Abstract
For modeling the electromechanical behavior of nano-bridge structures with slender narrow-width beam elements, not only the simultaneous effects of surface layer and size dependency should be taken into account but also corrected force models should be considered. In this paper, the instability of a narrow-width nano-bridge is studied based on strain gradient theory and Gurtin–Murdoch surface elasticity. The mid-plane stretching is incorporated in the governing equation as well as corrected force distribution. Using Rayleigh–Ritz method, a parametric analysis is conducted to examine the impacts of surface layer, size dependence, dispersion forces and structural damping on static and dynamic instability voltage of the nano-bridge.





Similar content being viewed by others
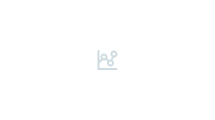
References
Rieth M, Schommers W (2006) Handbook of theoretical and computational nanotechnology.American Scientific Publishers, Los Angeles
Sedighi HM, Shirazi KH (2013) Vibrations of micro-beams actuated by an electric field via parameter expansion method. Acta Astronaut 85:19–24
Duan JS, Rach R (2013) A pull-in parameter analysis for the cantilever NEMS actuator model including surface energy, fringing field and Casimir effects. Int J Solids Struct 50:3511–3518
Muñoz-Gamarra JL, Alcaine P, Marigó E, Giner J, Uranga A, Esteve J, Barniol N (2013) Integration of NEMS resonators in a 65 nm CMOS technology. Microelectron Eng 110:246–249
Dragoman M, Dragoman D, Coccetti F, Plana R, Muller AA (2009) Microwave switches based on graphene. J Appl Phys 105:054309
Uranga A, Verd J, Marigó E, Giner J, Muñóz-Gamarra JL, Barniol N (2013) Exploitation of non-linearities in CMOS-NEMS electrostatic resonators for mechanical memories. Sens Actuators A 197:88–95
Hierold C, Jungen A, Stampfer C, Helbling T (2007) Nano electromechanical sensors based on carbon nanotubes. Sens Actuators A 136:51–61
Osterberg GPM (2007) Application of the generalized differential quadrature method to the study of pull-in phenomena of MEMS switches. Microelectromechanical Syst J 16:1334–1340
Beni YT, Koochi A, Abadyan M (2011) Theoretical study of the effect of Casimir force, elastic boundary conditions and size dependency on the pull-in instability of beam-type NEMS. Physica E 43:979–988
Noghrehabadi A, Beni YT, Koochi A, Kazemi AS, Yekrangi A, Abadyan M, Abadi MN (2011) Closed-form approximations of the pull-in parameters and stress field of electrostatic cantilever nano-actuators considering van der Waals attraction. Procedia Eng 10:3750–3756
Mobki H, Rezazadeh G, Sadeghi M, Vakili-Tahami F, Seyyed-Fakhrabadi MM (2013) A comprehensive study of stability in an electro-statically actuated micro-beam. Int J Non-Linear Mech 48:78–85
Koochi A, Hosseini-Toudeshky H (2015) Coupled effect of surface energy and size effect on the static and dynamic pull-in instability of narrow nano-switches. Int J Appl Mech 7(4):1550064
Fleck NA, Muller GM, Ashby MF, Hutchinson JW (1994) Strain gradient plasticity: theory and experiment. Acta Metall Mater 42:475–487
Lam DCC, Yang F, Chong ACM, Wang J, Tong P (2003) Experiments and theory in strain gradient elasticity. J Mech Phys Solids 51:1477–1508
McFarland AW, Colton JS (2005) Role of material microstructure in plate stiffness with relevance to microcantilever sensors. J Micromech Microeng 15(5):1060–1070
Chong ACM, Lam DCC (1999) Strain gradient plasticity effect in indentation hardness of polymers. J Mater Res 14(10):4103–4110
Al-Rub RKA, Voyiadjis GZ (2004) Determination of the material intrinsic length scale of gradient plasticity theory. Int J Multiscale Comput Eng 2(3):377–400
Wang W, Huang Y, Hsia KJ, Hu KX, Chandra A (2003) A study of microbend test by strain gradient plasticity. Int J Plast 19:365–382
Eringen AC, Edelen DGB (1972) On nonlocal elasticity. Int J Eng Sci 10:233–248
Ejike UB (1969) The plane circular crack problem in the linearized couple-stress theory. Int J Eng Sci 7:947–961
Kong S (2013) Size effect on pull-in behavior of electrostatically actuated microbeams based on a modified couple stress theory. Appl Math Model 37:7481–7488
Yang FACM, Chong ACM, Lam DCC, Tong P (2002) Couple stress based strain gradient theory for elasticity. Int J Solids Struct 39:2731–2743
Wang B, Zhou S, Zhao J, Chen X (2012) Pull-in instability of circular plate mems: a new model based on strain gradient elasticity theory. Int J Appl Mech 4:1250003
Ansari R, Gholami R, Mohammadi V, Shojaei MF (2013) Size-dependent pull-in instability of hydrostatically and electrostatically actuated circular microplates. J Comput Nonlinear Dyn 8:021015
Mohammadi V, Ansari R, Shojaei MF, Gholami R, Sahmani S (2013) Size-dependent dynamic pull-in instability of hydrostatically and electrostatically actuated circular microplates. Nonlinear Dyn 73:1515–1526
Kong S, Zhou S, Nie Z, Wang K (2009) Static and dynamic analysis of micro beams based on strain gradient elasticity theory. Int J Eng Sci 47:487–498
Wang B, Zhao J, Zhou S (2010) A micro scale Timoshenko beam model based on strain gradient elasticity theory. Eur J Mech A/Solids 29:591–599
Gurtin ME, Murdoch AI (1975) A continuum theory of elastic material surfaces. Arch Ration Mech Anal 57:291–323
Gurtin ME, Murdoch AI (1978) Surface stress in solids. Int J Solids Struct 14:431–440
Wang GF, Feng XQ (2009) Surface effects on buckling of nanowires under uniaxial compression. Appl Phys Lett 94:141913
He J, Lilley CM (2008) Surface effect on the elastic behavior of static bending nanowires. Nano Lett 8:1798–1802
Yan Z, Jiang LY (2011) The vibrational and buckling behaviors of piezoelectric nanobeams with surface effects. Nanotechnology 22:245703
Liu C, Rajapakse RKND (2010) Continuum models incorporating surface energy for static and dynamic response of nanoscale beams. IEEE Trans Nanotechnol 9(4):422–431
Fu Y, Zhang J (2011) Size-dependent pull-in phenomena in electrically actuated nanobeams incorporating surface energies. Appl Math Model 35:941–951
Ma JB, Jiang L, Asokanthan SF (2010) Influence of surface effects on the pull-in instability of NEMS electrostatic switches. Nanotechnology 21:505708
Koochi A, Hosseini-Toudeshky H, Ovesy HR, Abadyan M (2013) Modeling the influence of surface effect on instability of nano-cantilever in presence of van der Waals force. Int J Struct Stab Dyn 13:1250072
Koochi A, Kazemi A, Khandani F, Abadyan M (2012) Influence of surface effects on size-dependent instability of nano-actuators in the presence of quantum vacuum fluctuations. Phys Scr 85:035804
Rokni H, Lu W (2013) A continuum model for the static pull-in behavior of graphene nanoribbon electrostatic actuators with interlayer shear and surface energy effects. J Appl Phys 113:153512
Ansari R, Sahmani S (2011) Surface stress effects on the free vibration behavior of nanoplates. Int J Eng Sci 49:1204–1215
Gao XL, Mahmoud FF (2014) A new Bernoulli-Euler beam model incorporating microstructure and surface energy effects. Z Angew Math Phys 65:393–404
Zhou S, Gao XL (2013) Solutions of half-space and half-plane contact problems based on surface elasticity. Z Angew Math Phys 64:145–166
Dym CL, Shames IH (1984) Solid mechanics: a variational approach. Railway Publishing House, Beijing
Batra RC, Porfiri M, Spinello D (2006) Electromechanical model of electrically actuated narrow microbeams. Microelectromechanical Systems, Journal of 15:1175–1189
Klimchitskaya GL, Mohideen U, Mostepanenko VM (2000) Casimir and van der Waals forces between two plates or a sphere (lens) above a plate made of real metals. Phys Rev A 61:062107
Boström M, Sernelius BE (2000) Fractional van der Waals interaction between thin metallic films. Phys Rev B 61:2204
Israelachvili JN, Tabor DRFS (1972) The measurement of van der Waals dispersion forces in the range 1.5 to 130 nm. In: Proceedings of the Royal Society of London A: Mathematical, Physical and Engineering Sciences, Vol. 331, No. 1584, p 19–38
Gies H, Klingmüller K (2006) Casimir edge effects. Phys Rev Lett 97:220405
Lennard-Jones JE (1930) Perturbation problems in quantum mechanics. In: Proceedings of the Royal Society of London. Series A, Containing Papers of a Mathematical and Physical Character 598-615
Wang KF, Wang BL (2014) Influence of surface energy on the non-linear pull-in instability of nano-switches. Int J Non-Linear Mech 59:69–75
Author information
Authors and Affiliations
Corresponding author
Additional information
Technical Editor: Marcelo A. Savi.
Rights and permissions
About this article
Cite this article
Keivani, M., Koochi, A., Kanani, A. et al. Modeling the coupled effects of surface layer and size effect on the static and dynamic instability of narrow nano-bridge structure. J Braz. Soc. Mech. Sci. Eng. 39, 1735–1744 (2017). https://doi.org/10.1007/s40430-016-0644-1
Received:
Accepted:
Published:
Issue Date:
DOI: https://doi.org/10.1007/s40430-016-0644-1