]. However, silencing genes related to JA biosynthesis, such as OsAOS1, OsAOS2, or OsHI-LOX, in rice enhances the ability of rice to resist rice planthoppers [12, 103]. This discrepancy might be related to the genetic background of the rice varieties used and to the genes that were knocked out or silenced. Many genes have multiple functions; therefore, silencing such a gene may also influence plant insect resistance by affecting other pathways.
The ET-signaling pathway also contributes to the ability of rice to resist insect herbivores; however, its role changes with herbivore species [19, 111,112,113]. Silencing OsACS2, a gene encoding a key enzyme related to ET biosynthesis, ACC (1-amino-1-cyclopropanecarboxylic acid) synthase, decreases the production of SSB-elicited ET, TrypPIs, and HIPVs, and decreases the resistance of rice to SSB larvae [19]. However, silencing OsACS2 enhances the production of two BPH-induced rice volatiles, 2-heptanone and 2-heptanol; higher levels of these volatiles subsequently repel the feeding and oviposition of gravid BPH females but enhance the attractiveness of the rice plant to the egg parasitoid of BPH [19]. The negative effect of the ET pathway on the regulation of rice BPH resistance has also been reported in other studies. Ethylene-insensitive3-like1 (OsEIL1) [112] and OsEIL2 [111], two key ET-responsive transcription activators, have been reported to negatively affect the ability of rice to resist BPH; moreover, the E3 ligases, OsEBF1 (ethylene-insensitive3-binding F-box protein 1) and OsEBF2, positively modulate the ability of rice to resist BPH by directly interacting with OsEIL1 and degrading it through the ubiquitination pathway [112, 113]. Recently, the role of the ET pathway in regulating the light-induced ability of rice to resist BPH has been reported [111]: light facilitates the accumulation of the phytochrome protein (OsPhyB) in rice nuclei and accelerates the degradation of the phytochrome-interacting factor protein (OsPIL14); both processes decrease ET production and OsEIL2 protein levels, ultimately enhancing the resistance of rice to BPH.
The SA-signaling pathway can regulate the direct and indirect defenses of rice against planthoppers and LFs. For example, the exogenous application of methyl salicylate (MeSA) to rice plants not only restricts the growth, development, and reproduction of rice LFs [114], but also attracts the natural enemies of rice planthoppers [11, 115]. In BPH-resistant rice varieties, feeding by BPH nymphs significantly induces the expression of genes encoding rate-limiting enzymes involved in SA biosynthesis, like isochorismate synthase (OsICS1) and L-phenylalanine ammonia-lyase genes (OsPALs) [116]. The suppression of OsICS1 in rice enhances the feeding preference of BPH females for plants [117]; moreover, silencing OsPALs reduces BPH feeding-induced SA and lignin levels, compromising the ability of rice to tolerate BPH nymphs [116]. Rice plants carrying the BPH resistance gene Bph14 exhibit high SA levels, which leads to increased levels of BPH nymph-induced callose in plants, thereby enhancing rice resistance [84, 85].
In addition to signaling pathways mediated by JA, ET, and SA, pathways mediated by ABA, gibberellins (GAs) and cytokinins (CKs) also play roles in rice defense against insect herbivores [118,119,120,121,122,123] (Fig. 1). ABA levels are increased in rice plants by exogenously applying ABA, overexpressing the ABA biosynthesis-related gene OsNCED3 (9-cis-epoxycarotenoid dioxygenase), or silencing the ABA catabolic gene OsABA8ox3 (ABA 8’-hydroxylase), for instance, to effectively promote callose deposition in plant sieve plates and prevent BPHs from ingesting phloem sap [118, 119]. Similarly, strengthening the GA pathway in rice, by, for example, exogenously applying GA3 [120], expressing the GA receptor gene OsGID1 [121], or silencing the GA signaling suppressor DELLA gene OsSLR1 [122], enhances lignin and cellulose content, which in turn fortifies cell walls and ultimately enhances the ability of rice to resist gravid BPH females. BPH feeding also significantly induces the accumulation of cis-zeatin-type and N6-(Δ2-isopentenyl) adenine-type CKs in rice [123]. Treating rice with 6-benzylaminopurine (6-BA, a synthetic CK analog) or knocking out OsCKX1 (a CK oxidase/dehydrogenase gene that inactivates CKs) in rice facilitates the accumulation of CKs, JAs (JA-Ile and JA-Val), and lignin, leading to an increase in rice tolerance to BPH [123].
Accumulated evidence suggests that crosstalk plays an important role in regulating herbivore-induced rice defenses. For example, although some studies have found that JA signaling positively regulates the ability of rice to directly and indirectly resist planthoppers, as described above, other research has also revealed that silencing genes related to JA biosynthesis, such as OsAOS1, OsAOS2, or OsHI-LOX in rice, enhances the ability of rice to resist rice planthoppers by increasing the levels of SA and ROS [12, 103]. Silencing the SA-receptor gene OsNPR1 increases the resistance of rice to SSB by activating JA- and ET-mediated signaling pathways [124]. Additionally, the ET-responsive transcription activator OsEIL1 directly binds to the promoter of OsLOX9 (OsHI-LOX) and negatively regulates rice BPH resistance [112]. When JA biosynthesis is blocked in rice, 6-BA-induced rice tolerance to BPH is abolished, suggesting that the ability of CK-mediated rice to resist BPH depends on the JA pathway [123].
Transcription factors
Plant defense responses are accompanied by the activation and suppression of defense genes, with transcription factors (TFs) playing a crucial role in this process [4, 6, 7]. Hitherto, TFs associated with rice defense responses have been reported to be mainly in the WRKY, AP2/ERF (APETALA2/ethylene responsive factor), MYB, bZIP (basic leucine zipper factor), and bHLH (basic helix-loop-helix) subfamilies (Fig. 1).
WRKY TFs are essential components that function as both up- and downstream phytohormone signals in herbivore-induced rice defenses. Both OsWRKY53 and OsWRKY70, for example, interact directly with and are phosphorylated by OsMPK3/6 [14, 15], but their roles in mediating defensive responses in rice differ: OsWRKY70 positively regulates the SSB-induced accumulation of JA, ET, and TrypPIs, and the resistance of rice to SSB [15], whereas OsWRKY53 inhibits OsMPK3/6 activity and subsequent JA/ET-mediated rice defense responses, thereby maintaining an appropriate level of SSB-induced rice defenses [14]. Moreover, OsWRKY53 enhances the resistance of rice to BPH by increasing H2O2 production and reducing ET accumulation [14], but OsWRKY70 negatively modulates GA biosynthesis and the ability of rice to resist BPH [15]. OsWRKY46/72 directly binds to the promoter of the callose-biosynthesis gene LOC_Os01g67364.1, thereby enhancing callose deposition [84]. OsWRKY89 overexpression positively contributes to the resistance of rice to WBPH by regulating the lignin content in rice [125]. Silencing the SA-responsive gene OsWRKY45 confers on rice with the ability to resist BPH in both greenhouses and in the field by enhancing H2O2 levels in rice [126].
In addition to WRKYs, other TFs also play roles in regulating the ability of rice to resist insect herbivores. OsMYB22, for instance, is a transcriptional repressor that interacts with the TOPLESS transcriptional co-repressors and recruits a histone deacetylase (OsHDAC1); the result is a tripartite complex that suppresses the expression of the F3’H (flavanone 3’-hydroxylase) gene (a negative modulator of rice resistance to BPH), thereby enhancing the ability of rice to tolerate BPH [127]. OsMYB30 activates the transcription of OsPAL6/8 by directly binding to the promoters of these two genes, and contributes to the production of SA and lignin, as well as to the ability of rice to tolerate BPH nymphs [116]. OsERF3 (ethylene-responsive factor 3) and OsDREB1A (dehydration-responsive element-binding gene 1A) belong to the AP2/ERF subfamily [128, 129]. Silencing OsERF3 decreases the expression of OsMKK3, OsMPK3, and OsWRKY53/70 in rice, thereby inhibiting the JA-mediated defense responses of rice to SSB and enhancing the susceptibility of rice to SSB larvae [128]. The suppression of OsDREB1A enhances BPH-induced JA, JA-Ile, and ABA levels, and decreases ET production in rice, which subsequently decreases hatchability and delays the developmental duration of BPH eggs [129]. OsMYC2, a member of the bHLH- subfamily TF, activates the expression of OsNOMT (naringenin 7-O-methyltransferase, which catalyzes naringenin to yield sakuranetin) and OsLIS (linalool synthase), as well as the production of sakuranetin and linalool [130]. Moreover, the transactivation activity of OsMYC2 could be enhanced by other TFs, such as OsMYC2-like protein 1 (OsMYL1, also termed OsMYC3), OsMYL2 (also termed OsMYC4) and OsRERJ1, by physical interactions [130, 131].
Defensive compounds
Herbivore-induced changes in phytohormone profiles usually lead to an increase in defensive compounds and a decrease in nutrients in plants, which in turn enhances the direct and indirect resistance of plants to insect herbivores [3, 7, 26]. Plant defensive compounds mainly include terpenoids, phenolics, alkaloids, phenolamines, defensive proteins, HIPVs, and organic acids and their salts [7, 132]. These are thought to defend against insect herbivores by limiting their nutrient access, poisoning them, impairing their ability to digest food, and attracting their natural enemies, among other strategies [3, 26, 133,134,135].
In rice, some defensive compounds against insect herbivores have been reported (Table 2). They can influence the behavior, feeding, growth, development, survival, or fecundity of insect herbivores. Some organic acids and their salts (aconitic acid, oxalic acid, calcium oxalate, and sodium oxalate, among others), for instance, have been found to restrict the feeding of rice planthoppers [136, 137]. Additionally, certain flavonoids (for example, tricin [138], sakuranetin [139], quercitrin [140], schaftoside [141], isoschaftoside, neoschaftoside [142], and epigallocatechin [21]), a phenolic acid (ferulic acid) [143], and some phenolamines (feruloyl putrescine, isopentylamine, p-coumaroyl putrescine, N-feruloyl tyramine, feruloyl agmatine, and N1,N10-diferuloyl spermidine [61, 144]) significantly influence the survival and development of rice planthoppers. The actions of different defensive compounds exhibit different mechanisms. Schaftoside, for example, robustly binds with cyclin-dependent kinase 1 of BPH (NlCDK1) and then inhibits the activation of NlCDK1 as a kinase [145]. Some phenolic acids, including ferulic acid, vanillic acid, and 4-hydroxybenzoic acid, inhibit the acetylcholinesterase activity in rice weevils, delaying their molting process [146]. Sakuranetin directly impedes the growth of beneficial endosymbionts in BPH and disrupts the nutritional balance essential for BPH development [139]. Lignin and polysaccharides, such as cellulose, hemicellulose, pectin, and callose, bolster the rigidity of plant cell walls, increasing the difficulty of herbivore feeding and digestion [83, 84, 119].
Plant defensive proteins, such as polyphenol oxidases (PPOs), peroxidases (PODs), and PALs, can modify plant cell walls and compounds, and confer the ability to resist insect herbivores on plants [135]. For example, PODs and PPOs can modify the structure of polyphenols and convert them into quinones, which bind to amino acids or proteins, reducing the availability of nutrients to insect herbivores [135]. Moreover, PALs are key enzymes related to the biosynthesis of salicylic acid and phenylpropanoids in rice plants [149]. PODs and PALs are required for the lignification of plant cell walls, thereby reducing the ability of insect herbivores to feed and digest food [116, 135]. Protease inhibitors, including TrypPIs, act to inhibit the activity of digestive enzymes in the midgut of multiple rice pests, thereby impeding insect development [7, 26].
HIPVs encompass terpenoids, green leaf volatiles (GLVs), aromatic compounds (such as MeSA, methyl benzoate (MeBA), and indole), and others [161, 162]. HIPVs influence the behavior and performance of insect herbivores and their natural enemies, including predators and parasitoids [161]. Notably, different volatile compounds exert varying effects on the same insect (herbivore, parasitoid, or predator), and the same compound may function differently for different insects. In rice, certain volatiles, such as 2-heptanone, 2-heptanol, linalool, (Z)-3-hexenal, and (Z)-3-hexen-1-ol, are repellent to BPH [19, 150, 153, 158], whereas (E)-2-hexenal and (Z)-3-hexen-1-ol attract females of the WBPH and LF [157, 163]. MeBA demonstrates strong direct toxicity and repellent effects on S. frugiperda [164]. Additionally, volatiles like linalool, (E)-β-caryophyllene, (E,E)-4,8-dimethyl-1,3,7-nonanetriene (DMNT), MeSA, (Z)-3-hexenal, (E)-2-hexenal, and (Z)-3-hexen-1-ol are attractive to Anagrus nilaparvatae, an egg parasitoid of rice planthoppers [115, 153,154,155]. Some volatiles, including (Z)-3-hexene-1-ol, linalool, MeSA, DMNT, and (E,E,E)-4,8,12-trimethyltrideca-1,3,7,11-tetraene (TMTT), serve as attractants for Apanteles chilonis, a larval parasitoid of SSB [152]. Moreover, volatiles emitted from rice plants attacked by LF, Mythimna separata, or S. frugiperda can attract corresponding parasitoids such as Trathala flavo-orbitalis, Itoplectis naranyae, Microplitis mediator, and Cotesia marginiventris [165,166,167]. Interestingly, HIPVs may also function as DAMPs, prompting non-attacked tissues on the same plant and/or neighboring plants to respond more rapidly and robustly to subsequent herbivore attacks. For example, pre-exposing rice plants to indole activates the expression of defense genes (OsMPK3, OsWRKY70, and JA biosynthesis genes) and enhances the accumulation of JA and JA-Ile, priming rice defenses against S. frugiperda larvae [168]. Similarly, pre-exposure to rice volatiles emitted from SSB-infested rice plants enhances the direct and indirect resistance of rice to SSB [154].
Regulation of rice defenses and susceptibility by insect herbivores
To cope with plant resistance or obtain nutrients from plants, adapted herbivores also secrete a repertoire of effectors or factors to suppress plant defenses or enhance plant susceptibility [4, 6, 7] (Fig. 1).
Suppressing defense responses
By now, many herbivore effectors that suppress plant defenses have been identified. Like elicitors, herbivore effectors may also be present in oral secretions, excreta (frass or honeydew), and oviposition-associated secretions [1, 2]. In rice, such effectors have also been identified in piercing-sucking insect herbivores, principally in the BPH and SBPH (Table 1). As stated above, the infestation of BPH, SBPH or the green rice leafhopper (Nephotettix cincticeps) causes a significant Ca2+ influx in rice plants and induces resistance [16, 53, 57]. In response, rice planthoppers and N. cincticeps secrete calcium-binding proteins, like NlSEF1 from BPH [16], LsECF1 from SBPH [53], and NcSP84 from N. cincticeps [57], into rice tissues during feeding, and they bind free cytoplasmic Ca2+, which impairs rice defenses. The DNase II secreted from SBPH salivary glands degrades the extracellular DNA that is released from damaged plant cells in response to herbivore infestation, which prevents the triggering of rice defenses, including H2O2 bursts [54]. Overexpressing an odorant-binding protein (NlugOBP11) or heat shock 70 kDa protein cognate 3 (NlHSC70-3), which is secreted from BPH salivary glands in N. benthamiana leaves, inhibits the production of SA and the bacterial elicitor flg22-induced ROS [51, 52]. Moreover, effectors secreted from BPH salivary glands, such as the endo-β-1,4-glucanase (NlEG1) [48] and salivary protein 7 (NlSP7) [50], degrade celluloses in rice cell walls (the former effector) and mediate the metabolism of rice tricin (the latter effector). The C-terminal subunit of SBPH Vg (LsVgC) targets a rice TF, OsWRKY71, to inhibit H2O2-mediated rice defense [55]. LsSP1, which can be secreted into rice plants during SBPH feeding, interacts with rice papain-like cysteine proteases to impair SA biosynthesis and subsequent SA-mediated rice defenses [56]. Thus far, no effectors derived from rice-chewing insects have been identified. Recently, Xue et al. [58] reported that mechanically wounding plants and applying oral secretions from antibiotic-treated SSB larvae to damaged areas induced higher levels of JA-mediated rice defenses compared to levels induced by mechanically wounding plants and treating them with oral secretions from non-antibiotic-treated SSB larvae; these results suggest that SSB larvae may exploit orally secreted microbes to avoid hyperactivating JA-mediated rice defenses, thereby facilitating the fitness of SSB larvae [58].
Effectors may also inhibit plant defenses by inducing the expression of genes that encode negative regulators of immune signaling [169, 170]. In rice, several genes, such as OsLRR-RLK2 [13], the simple extracellular LRR gene OsI-BAK1 [171], OsMAPK20-5 [100], the E3 ubiquitin ligase gene OsJMJ715 [18], OsNPR1 [124], the 13-lipoxygenase gene Osr9-LOX1 [172], and the histone H3K9 methyltransferase gene OsSDG703 [173], have been reported to negatively regulate defense-related signaling pathways and plant resistance to insect herbivores; moreover, the expression of these genes can be induced by herbivore infestation, suggesting that insect herbivores may suppress rice defenses by secreting effectors to regulate the expression of these genes. However, such of these effectors have not been identified in rice insect herbivores.
Inducing susceptible responses
To establish a population on a plant species, insect herbivores need to obtain nutrients from plants that satisfy their growth, development, and fecundity requirements. For this purpose, insect herbivores may also secrete effectors or factors to regulate the expression of the genes that allow the herbivore to reproduce, i.e., genes that induce plant-susceptible responses [169, 174]. In rice, herbivore infestation has been well documented to increase the levels of some compounds that are crucial for herbivore survival, growth, and development. For example, BPH infestation significantly increases the levels of some sugars (sucrose and glucose) and amino acids (alanine, Val, Ile, cysteine, proline, phenylalanine, glutamate, glutamine, histidine, and asparagine) in rice varieties that are susceptible to BPH but elicits few changes in resistant rice plants [22, 23]. Sucrose and some amino acids (aspartate, glutamine, and alanine) can serve as feeding stimulants of BPH [175, 176]. Lack of cysteine, histidine, and methionine in an artificial diet can reduce the survival rate of BPH nymphs and prolong their developmental duration [175]. Recent research has found that BPH or SSB infestation induces the biosynthesis of serotonin, an indole alkaloid in rice, and this compound contributes to the growth and development of BPH and SSB [177, 178]. These results demonstrate that rice insect herbivores may promote their fitness by inducing rice to become more susceptible to them. However, so far, no such effectors or factors have been reported.
Conclusions and perspectives
The extensive study of molecular interactions between plants and insect herbivores started at the beginning of this century. During the past 20 years or more, many mechanisms underlying plant–herbivore molecular interactions, such as those affecting both the production of herbivore-induced plant defenses and how insect herbivores regulate plant defense, have been found. These molecular mechanisms promote the establishment of a plant–insect interaction model. However, many aspects of the model still need to be clarified. First, although many herbivore elicitors and effectors have been identified, how plants detect these herbivore signals and then activate defenses in response, such as initiating early signaling events and activating phytohormone signaling, remains unclear. Moreover, how insect herbivores suppress plant defenses by secreting effectors, and what the targets of the effectors are are also unknown. Second, it has been observed that infestation by different insect herbivores induces different plant defenses [3, 37, 174]. However, which early, middle, and late components or pathways in plant defense responses shape the specificity of these responses is still unknown. Third, similar to pathogens, insect herbivores may also induce plant-susceptible responses, as stated above. However, how insect herbivores affect this process remains largely unknown. Fourth, only a limited number of defensive compounds in plants, including rice, have been confirmed to both directly and indirectly affect the growth, survival, development, and fecundity of insect herbivores. Hence, it is important to identify plant defensive compounds and elucidate their mechanisms of action. Moreover, exploring how insects perceive and respond to plant defensive compounds will also be essential. Fifth, in the field, plants generally face infestation by multiple insect herbivores, together with the natural enemies of insect herbivores and other organisms, simultaneously or successively. However, how plants and insect herbivores cope with this complex situation remains to be elucidated. Finally, abiotic factors (such as light, temperature, water, and soil fertility) can influence plant defense [179,180,181]. Moreover, insect herbivores may exploit the mechanisms by which plants resist these factors and suppress plant defenses for their own benefit [129]. How abiotic factors regulate the molecular interaction between plants and insect herbivores deserves future study. Expanding studies to these unexplored aspects would deepen the understanding of plant-insect interactions.
A comprehensive understanding of plant-insect molecular interactions in rice should inform the development of new control methods for insect pests, such as agents that induce plants to resist insects [9, 182], attractants or repellents for insect pests and their natural enemies [8, 9], and new varieties with broad-spectrum and durable resistance to pests or with resistance to both biotic and abiotic stresses [183, 184]. Moreover, the elucidation of plant-insect molecular interactions will lay a solid foundation for developing measures that can synergistically control multiple pests at the same time. These new measures, techniques, and/or strategies will promote the sustainable management of crop pests.
Availability of data and materials
This article comprehensively summarizes the latest literature in the field. All information and references cited in this review paper are duly acknowledged and properly cited within the text and in the reference list. Data sharing is not applicable to this article.
References
Stahl E, Hilfiker O, Reymond P. Plant-arthropod interactions: who is the winner? Plant J. 2018;93(4):703–28. https://doi.org/10.1111/tpj.13773.
Snoeck S, Guayazan-Palacios N, Steinbrenner AD. Molecular tug-of-war: plant immune recognition of herbivory. Plant Cell. 2022;34(5):1497–513. https://doi.org/10.1093/plcell/koac009.
Fernandez de Bobadilla M, Vitiello A, Erb M, Poelman EH. Plant defense strategies against attack by multiple herbivores. Trends Plant Sci. 2022;27(6):528–35. https://doi.org/10.1016/j.tplants.2021.12.010.
Schuman MC, Baldwin IT. The layers of plant responses to insect herbivores. Annu Rev Entomol. 2016;61:373–94. https://doi.org/10.1146/annurev-ento-010715-023851.
Wu J, Baldwin IT. New insights into plant responses to the attack from insect herbivores. Annu Rev Genet. 2010;44:1–24. https://doi.org/10.1146/annurev-genet-102209-163500.
Chen CY, Mao YB. Research advances in plant-insect molecular interaction. F1000Res. 2020;9(F1000 Faculty Rev):198. https://doi.org/10.12688/f1000research.21502.1.
Erb M, Reymond P. Molecular interactions between plants and insect herbivores. Annu Rev Plant Biol. 2019;70:527–57. https://doi.org/10.1146/annurev-arplant-050718-095910.
Lou YG, Zhang GR, Zhang WQ, Hu Y, Zhang J. Biological control of rice insect pests in China. Bio Control. 2013;67(1):8–20. https://doi.org/10.1016/j.biocontrol.2013.06.011.
Lou Y, Hu L, Li J. Herbivore-induced defenses in rice and their potential application in rice planthopper management. Dordrecht: Springer; 2015.
Hu L, Ye M, Kuai P, Ye M, Erb M, Lou Y. OsLRR-RLK1, an early responsive leucine-rich repeat receptor-like kinase, initiates rice defense responses against a chewing herbivore. New Phytol. 2018;219(3):1097–111. https://doi.org/10.1111/nph.15247.
Wang X, Du MH, Zhou GX, Cheng JA, Lou YG. Role of salicylic acid and H2O2 signaling pathways in the production of rice volatiles induced by the rice brown planthopper Nilparvata lugens. J Zhejiang Univ. 2007;33(1):15–23.
Zeng J, Zhang T, Huangfu J, Li R, Lou Y. Both allene oxide synthases genes are involved in the biosynthesis of herbivore-induced jasmonic acid and herbivore resistance in rice. Plants (Basel). 2021;10(3):442. https://doi.org/10.3390/plants10030442.
Ye M, Kuai P, Hu L, Ye M, Sun H, Erb M, et al. Suppression of a leucine-rich repeat receptor-like kinase enhances host plant resistance to a specialist herbivore. Plant Cell Environ. 2020;43(10):2571–85. https://doi.org/10.1111/pce.13834.
Hu L, Ye M, Li R, Zhang T, Zhou G, Wang Q, et al. The rice transcription factor WRKY53 suppresses herbivore-induced defenses by acting as a negative feedback modulator of mitogen-activated protein kinase activity. Plant Physiol. 2015;169(4):2907–21. https://doi.org/10.1104/pp.15.01090.
Li R, Zhang J, Li J, Zhou G, Wang Q, Bian W, et al. Prioritizing plant defence over growth through WRKY regulation facilitates infestation by non-target herbivores. Elife. 2015;4:e04805. https://doi.org/10.7554/eLife.04805.
Ye W, Yu H, Jian Y, Zeng J, Ji R, Chen H, et al. A salivary EF-hand calcium-binding protein of the brown planthopper Nilaparvata lugens functions as an effector for defense responses in rice. Sci Rep. 2017;7:40498. https://doi.org/10.1038/srep40498.
Zeng J, Ye W, Hu W, Jin X, Kuai P, Xiao W, et al. The N-terminal subunit of vitellogenin in planthopper eggs and saliva acts as a reliable elicitor that induces defenses in rice. New Phytol. 2023;238(3):1230–44. https://doi.org/10.1111/nph.18791.
Zhang Y, Chen M, Zhou S, Lou Y, Lu J. Silencing an E3 ubiquitin ligase gene OsJMJ715 enhances the resistance of rice to a piercing-sucking herbivore by activating ABA and JA signaling pathways. Int J Mol Sci. 2021;22(23):13020. https://doi.org/10.3390/ijms222313020.
Lu J, Li J, Ju H, Liu X, Erb M, Wang X, et al. Contrasting effects of ethylene biosynthesis on induced plant resistance against a chewing and a piercing-sucking herbivore in rice. Mol Plant. 2014;7(11):1670–82. https://doi.org/10.1093/mp/ssu085.
Yan L, Luo T, Huang D, Wei M, Ma Z, Liu C, et al. Recent advances in molecular mechanism and breeding utilization of brown planthopper resistance genes in rice: an integrated review. Int J Mol Sci. 2023;24(15):12061. https://doi.org/10.3390/ijms241512061.
Zhang Q, Li T, Gao M, Ye M, Lin M, Wu D, et al. Transcriptome and metabolome profiling reveal the resistance mechanisms of rice against brown planthopper. Int J Mol Sci. 2022;23(8):4083. https://doi.org/10.3390/ijms23084083.
Kang K, Yue L, Xia X, Liu K, Zhang W. Comparative metabolomics analysis of different resistant rice varieties in response to the brown planthopper Nilaparvata lugens Hemiptera: Delphacidae. Metabolomics. 2019;15(4):62. https://doi.org/10.1007/s11306-019-1523-4.
Peng L, Zhao Y, Wang H, Zhang J, Song C, Shangguan X, et al. Comparative metabolomics of the interaction between rice and the brown planthopper. Metabolomics. 2016;12(8). https://doi.org/10.1007/s11306-016-1077-7.
Xu J, Wang X, Zu H, Zeng X, Baldwin IT, Lou Y, et al. Molecular dissection of rice phytohormone signaling involved in resistance to a piercing-sucking herbivore. New Phytol. 2021;230(4):1639–52. https://doi.org/10.1111/nph.17251.
Li S, Qi L, Tan X, Li S, Fang J, Ji R. Small brown planthopper nymph infestation regulates plant defenses by affecting secondary metabolite biosynthesis in rice. Int J Mol Sci. 2023;24(5):4764. https://doi.org/10.3390/ijms24054764.
Erb M, Kliebenstein DJ. Plant secondary metabolites as defenses, regulators, and primary metabolites: the blurred functional trichotomy. Plant Physiol. 2020;184(1):39–52. https://doi.org/10.1104/pp.20.00433.
Reymond P. Receptor kinases in plant responses to herbivory. Curr Opin Biotechnol. 2021;70:143–50. https://doi.org/10.1016/j.copbio.2021.04.004.
Li Q, Wang C, Mou Z. Perception of damaged self in plants. Plant Physiol. 2020;182(4):1545–65. https://doi.org/10.1104/pp.19.01242.
Ngou BPM, Ding P, Jones JDG. Thirty years of resistance: zig-zag through the plant immune system. Plant Cell. 2022;34(5):1447–78. https://doi.org/10.1093/plcell/koac041.
Alborn HT, Turlings TCJ, Jones TH, Stenhagen G, Loughrin JH, Tumlinson JH. An elicitor of plant volatiles from beet armyworm oral secretion. Science. 1997;276(5314):945–9. https://doi.org/10.1126/science.276.5314.945.
Alborn HT, Hansen TV, Jones TH, Bennett DC, Tumlinson JH, Schmelz EA, et al. Disulfooxy fatty acids from the American bird grasshopper Schistocerca americana, elicitors of plant volatiles. P Natl Acad Sci USA. 2007;104(32):12976–81. https://doi.org/10.1073/pnas.0705947104.
Schmelz EA, LeClere S, Carroll MJ, Alborn HT, Teal PE. Cowpea chloroplastic ATP synthase is the source of multiple plant defense elicitors during insect herbivory. Plant Physiol. 2007;144(2):793–805. https://doi.org/10.1104/pp.107.097154.
Doss RP, Oliver JE, Proebsting WM, Potter SW, Kuy SR, Clement SL, et al. Bruchins: Insect-derived plant regulators that stimulate neoplasm formation. P Natl Acad Sci USA. 2000;97(11):6218–23. https://doi.org/10.1073/pnas.110054697.
Fatouros NE, Paniagua Voirol LR, Drizou F, Doan QT, Pineda A, Frago E, et al. Role of large cabbage white butterfly male-derived compounds in elicitation of direct and indirect egg-killing defenses in the black mustard. Front Plant Sci. 2015;6:794. https://doi.org/10.3389/fpls.2015.00794.
Stahl E, Brillatz T, Ferreira Queiroz E, Marcourt L, Schmiesing A, Hilfiker O, et al. Phosphatidylcholines from Pieris brassicae eggs activate an immune response in Arabidopsis. Elife. 2020;9:e60293. https://doi.org/10.7554/eLife.60293.
Hundacker J, Bittner N, Weise C, Brohan G, Varama M, Hilker M. Pine defense against eggs of an herbivorous sawfly is elicited by an annexin-like protein present in egg-associated secretion. Plant Cell Environ. 2022;45(4):1033–48. https://doi.org/10.1111/pce.14211.
Arimura GI. Making sense of the way plants sense herbivores. Trends Plant Sci. 2021;26(3):288–98. https://doi.org/10.1016/j.tplants.2020.11.001.
Gao H, Zou J, Lin X, Zhang H, Yu N, Liu Z. Nilaparvata lugens salivary protein NlG14 triggers defense response in plants. J Exp Bot. 2022;73(22):7477–87. https://doi.org/10.1093/jxb/erac354.
Shangguan X, Zhang J, Liu B, Zhao Y, Wang H, Wang Z, et al. A mucin-like protein of planthopper is required for feeding and induces immunity response in plants. Plant Physiol. 2018;176(1):552–65. https://doi.org/10.1104/pp.17.00755.
Gao H, Lin X, Yuan X, Zou J, Zhang H, Zhang Y, et al. The salivary chaperone protein NlDNAJB9 of Nilaparvata lugens activated plant immune responses. J Exp Bot. 2023. https://doi.org/10.1093/jxb/erad154.
Fu J, Shi Y, Wang L, Zhang H, Li J, Fang J, et al. Planthopper-secreted salivary disulfide isomerase activates immune responses in plants. Front Plant Sci. 2020;11:622513. https://doi.org/10.3389/fpls.2020.622513.
Yang JO, Nakayama N, Toda K, Tebayashi S, Kim CS. Structural determination of elicitors in Sogatella furcifera (Horváth) that induce Japonica rice plant varieties (Oryza sativa L.) to produce an ovicidal substance against S. furcifera eggs. Biosci Biotechnol Biochem. 2014;78(6):937–42. https://doi.org/10.1080/09168451.2014.917266.
Wari D, Kabir MA, Mujiono K, Hojo Y, Shinya T, Tani A, et al. Honeydew-associated microbes elicit defense responses against brown planthopper in rice. J Exp Bot. 2019;70(5):1683–96. https://doi.org/10.1093/jxb/erz041.
Shinya T, Hojo Y, Desaki Y, Christeller JT, Okada K, Shibuya N, et al. Modulation of plant defense responses to herbivores by simultaneous recognition of different herbivore-associated elicitors in rice. Sci Rep. 2016;6:32537. https://doi.org/10.1038/srep32537.
Shen W, Zhang X, Liu J, Tao K, Li C, Xiao S, et al. Plant elicitor peptide signalling confers rice resistance to piercing-sucking insect herbivores and pathogens. Plant Biotechnol J. 2022;20(5):991–1005. https://doi.org/10.1111/pbi.13781.
Dai YS, Liu D, Guo W, Liu ZX, Zhang X, Shi LL, et al. Poaceae-specific β-1,3;1,4-D-glucans link jasmonate signalling to OsLecRK1-mediated defence response during rice-brown planthopper interactions. Plant Biotechnol J. 2023;21(6):1286–300. https://doi.org/10.1111/pbi.14038.
Petrova A, Smith CM. Immunodetection of a brown planthopper (Nilaparvata lugens Stal) salivary catalase-like protein into tissues of rice, Oryza sativa. Insect Mol Biol. 2014;23(1):13–25. https://doi.org/10.1111/imb.12058.
Ji R, Ye W, Chen H, Zeng J, Li H, Yu H, et al. A salivary endo-β-1,4-glucanase acts as an effector that enables the brown planthopper to feed on rice. Plant Physiol. 2017;173(3):1920–32. https://doi.org/10.1104/pp.16.01493.
Rao W, Zheng X, Liu B, Guo Q, Guo J, Wu Y, et al. Secretome analysis and in planta expression of salivary proteins identify candidate effectors from the brown planthopper Nilaparvata lugens. Mol Plant Microbe Interact. 2019;32(2):227–39. https://doi.org/10.1094/MPMI-05-18-0122-R.
Gong G, Yuan LY, Li YF, Xiao HX, Li YF, Zhang Y, et al. Salivary protein 7 of the brown planthopper functions as an effector for mediating tricin metabolism in rice plants. Sci Rep. 2022;12(1):3205. https://doi.org/10.1038/s41598-022-07106-6.
Liu H, Wang C, Qiu CL, Shi JH, Sun Z, Hu XJ, et al. A salivary odorant-binding protein mediates Nilaparvata lugens feeding and host plant phytohormone suppression. Int J Mol Sci. 2021;22(9):4988. https://doi.org/10.3390/ijms22094988.
Yang H, Zhang X, Li H, Ye Y, Li Z, Han X, et al. Heat shock 70 kDa protein cognate 3 of brown planthopper is required for survival and suppresses immune response in plants. Insects. 2022;13(3):299. https://doi.org/10.3390/insects13030299.
Tian T, Ji R, Fu J, Li J, Wang L, Zhang H, et al. A salivary calcium-binding protein from Laodelphax striatellus acts as an effector that suppresses defense in rice. Pest Manag Sci. 2021;77(5):2272–81. https://doi.org/10.1002/ps.6252.
Huang HJ, Cui JR, Xia X, Chen J, Ye YX, Zhang CX, et al. Salivary DNase II from Laodelphax striatellus acts as an effector that suppresses plant defence. New Phytol. 2019;224(2):860–74. https://doi.org/10.1111/nph.15792.
Ji R, Fu J, Shi Y, Li J, Jing M, Wang L, et al. Vitellogenin from planthopper oral secretion acts as a novel effector to impair plant defenses. New Phytol. 2021;232(2):802–17. https://doi.org/10.1111/nph.17620.
Huang HJ, Wang YZ, Li LL, Lu HB, Lu JB, Wang X, et al. Planthopper salivary sheath protein LsSP1 contributes to manipulation of rice plant defenses. Nat Commun. 2023;14(1):737. https://doi.org/10.1038/s41467-023-36403-5.
Hattori M, Nakamura M, Komatsu S, Tsuchihara K, Tamura Y, Hasegawa T. Molecular cloning of a novel calcium-binding protein in the secreted saliva of the green rice leafhopper Nephotettix cincticeps. Insect Biochem Mol Biol. 2012;42(1):1–9. https://doi.org/10.1016/j.ibmb.2011.10.001.
Xue R, Li Q, Guo R, Yan H, Ju X, Liao L, et al. Rice defense responses orchestrated by oral bacteria of the rice striped stem borer, Chilo suppressalis. Rice (N Y). 2023;16(1):1. https://doi.org/10.1186/s12284-022-00617-w.
Sobhy IS, Miyake A, Shinya T, Galis I. Oral secretions affect HIPVs induced by generalist (Mythimna loreyi) and specialist (Parnara guttata) herbivores in rice. J Chem Ecol. 2017;43(9):929–43. https://doi.org/10.1007/s10886-017-0882-4.
Acevedo FE, Smith P, Peiffer M, Helms A, Tooker J, Felton GW. Phytohormones in fall armyworm saliva modulate defense responses in plants. J Chem Ecol. 2019;45(7):598–609. https://doi.org/10.1007/s10886-019-01079-z.
Alamgir KM, Hojo Y, Christeller JT, Fukumoto K, Isshiki R, Shinya T, et al. Systematic analysis of rice (Oryza sativa) metabolic responses to herbivory. Plant Cell Environ. 2016;39(2):453–66. https://doi.org/10.1111/pce.12640.
Asano T, Tamura Y, Yasui H, Satoh K, Hattori M, Yasui H, et al. The rice GRH2 and GRH4 activate various defense responses to the green rice leafhopper and confer strong insect resistance. Plant Biotechnol. 2015;32(3):215–24. https://doi.org/10.5511/plantbiotechnology.15.0615a.
Tanaka K, Heil M. Damage-associated molecular patterns (DAMPs) in plant innate immunity: applying the danger model and evolutionary perspectives. Annu Rev Phytopathol. 2021;59:53–75. https://doi.org/10.1146/annurev-phyto-082718-100146.
Li C, Liu G, Xu C, Lee GI, Bauer P, Ling HQ, et al. The tomato suppressor of prosystemin-mediated responses2 gene encodes a fatty acid desaturase required for the biosynthesis of jasmonic acid and the production of a systemic wound signal for defense gene expression. Plant Cell. 2003;15(7):1646–61. https://doi.org/10.1105/tpc.012237.
Huffaker A, Pearce G, Veyrat N, Erb M, Turlings TC, Sartor R, et al. Plant elicitor peptides are conserved signals regulating direct and indirect antiherbivore defense. Proc Natl Acad Sci USA. 2013;110(14):5707–12. https://doi.org/10.1073/pnas.1214668110.
Poretsky E, Dressano K, Weckwerth P, Ruiz M, Char SN, Shi D, et al. Differential activities of maize plant elicitor peptides as mediators of immune signaling and herbivore resistance. Plant J. 2020;104(6):1582–602. https://doi.org/10.1111/tpj.15022.
Shinya T, Yasuda S, Hyodo K, Tani R, Hojo Y, Fujiwara Y, et al. Integration of danger peptide signals with herbivore-associated molecular pattern signaling amplifies anti-herbivore defense responses in rice. Plant J. 2018;94(4):626–37. https://doi.org/10.1111/tpj.13883.
Couto D, Zipfel C. Regulation of pattern recognition receptor signalling in plants. Nat Rev Immunol. 2016;16(9):537–52. https://doi.org/10.1038/nri.2016.77.
Wang J, Song W, Chai J. Structure, biochemical function, and signaling mechanism of plant NLRs. Mol Plant. 2023;16(1):75–95. https://doi.org/10.1016/j.molp.2022.11.011.
Steinbrenner AD, Munoz-Amatriain M, Chaparro AF, Aguilar-Venegas JM, Lo S, Okuda S, et al. A receptor-like protein mediates plant immune responses to herbivore-associated molecular patterns. Proc Natl Acad Sci USA. 2020;117(49):31510–8. https://doi.org/10.1073/pnas.2018415117.
Poretsky E, Ruiz M, Ahmadian N, Steinbrenner AD, Dressano K, Schmelz EA, et al. Comparative analyses of responses to exogenous and endogenous antiherbivore elicitors enable a forward genetics approach to identify maize gene candidates mediating sensitivity to herbivore-associated molecular patterns. Plant J. 2021;108(5):1295–316. https://doi.org/10.1111/tpj.15510.
Wang L, Einig E, Almeida-Trapp M, Albert M, Fliegmann J, Mithofer A, et al. The systemin receptor SYR1 enhances resistance of tomato against herbivorous insects. Nat Plants. 2018;4(3):152–6. https://doi.org/10.1038/s41477-018-0106-0.
Yamaguchi Y, Pearce G, Ryan CA. The cell surface leucine-rich repeat receptor for AtPep1, an endoaenous peptide elicitor in Arabidopsis, is functional in transgenic tobacco cells. P Natl Acad Sci USA. 2006;103(26):10104–9. https://doi.org/10.1073/pnas.0603729103.
Yamaguchi Y, Huffaker A, Bryan AC, Tax FE, Ryan CA. PEPR2 is a second receptor for the Pep1 and Pep2 peptides and contributes to defense responses in Arabidopsis. Plant Cell. 2010;22(2):508–22. https://doi.org/10.1105/tpc.109.068874.
Ma C, Guo J, Kang Y, Doman K, Bryan AC, Tax FE, et al. AtPEPTIDE RECEPTOR2 mediates the AtPEPTIDE1-induced cytosolic Ca2+ rise, which is required for the suppression of Glutamine Dumper gene expression in Arabidopsis roots. J Integr Plant Biol. 2014;56(7):684–94. https://doi.org/10.1111/jipb.12171.
Liu Y, Wu H, Chen H, Liu Y, He J, Kang H, et al. A gene cluster encoding lectin receptor kinases confers broad-spectrum and durable insect resistance in rice. Nat Biotechnol. 2015;33(3):301–5. https://doi.org/10.1038/nbt.3069.
Lecouls AC, Salesses G, Minot JC, Voisin R, Bonnet A, Esmenjaud D. Spectrum of the Ma genes for resistance to Meloidogyne spp in Myrobalan plum. Theor Appl Genet. 1997;95(8):1325–34. https://doi.org/10.1007/s001220050700.
Zhu X, Xiao K, Cui H, Hu J. Overexpression of the Prunus sogdiana NBS-LRR subgroup gene PsoRPM2 promotes resistance to the root-knot nematode Meloidogyne incognita in Tobacco. Front Microbiol. 2017;8:2113. https://doi.org/10.3389/fmicb.2017.02113.
Warmerdam S, Sterken MG, Sukarta OCA, van Schaik CC, Oortwijn MEP, Lozano-Torres JL, et al. The TIR-NB-LRR pair DSC1 and WRKY19 contributes to basal immunity of Arabidopsis to the root-knot nematode Meloidogyne incognita. BMC Plant Biol. 2020;20(1):73. https://doi.org/10.1186/s12870-020-2285-x.
Messeguer R, Ganal MW, Devicente MC, Young ND, Bolkan H, Tanksly SD. High resolution RFLP map around the root knot nematode resistance gene (Mi) in tomato. Theor Appl Genet. 1991;82(5):529–36. https://doi.org/10.1007/BF00226787.
Du B, Zhang W, Liu B, Hu J, Wei Z, Shi Z, et al. Identification and characterization of Bph14, a gene conferring resistance to brown planthopper in rice. Proc Natl Acad Sci USA. 2009;106(52):22163–8. https://doi.org/10.1073/pnas.0912139106.
Zhao Y, Huang J, Wang Z, Jing S, Wang Y, Ouyang Y, et al. Allelic diversity in an NLR gene BPH9 enables rice to combat planthopper variation. Proc Natl Acad Sci USA. 2016;113(45):12850–5. https://doi.org/10.1073/pnas.1614862113.
Tamura Y, Hattori M, Yoshioka H, Yoshioka M, Takahashi A, Wu J, et al. Map-based cloning and characterization of a brown planthopper resistance gene Bph26 from Oryza sativa L. Indica cultivar ADR52. Sci Rep. 2014;4:5872. https://doi.org/10.1038/srep05872.
Hu L, Wu Y, Wu D, Rao W, Guo J, Ma Y, et al. The coiled-coil and nucleotide binding domains of BROWN PLANTHOPPER RESISTANCE14 function in signaling and resistance against planthopper in rice. Plant Cell. 2017;29(12):3157–85. https://doi.org/10.1105/tpc.17.00263.
Guo J, Wang H, Guan W, Guo Q, Wang J, Yang J, et al. A tripartite rheostat controls self-regulated host plant resistance to insects. Nature. 2023;618(7966):799–807. https://doi.org/10.1038/s41586-023-06197-z.
Ji H, Kim S, Kim Y, Suh J, Park H, Sreenivasulu N, Hechanova SL, Kim H, et al. Map-based cloning and characterization of the Bph18 gene from wild rice conferring resistance to brown planthopper insect pest. Sci Rep. 2016;6:34376. https://doi.org/10.1038/srep34376.
Jiang Y, Ding P. Calcium signaling in plant immunity: a spatiotemporally controlled symphony. Trends Plant Sci. 2022;28(1):74–89. https://doi.org/10.1016/j.tplants.2022.11.001.
Liang X, Zhou JM. Receptor-like cytoplasmic kinases: central players in plant receptor kinase-mediated signaling. Annu Rev Plant Biol. 2018;69:267–99. https://doi.org/10.1146/annurev-arplant-042817-040540.
He Y, Zhou J, Meng X. Phosphoregulation of Ca2+ influx in plant immunity. Trends Plant Sci. 2019;24(12):1067–9. https://doi.org/10.1016/j.tplants.2019.10.004.
Wang Q, Li J, Hu L, Zhang T, Zhang G, Lou Y. OsMPK3 positively regulates the JA signaling pathway and plant resistance to a chewing herbivore in rice. Plant Cell Rep. 2013;32(7):1075–84. https://doi.org/10.1007/s00299-013-1389-2.
Wang C, Wang G, Zhang C, Zhu P, Dai H, Yu N, et al. OsCERK1-mediated chitin perception and immune signaling requires receptor-like cytoplasmic kinase 185 to activate an MAPK cascade in rice. Mol Plant. 2017;10(4):619–33. https://doi.org/10.1016/j.molp.2017.01.006.
Wang J, Liu X, Zhang A, Ren Y, Wu F, Wang G, et al. A cyclic nucleotide-gated channel mediates cytoplasmic calcium elevation and disease resistance in rice. Cell Res. 2019;29(10):820–31. https://doi.org/10.1038/s41422-019-0219-7.
Ao Y, Li Z, Feng D, Xiong F, Liu J, Li JF, et al. OsCERK1 and OsRLCK176 play important roles in peptidoglycan and chitin signaling in rice innate immunity. Plant J. 2014;80(6):1072–84. https://doi.org/10.1111/tpj.12710.
Kanda Y, Shinya T, Maeda S, Mujiono K, Hojo Y, Tomita K, et al. BSR1, a rice receptor-like cytoplasmic kinase, positively regulates defense responses to herbivory. Int J Mol Sci. 2023;24(12):10395. https://doi.org/10.3390/ijms241210395.
Pitzschke A. Modes of MAPK substrate recognition and control. Trends Plant Sci. 2015;20(1):49–55. https://doi.org/10.1016/j.tplants.2014.09.006.
Zhang M, Su J, Zhang Y, Xu J, Zhang S. Conveying endogenous and exogenous signals: MAPK cascades in plant growth and defense. Curr Opin Plant Biol. 2018;45(Pt A):1–10. https://doi.org/10.1016/j.pbi.2018.04.012.
Liu X, Li J, Xu L, Wang Q, Lou Y. Expressing OsMPK4 impairs plant growth but enhances the resistance of rice to the striped stem borer Chilo suppressalis. Int J Mol Sci. 2018;19(4):1182. https://doi.org/10.3390/ijms19041182.
Ye M. Functional characterization of receptor-like kinase genes OsHI-RLK2 and OsHI-PSKR in herbivore-induced defenses in rice. Ph.D. Thesis. Hangzhou: Zhejiang University; 2016.
Zhou S, Chen M, Zhang Y, Gao Q, Noman A, Wang Q, et al. OsMKK3, a stress-responsive protein kinase, positively regulates rice resistance to Nilaparvata lugens via phytohormone dynamics. Int J Mol Sci. 2019;20(12):3023. https://doi.org/10.3390/ijms20123023.
Li J, Liu X, Wang Q, Huangfu J, Schuman MC, Lou Y. A group D MAPK protects plants from autotoxicity by suppressing herbivore-induced defense signaling. Plant Physiol. 2019;179(4):1386–401. https://doi.org/10.1104/pp.18.01411.
Lu J, Robert CA, Riemann M, Cosme M, Mene-Saffrane L, Massana J, et al. Induced jasmonate signaling leads to contrasting effects on root damage and herbivore performance. Plant Physiol. 2015;167(3):1100–16. https://doi.org/10.1104/pp.114.252700.
Guo HM, Li HC, Zhou SR, Xue HW, Miao XX. Cis-12-oxo-phytodienoic acid stimulates rice defense response to a piercing-sucking insect. Mol Plant. 2014;7(11):1683–92. https://doi.org/10.1093/mp/ssu098.
Zhou G, Qi J, Ren N, Cheng J, Erb M, Mao B, et al. Silencing OsHI-LOX makes rice more susceptible to chewing herbivores, but enhances resistance to a phloem feeder. Plant J. 2009;60(4):638–48. https://doi.org/10.1111/j.1365-313X.2009.03988.x.
Qi J, Zhou G, Yang L, Erb M, Lu Y, Sun X, et al. The chloroplast-localized phospholipases D α4 and α5 regulate herbivore-induced direct and indirect defenses in rice. Plant Physiol. 2011;157(4):1987–99. https://doi.org/10.1104/pp.111.183749.
Zhuang Y, Wang X, Llorca LC, Lu J, Lou Y, Li R. Role of jasmonate signaling in rice resistance to the leaf folder Cnaphalocrocis medinalis. Plant Mol Biol. 2022;109(4–5):627–37. https://doi.org/10.1007/s11103-021-01208-x.
Ye M, Luo SM, Xie JF, Li YF, Xu T, Liu Y, et al. Silencing COI1 in rice increases susceptibility to chewing insects and impairs inducible defense. PLoS One. 2012;7(4):e36214. https://doi.org/10.1371/journal.pone.0036214.
Zhou G, Wang X, Yan F, Wang X, Li R, Cheng J, et al. Genome-wide transcriptional changes and defence-related chemical profiling of rice in response to infestation by the rice striped stem borer Chilo suppressalis. Physiol Plant. 2011;143(1):21–40. https://doi.org/10.1111/j.1399-3054.2011.01483.x.
Senthil-Nathan S. Effect of methyl jasmonate (MeJA)-induced defenses in rice against the rice leaffolder Cnaphalocrocis medinalis (Guenée) (Lepidoptera: Pyralidae). Pest Manag Sci. 2019;75(2):460–5. https://doi.org/10.1002/ps.5139.
Wang X, Chen Y, Liu S, Fu W, Zhuang Y, Xu J, et al. Functional dissection of rice jasmonate receptors involved in development and defense. New Phytol. 2023;238(5):2144–58. https://doi.org/10.1111/nph.18860.
Li L, Zhang H, Chen C, Huang H, Tan X, Wei Z, et al. A class of independently evolved transcriptional repressors in plant RNA viruses facilitates viral infection and vector feeding. Proc Natl Acad Sci USA. 2021;118(11):e2016673118. https://doi.org/10.1073/pnas.2016673118.
Huang J, Qiu ZY, He J, Xu HS, Wang K, Du HY, et al. Phytochrome B mediates dim-light-reduced insect resistance by promoting the ethylene pathway in rice. Plant Physiol. 2023;191(2):1272–87. https://doi.org/10.1093/plphys/kiac518.
Ma F, Yang X, Shi Z, Miao X. Novel crosstalk between ethylene- and jasmonic acid-pathway responses to a piercing-sucking insect in rice. New Phytol. 2020;225(1):474–87. https://doi.org/10.1111/nph.16111.
Ma F, Li Z, Wang S, Li K, Tang F, Jia J, et al. The F-box protein OsEBF2 confers the resistance to the brown planthopper (Nilparvata lugens Stål). Plant Sci. 2023;327:111547. https://doi.org/10.1016/j.plantsci.2022.111547.
Kalaivani K, Kalaiselvi MM, Senthil-Nathan S. Effect of methyl salicylate (MeSA) induced changes in rice plant (Oryza sativa) that affect growth and development of the rice leaffolder, Cnaphalocrocis medinalis. Physiol Mol Plant Pathol. 2018;101:116–26. https://doi.org/10.1016/j.pmpp.2017.07.001.
Wang P, Lou YG. Screening and field evaluation of synthetic plant volatiles as attractants for Anagrus nilaparvatae Pang et Wang, an egg parasitoid of rice planthoppers. Chin J Appl Entomol. 2013;50(2):431–40. http://www.ent-bull.com.cn/qikanview.aspx.
He J, Liu Y, Yuan D, Duan M, Liu Y, Shen Z, et al. An R2R3 MYB transcription factor confers brown planthopper resistance by regulating the phenylalanine ammonia-lyase pathway in rice. Proc Natl Acad Sci USA. 2020;117(1):271–7. https://doi.org/10.1073/pnas.1902771116.
Wang B. Function analysis of herbivore resistance-related genes OsICS1 and OsHPL3 in rice. In: Thesis. vol. Ph.D. Hangzhou: Zhejiang University; 2012.
Zhou Y, Sun L, Wang S, Xie P, Liu J. A key ABA hydrolase gene, OsABA8ox3 is involved in rice resistance to Nilaparvata lugens by affecting callose deposition. J Asia-Pac Entomol. 2019;22(2):625–31. https://doi.org/10.1016/j.aspen.2019.04.008.
Liu J, Du H, Ding X, Zhou Y, Xie P, Wu J. Mechanisms of callose deposition in rice regulated by exogenous abscisic acid and its involvement in rice resistance to Nilaparvata lugens Stål (Hemiptera: Delphacidae). Pest Manag Sci. 2017;73(12):2559–68. https://doi.org/10.1002/ps.4655.
Wang W, Jin N, Mo X, Wu J, Lu J, Lou Y. Exogenous gibberellin GA3 enhances defense responses in rice to the brown planthopper Nilaparvata lugens (Stål). J Plant Biol. 2020;64(5):379–87. https://doi.org/10.1007/s12374-020-09271-5.
Chen L, Cao T, Zhang J, Lou Y. Overexpression of OsGID1 enhances the resistance of rice to the brown planthopper Nilaparvata lugens. Int J Mol Sci. 2018;19(9):2744. https://doi.org/10.3390/ijms19092744.
Zhang J, Luo T, Wang W, Cao T, Li R, Lou Y. Silencing OsSLR1 enhances the resistance of rice to the brown planthopper Nilaparvata lugens. Plant Cell Environ. 2017;40(10):2147–59. https://doi.org/10.1111/pce.13012.
Zhang X, Liu D, Gao D, Zhao W, Du H, Qiu Z, et al. Cytokinin confers brown planthopper resistance by elevating jasmonic acid pathway in rice. Int J Mol Sci. 2022;23(11):5946. https://doi.org/10.3390/ijms23115946.
Li R, Afsheen S, Xin Z, Han X, Lou Y. OsNPR1 negatively regulates herbivore-induced JA and ethylene signaling and plant resistance to a chewing herbivore in rice. Physiol Plant. 2013;147(3):340–51. https://doi.org/10.1111/j.1399-3054.2012.01666.x.
Wang H, Hao J, Chen X, Hao Z, Wang X, Lou Y, et al. Overexpression of rice WRKY89 enhances ultraviolet B tolerance and disease resistance in rice plants. Plant Mol Biol. 2007;65(6):799–815. https://doi.org/10.1007/s11103-007-9244-x.
Huangfu J, Li J, Li R, Ye M, Kuai P, Zhang T, et al. The transcription factor OsWRKY45 negatively modulates the resistance of rice to the brown planthopper Nilaparvata lugens. Int J Mol Sci. 2016;17(6):697. https://doi.org/10.3390/ijms17060697.
Sun B, Shen Y, Chen S, Shi Z, Li H, Miao X. A novel transcriptional repressor complex MYB22-TOPLESS-HDAC1 promotes rice resistance to brown planthopper by repressing F3’H expression. New Phytol. 2023;239(2):720–38. https://doi.org/10.1111/nph.18958.
Lu J, Ju H, Zhou G, Zhu C, Erb M, Wang X, et al. An EAR-motif-containing ERF transcription factor affects herbivore-induced signaling, defense and resistance in rice. Plant J. 2011;68(4):583–96. https://doi.org/10.1111/j.1365-313X.2011.04709.x.
Zhou S, Gao Q, Chen M, Zhang Y, Li J, Guo J, et al. Silencing a dehydration-responsive element-binding gene enhances the resistance of plants to a phloem-feeding herbivore. Plant Cell Environ. 2023;46(10):3090–101. https://doi.org/10.1111/pce.14569.
Valea I, Motegi A, Kawamura N, Kawamoto K, Miyao A, Ozawa R, et al. The rice wound-inducible transcription factor RERJ1 sharing same signal transduction pathway with OsMYC2 is necessary for defense response to herbivory and bacterial blight. Plant Mol Biol. 2022;109(4–5):651–66. https://doi.org/10.1007/s11103-021-01186-0.
Ogawa S, Miyamoto K, Nemoto K, Sawasaki T, Yamane H, Nojiri H, et al. OsMYC2, an essential factor for JA-inductive sakuranetin production in rice, interacts with MYC2-like proteins that enhance its transactivation ability. Sci Rep. 2017;7:40175. https://doi.org/10.1038/srep40175.
Deng Y, Lu S. Biosynthesis and regulation of phenylpropanoids in plants. Crit Rev Plant Sci. 2017;36(4):257–90. https://doi.org/10.1080/07352689.2017.1402852.
Negin B, Jander G. Convergent and divergent evolution of plant chemical defenses. Curr Opin Plant Biol. 2023;73:102368. https://doi.org/10.1016/j.pbi.2023.102368.
Li C, Zha W, Li W, Wang J, You A. Advances in the biosynthesis of terpenoids and their ecological functions in plant resistance. Int J Mol Sci. 2023;24(14):11561. https://doi.org/10.3390/ijms241411561.
Singh S, Kaur I, Kariyat R. The multifunctional roles of polyphenols in plant-herbivore interactions. Int J Mol Sci. 2021;22(3):1442. https://doi.org/10.3390/ijms22031442.
Yoshihara T, SÖGawa K, Pathak MD, Juliano BO, Sakamura S. Soluble silicic acid as a sucking inhibitory substance in rice against the brown plant hopper (Delphacidae, Homoptera). Entomol Exp Appl. 1979;26(3):314–22. https://doi.org/10.1111/j.1570-7458.1979.tb02932.x.
Yoshihara T, Sogawa K, Pathak MD, Juliano BO, Sakamura S. Oxalic acid as a sucking inhibitor of the brown planthopper in rice (Delphacidae, Homoptera). Entomol Exp Appl. 1980;27(2):149–55. https://doi.org/10.1111/j.1570-7458.1980.tb02959.x.
Zhang Z, Cui B, Zhang Y. Electrical penetration graphs indicate that tricin is a key secondary metabolite of rice, inhibiting phloem feeding of brown planthopper, Nilaparvata lugens. Entomol Exp Appl. 2015;156(1):14–27. https://doi.org/10.1111/eea.12307.
Liu M, Hong G, Li H, Bing X, Chen Y, Jing X, et al. Sakuranetin protects rice from brown planthopper attack by depleting its beneficial endosymbionts. Proc Natl Acad Sci USA. 2023;120(23):e2305007120. https://doi.org/10.1073/pnas.2305007120.
Jan R, Khan MA, Asaf S, Lee IJ, Kim KM. Overexpression of OsF3H modulates WBPH stress by alteration of phenylpropanoid pathway at a transcriptomic and metabolomic level in Oryza sativa. Sci Rep. 2020;10(1):14685. https://doi.org/10.1038/s41598-020-71661-z.
Stevenson PC, Kimmins FM, Grayer RJ, Raveendranath S. Schaftosides from rice phloem as feeding inhibitors and resistance factors to brown planthoppers, Nilaparvata lugens. Entomol Exp Appl. 1996;80(1):246–9. https://doi.org/10.1111/j.1570-7458.1996.tb00928.x.
Grayer RG, Harborne JB, Kimmins FM, Stevenson PC, Wijayagunasekera HNP. Phenolics in rice phloem sap as sucking deterrents to the brown planthopper, (Nilaparvata lugens). Acta Hortic. 1994;381:691–4. https://doi.org/10.17660/ActaHortic.1994.381.100.
Yang J, Sun XQ, Yan SY, Pan WJ, Zhang MX, Cai QN. Interaction of ferulic acid with glutathione s-transferase and carboxylesterase genes in the brown planthopper, Nilaparvata lugens. J Chem Ecol. 2017;43(7):693–702. https://doi.org/10.1007/s10886-017-0859-3.
Wang W, Yu Z, Meng J, Zhou P, Luo T, Zhang J, et al. Rice phenolamindes reduce the survival of female adults of the white-backed planthopper Sogatella furcifera. Sci Rep. 2020;10(1):5778. https://doi.org/10.1038/s41598-020-62752-y.
Hao PY, Feng YL, Zhou YS, Song XM, Li HL, Ma Y, et al. Schaftoside Interacts with NlCDK1 protein: a mechanism of rice resistance to brown planthopper, Nilaparvata lugens. Front Plant Sci. 2018;9:710. https://doi.org/10.3389/fpls.2018.00710.
Maazoun AM, Hlel TB, Hamdi SH, Belhadj F, Jemâa JMB, Marzouki MN. Screening for insecticidal potential and acetylcholinesterase activity inhibition of Urginea maritima bulbs extract for the control of Sitophilus oryzae (L.). J Asia-Pac Entomol. 2017;20(3):752–60. https://doi.org/10.1016/j.aspen.2017.04.004.
Yang Z, Li N, Kitano T, Li P, Spindel JE, Wang L, et al. Genetic mapping identifies a rice naringenin O-glucosyltransferase that influences insect resistance. Plant J. 2021;106(5):1401–13. https://doi.org/10.1111/tpj.15244.
Aboshi T, Iitsuka C, Galis I, Teraishi M, Kamo M, Nishimura A, et al. Isopentylamine is a novel defence compound induced by insect feeding in rice. Plant Cell Environ. 2021;44(1):247–56. https://doi.org/10.1111/pce.13902.
Gaquerel E, Gulati J, Baldwin IT. Revealing insect herbivory-induced phenolamide metabolism: from single genes to metabolic network plasticity analysis. Plant J. 2014;79(4):679–92. https://doi.org/10.1111/tpj.12503.
Liao Z, Wang L, Li C, Cao M, Wang J, Yao Z, et al. The lipoxygenase gene OsRCI-1 is involved in the biosynthesis of herbivore-induced JAs and regulates plant defense and growth in rice. Plant Cell Environ. 2022. https://doi.org/10.1111/pce.14341.
Xin Z, Wang Q, Yu Z, Hu L, Li J, Xiang C, et al. Overexpression of a xylanase inhibitor gene, OsHI-XIP, enhances resistance in rice to herbivores. Plant Mol Biol Rep. 2013;32(2):465–75. https://doi.org/10.1007/s11105-013-0661-5.
Hu X, Su S, Liu Q, Jiao Y, Peng Y, Li Y, et al. Caterpillar-induced rice volatiles provide enemy-free space for the offspring of the brown planthopper. Elife. 2020;9:e55421. https://doi.org/10.7554/eLife.55421.
Xiao Y, Wang Q, Erb M, Turlings TC, Ge L, Hu L, et al. Specific herbivore-induced volatiles defend plants and determine insect community composition in the field. Ecol Lett. 2012;15(10):1130–9. https://doi.org/10.1111/j.1461-0248.2012.01835.x.
Yao C, Du L, Liu Q, Hu X, Ye W, Turlings TCJ, et al. Stemborer-induced rice plant volatiles boost direct and indirect resistance in neighboring plants. New Phytol. 2023;237(6):2375–87. https://doi.org/10.1111/nph.18548.
Li CZ, Sun H, Gao Q, Bian FY, Noman A, Xiao WH, et al. Host plants alter their volatiles to help a solitary egg parasitoid distinguish habitats with parasitized hosts from those without. Plant Cell Environ. 2020;43(7):1740–50. https://doi.org/10.1111/pce.13747.
Ulhoa LA, Barrigossi JAF, Borges M, Laumann RA, Blassioli-Moraes MC. Differential induction of volatiles in rice plants by two stink bug species influence behaviour of conspecifics and their natural enemy Telenomus podisi. Entomol Exp Appl. 2020;168(1):76–90. https://doi.org/10.1111/eea.12869.
Wang B, Zhou G, Xin Z, Ji R, Lou Y. (Z)-3-Hexenal, one of the green leaf volatiles, increases susceptibility of rice to the white-backed planthopper Sogatella furcifera. Plant Mol Biol Rep. 2014;33(3):377–87. https://doi.org/10.1007/s11105-014-0756-7.
Tong X, Qi J, Zhu X, Mao B, Zeng L, Wang B, et al. The rice hydroperoxide lyase OsHPL3 functions in defense responses by modulating the oxylipin pathway. Plant J. 2012;71(5):763–75. https://doi.org/10.1111/j.1365-313X.2012.05027.x.
Sun X, Liu Z, Zhang AJ, Dong HB, Zeng FF, Pan XY, et al. Electrophysiological responses of the rice leaffolder, Cnaphalocrocis medinalis, to rice plant volatiles. J Insect Sci. 2014;14(1):70. https://doi.org/10.1093/jis/14.1.70.
Fujii T, Hori M, Matsuda K. Attractants for rice leaf bug, Trigonotylus caelestialium (Kirkaldy), are emitted from flowering rice panicles. J Chem Ecol. 2010;36(9):999–1005. https://doi.org/10.1007/s10886-010-9839-6.
Turlings TCJ, Erb M. Tritrophic interactions mediated by herbivore-induced plant volatiles: mechanisms, ecological relevance, and application potential. Annu Rev Entomol. 2018;63:433–52. https://doi.org/10.1146/annurev-ento-020117-043507.
Hu L. Integration of multiple volatile cues into plant defense responses. New Phytol. 2022;233(2):618–23. https://doi.org/10.1111/nph.17724.
Sun X, Liu Z, Zhang A, Dong HB, Zeng FF, Pan XY, et al. Electrophysiological responses of the rice leaffolder, Cnaphalocrocis medinalis, to rice plant volatiles. J Insect Sci. 2014;14:70. http://www.insectscience.org/14.70.
Zhao R, Wang H-H, Gao J, Zhang Y-J, Li X, Zhou J-J, et al. Plant volatile compound methyl benzoate is highly effective against Spodoptera frugiperda and safe to non-target organisms as an eco-friendly botanical-insecticide. Ecotox Environ Safe. 2022;245:114101. https://doi.org/10.1016/j.ecoenv.2022.114101.
Yuan JS, Kollner TG, Wiggins G, Grant J, Degenhardt J, Chen F. Molecular and genomic basis of volatile-mediated indirect defense against insects in rice. Plant J. 2008;55(3):491–503. https://doi.org/10.1111/j.1365-313X.2008.03524.x.
Liu J, Zhu J, Zhang P, Han L, Reynolds OL, Zeng R, et al. Silicon supplementation alters the composition of herbivore induced plant volatiles and enhances attraction of parasitoids to infested rice plants. Front Plant Sci. 2017;8:1265. https://doi.org/10.3389/fpls.2017.01265.
Shi JH, Sun Z, Hu XJ, Jin H, Foba CN, Liu H, et al. Rice defense responses are induced upon leaf rolling by an insect herbivore. BMC Plant Biol. 2019;19(1):514. https://doi.org/10.1186/s12870-019-2116-0.
Ye M, Glauser G, Lou Y, Erb M, Hu L. Molecular dissection of early defense signaling underlying volatile-mediated defense regulation and herbivore resistance in rice. Plant Cell. 2019;31(3):687–98. https://doi.org/10.1105/tpc.18.00569.
Gorshkov V, Tsers I. Plant susceptible responses: the underestimated side of plant-pathogen interactions. Biol Rev Camb Philos Soc. 2022;97(1):45–66. https://doi.org/10.1111/brv.12789.
Koseoglou E, van der Wolf JM, Visser RGF, Bai Y. Susceptibility reversed: modified plant susceptibility genes for resistance to bacteria. Trends Plant Sci. 2022;27(1):69–79. https://doi.org/10.1016/j.tplants.2021.07.018.
Ye M, Kuai P, Chen S, Lin N, Ye M, Hu L, et al. Silencing a simple extracellular leucine-rich repeat gene OsI-BAK1 enhances the resistance of rice to brown planthopper Nilaparvata lugens. Int J Mol Sci. 2021;22(22):12182. https://doi.org/10.3390/ijms222212182.
Zhou G, Ren N, Qi J, Lu J, Xiang C, Ju H, et al. The 9-lipoxygenase Osr9-LOX1 interacts with the 13-lipoxygenase-mediated pathway to regulate resistance to chewing and piercing-sucking herbivores in rice. Physiol Plant. 2014;152(1):59–69. https://doi.org/10.1111/ppl.12148.
Wen P, He J, Zhang Q, Qi H, Zhang A, Liu D, et al. SET domain group 703 regulates planthopper resistance by suppressing the expression of defense-related genes. Int J Mol Sci. 2023;24(16):13003. https://doi.org/10.3390/ijms241613003.
Stam JM, Kroes A, Li Y, Gols R, van Loon JJ, Poelman EH, et al. Plant interactions with multiple insect herbivores: from community to genes. Annu Rev Plant Biol. 2014;65:689–713. https://doi.org/10.1146/annurev-arplant-050213-035937.
Koyama K. Nutritional physiology of the brown rice planthopper, Nilaparvata lugens STÅL (Hemiptera: Delphacidae).: II. Essential amino acids for nymphal development. Appl Entomol Zool. 1985;20(4):424–30. https://doi.org/10.1303/aez.20.424.
Koyama K. Nutritional physiology of the brown rice planthopper, Nilaparvata lugens STÅL (Hemiptera: Delphacidae): I. Effect of sugars on nymphal development. Appl Entomol Zool. 1985;20(3):292–8. https://doi.org/10.1303/aez.20.292.
Lu HP, Luo T, Fu HW, Wang L, Tan YY, Huang JZ, et al. Resistance of rice to insect pests mediated by suppression of serotonin biosynthesis. Nat Plants. 2018;4(6):338–44. https://doi.org/10.1038/s41477-018-0152-7.
Wang L, Lu H, Zhang X, He Y, Zhang J, Guo X, et al. Disruption of serotonin biosynthesis increases resistance to striped stem borer without changing innate defense response in rice. J Pineal Res. 2023;75(2):e12895. https://doi.org/10.1111/jpi.12895.
Saijo Y, Loo EP. Plant immunity in signal integration between biotic and abiotic stress responses. New Phytol. 2020;225(1):87–104. https://doi.org/10.1111/nph.15989.
Aerts N, Pereira Mendes M, Van Wees SCM. Multiple levels of crosstalk in hormone networks regulating plant defense. Plant J. 2021;105(2):489–504. https://doi.org/10.1111/tpj.15124.
Nguyen D, Rieu I, Mariani C, van Dam NM. How plants handle multiple stresses: hormonal interactions underlying responses to abiotic stress and insect herbivory. Plant Mol Biol. 2016;91(6):727–40. https://doi.org/10.1007/s11103-016-0481-8.
Sobhy IS, Lou YG, Bruce TJA. Inducing plant resistance against insects using exogenous bioactive chemicals: key advances and future perspectives. Front Plant Sci. 2022;13:890884. https://doi.org/10.3389/fpls.2022.890884.
Mishra A, Barik SR, Pandit E, Yadav SS, Das SR, Pradhan SK. Genetics, mechanisms and deployment of brown planthopper resistance genes in rice. Crit Rev Plant Sci. 2022;41(2):91–127. https://doi.org/10.1080/07352689.2022.2062906.
Xiang X, Liu SH, Li HJ, Ofori AD, Yi XQ, Zheng AP. Defense strategies of rice in response to the attack of the herbivorous insect, Chilo suppressalis. Int J Mol Sci. 2023;24(18):14361. https://doi.org/10.3390/ijms241814361.
Acknowledgements
We thank Emily Wheeler for editorial assistance.
Funding
This study was funded by the National Natural Science Foundation of China (grant number 31930091 to Y.L. and 32202396 to P.K.), the Earmarked Fund for China Agriculture Research System (grant number CARS-01-41 to Y.L.) and the Zhejiang Provincial Natural Science Foundation (grant number LY24C140003 to P.K.).
Author information
Authors and Affiliations
Contributions
P.K. and Y.L. had the idea for the article. P.K. performed the literature searches, synthesized the data and drafted the sections. Y.L. assisted with the literature evaluation, manuscript revision and final editing. All authors have read and agreed to the published version of the manuscript.
Corresponding author
Ethics declarations
Ethics approval and consent to participate
Not applicable.
Consent for publication
Not applicable.
Competing interests
The authors declare no competing interests.
Additional information
Publisher’s Note
Springer Nature remains neutral with regard to jurisdictional claims in published maps and institutional affiliations.
Rights and permissions
Open Access This article is licensed under a Creative Commons Attribution 4.0 International License, which permits use, sharing, adaptation, distribution and reproduction in any medium or format, as long as you give appropriate credit to the original author(s) and the source, provide a link to the Creative Commons licence, and indicate if changes were made. The images or other third party material in this article are included in the article's Creative Commons licence, unless indicated otherwise in a credit line to the material. If material is not included in the article's Creative Commons licence and your intended use is not permitted by statutory regulation or exceeds the permitted use, you will need to obtain permission directly from the copyright holder. To view a copy of this licence, visit http://creativecommons.org/licenses/by/4.0/.
About this article
Cite this article
Kuai, P., Lou, Y. Advances in molecular interactions between rice and insect herbivores. Crop Health 2, 6 (2024). https://doi.org/10.1007/s44297-024-00027-y
Received:
Revised:
Accepted:
Published:
DOI: https://doi.org/10.1007/s44297-024-00027-y