Abstract
The current study examines the potential of utilizing the cold energy stored in liquefied natural gas (LNG) for power generation. Approximately 830 kJ/kg of the energy needed to produce LNG must be stored as cold energy, and regasification terminals can utilize this cold energy for a variety of purposes, including power generation. While the efficiency and net power gain of power generation cycles utilizing cold LNG usage have both increased, there is still room for improvement. An analysis of four alternative systems, combining the Rankine cycles (RC) and the direct expansion cycle (DEC), was conducted to determine the net power production and efficiencies of the system. The findings demonstrate that when the DEC and RCs are combined, net power gain and power cycle efficiency increase substantially. The DEC and single-stage stage RC have efficiency values of 21.05% and 23.38%, respectively, whereas the efficiencies of the four alternative combinations are 38.78%, 34.93%, 47.52%, and 47.23%. Maximum efficiency and net power gain were achieved when preheated DEC and multistage RCs were coupled. R-1150 was found to produce the best results when 11 different working fluids were examined for the given conditions with single-stage Rankine cycles. This indicates that condensation temperature has a significant influence on the cycle’s effectiveness. Sensitivity analysis reveals that the working fluid pressure, LNG mass flow rate, and turbine output pressure are crucial variables to achieve the best outcomes. Finally, the economic study demonstrated the viability of the suggested power system.















Similar content being viewed by others
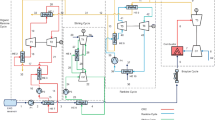
Data Availability
This is the research paper, and all data are properly cited. All data are available from the authors upon reasonable request.
Abbreviations
- W :
-
Power (kW)
- \(\eta \) :
-
Efficiency (%)
- Q :
-
Heat transfer rate (kW)
- i :
-
Number of stages
- net :
-
Net value
- th :
-
Thermal
- in :
-
Input
- out :
-
Output
- \({\text {NG}}\) :
-
Natural gas
- \({\text {LNG}}\) :
-
Liquefied natural gas
- \({\text {CULNG}}\) :
-
Cold utilization of LNG
- \({\text {DEC}}\) :
-
Direct expansion cycle
- \({\text {RC}}\) :
-
Rankine cycle
- \({\text {ORC}}\) :
-
Organic Rankine cycle
- \({\text {NPG}}\) :
-
Net power gain
- T :
-
Turbine
- E :
-
Heat exchanger
- P :
-
Pump
- \({\text {INR}}\) :
-
Indian Rupees
- \({\text {USD}}\) :
-
US Dollar
References
He, T.; Chong, Z.R.; Zheng, J.; Ju, Y.; Linga, P.: LNG cold energy utilization: prospects and challenges. Energy 170(C), 557–568 (2019). https://doi.org/10.1016/j.energy.2018.12
Arya, A.K.; Honwad, S.: Multiobjective optimization of a gas pipeline network: an ant colony approach. J. Pet. Explor. Prod. Technol. 8(4), 1389–1400 (2018). https://doi.org/10.1007/s13202-017-0410-7
Thomas, S.; Dawe, R.A.: Review of ways to transport natural gas energy from countries which do not need the gas for domestic use. Energy 28(14), 1461–1477 (2003). https://doi.org/10.1016/S0360-5442(03)001
Shingan, B.; Parthasarthy, V.: Recent progress in cold utilization of liquefied natural gas. Chem. Eng. Technol. (2023). https://doi.org/10.1002/ceat.202200484
Foss, M.M.: Introduction to LNG. Centre for energy economics, The University of Texas-Austin (2012)
Jensen, R.H.; Kurata, F.: Density of liquefied natural gas. J. Petrol. Technol. 21(06), 683–691 (1969)
Lentner, R.; Richter, M.; Kleinrahm, R.; Span, R.: Density measurements of liquefied natural gas (LNG) over the temperature range from (105 to 135) k at pressures up to 8.9 mpa. J. Chem. Thermodyn. 112, 68–76 (2017). https://doi.org/10.1016/j.jct.2017.04.002
Pospís̆il, J.; Charvát, P.; Arsenyeva, O.; Klimes̆, L.; S̆pilác̆ek, M.; Klemes̆, J.J.: Energy demand of liquefaction and regasification of natural gas and the potential of LNG for operative thermal energy storage. Renew. Sustain. Energy Rev. 99, 1–15 (2019). https://doi.org/10.1016/j.rser.2018.09.027
Vatani, A.; Mehrpooya, M.; Pakravesh, H.: Modification of an industrial ethane recovery plant using mixed integer optimization and shuffled frog leaping algorithm. Arab. J. Sci. Eng. 38(2), 439–455 (2013). https://doi.org/10.1007/s13369-012-0433-9
Pan, J.; Li, M.; Li, R.; Tang, L.; Bai, J.: Design and analysis of LNG cold energy cascade utilization system integrating light hydrocarbon separation, organic rankine cycle and direct cooling. Appl. Therm. Eng. 213, 118672 (2022). https://doi.org/10.1016/j.applthermaleng.2022.118672
Yao, S.-G.; Zhang, Z.; Wei, Y.; Liu, R.: Integrated design and optimization research of LNG cold energy and main engine exhaust heat utilization for LNG powered ships. Case Stud. Therm. Eng 33, 101976 (2022). https://doi.org/10.1016/j.csite.2022.101976
Liu, Y.; Guo, K.: A novel cryogenic power cycle for LNG cold energy recovery. Energy 36(5), 2828–2833 (2011). https://doi.org/10.1016/j.energy.2011.02.024
Romero Gómez, M.; Garcia, R.F.; Carbia Carril, J.; Romero Gómez, J.: High efficiency power plant with liquefied natural gas cold energy utilization. J. Energy Inst. 87(1), 59–68 (2014). https://doi.org/10.1016/j.joei.2014.02.007
Huang, Z.; Wan, Y.; Soh, K.; Islam, M.; Chua, K.: Off-design and flexibility analyses of combined cooling and power based liquified natural gas (LNG) cold energy utilization system under fluctuating regasification rates. Appl. Energy 310, 118529 (2022). https://doi.org/10.1016/j.apenergy.2022.118529
Griepentrog, H.; Sackarendt, P.: Vaporization of LNG with closed-cycle gas turbines. In: Turbo Expo: Power for Land, Sea, and Air. vol. 79740, pp. 01–01038. (1976).
Angelino, G.; Invernizzi, C.M.: The role of real gas brayton cycles for the use of liquid natural gas physical exergy. Appl. Therm. Eng. 31(5), 827–833 (2011)
Krey, G.: Utilization of the cold by LNG vaporization with the closed-cycle gas turbine. Atomkernenergie 32(4), 259–264 (1978). https://doi.org/10.1115/1.3230241
Kanbur, B.B.; Xiang, L.; Dubey, S.; Choo, F.H.; Duan, F.: Cold utilization systems of LNG: a review. Renew. Sustain. Energy Rev. 79, 1171–1188 (2017). https://doi.org/10.1016/j.rser.2017.05.161
Xue, F.; Chen, Y.; Ju, Y.: A review of cryogenic power generation cycles with liquefied natural gas cold energy utilization. Front. Energy 10(3), 363–374 (2016)
Choi, I.-H.; Lee, S.; Seo, Y.; Chang, D.: Analysis and optimization of cascade rankine cycle for liquefied natural gas cold energy recovery. Energy 61, 179–195 (2013). https://doi.org/10.1016/j.energy.2013.08.047
Zhang, L.; Tang, Q.: Comparisons of different power generation processes by LNG cold energy. 2012 AASRI Conf. Power Energy Syst. 2, 31 (2012). https://doi.org/10.1016/j.aasri.2012.09.010
Bao, J.; Yuan, T.; Zhang, L.; Zhang, N.; Zhang, X.; He, G.: Comparative study of liquefied natural gas (LNG) cold energy power generation systems in series and parallel. Energy Convers. Manag. 184, 107–126 (2019). https://doi.org/10.1016/j.enconman.2019.01.040
Shi, X.; Agnew, B.; Che, D.; Gao, J.: Performance enhancement of conventional combined cycle power plant by inlet air cooling, inter-cooling and LNG cold energy utilization. Appl. Therm. Eng. 30(14), 2003–2010 (2010). https://doi.org/10.1016/j.applthermaleng.2010.05.005
Wang, J.; Wang, J.; Dai, Y.; Zhao, P.: Thermodynamic analysis and optimization of a transcritical Co2 geothermal power generation system based on the cold energy utilization of lng. Appl. Therm. Eng. 70, 531–540 (2014). https://doi.org/10.1016/j.applthermaleng.2016.08.141
Stradioto, D.A.; Seelig, M.F.; Schneider, P.S.: Performance analysis of a CCGT power plant integrated to a LNG regasification process. J. Nat. Gas Sci. Eng. 23, 112–117 (2015). https://doi.org/10.1016/j.jngse.2015.01.032
Xue, F.; Chen, Y.; Ju, Y.: Design and optimization of a novel cryogenic rankine power generation system employing binary and ternary mixtures as working fluids based on the cold exergy utilization of liquefied natural gas (LNG). Energy 138, 706–720 (2017). https://doi.org/10.1016/j.energy.2017.07.122
Ma, G.; Lu, H.; Cui, G.; Huang, K.: Multi-stage rankine cycle (MSRC) model for LNG cold-energy power generation system. Energy 165, 673–688 (2018). https://doi.org/10.1016/j.energy.2018.09.203
Pattanayak, L.; Padhi, B.N.: Thermodynamic analysis of combined cycle power plant using regasification cold energy from LNG terminal. Energy 164, 1–9 (2018). https://doi.org/10.1016/j.energy.2018.08.187
Li, Y.; Zhang, G.; Liu, Y.; Song, X.; Yang, Y.: A cold and power cogeneration system utilizing LNG cryogenic energy and low-temperature waste heat. Energy Proced. 158, 2335–2340 (2019). https://doi.org/10.1016/j.egypro.2019.01.280
Qi, M.; Park, J.; Kim, J.; Lee, I.; Moon, I.: Advanced integration of LNG regasification power plant with liquid air energy storage: enhancements in flexibility, safety, and power generation. Appl. Energy 269, 115049 (2020). https://doi.org/10.1016/j.apenergy.2020.115049
Yu, H.; Gundersen, T.; Gençer, E.: Optimal liquified natural gas (LNG) cold energy utilization in an allam cycle power plant with carbon capture and storage. Energy Convers. Manage. 228, 113725 (2021). https://doi.org/10.1016/j.enconman.2020.113725
Li, Z.; Zhang, X.; He, X.; Wu, G.; Tian, S.; Zhang, D.; Zhang, Q.; Liu, Y.: Comparative analysis of thermal economy of two SOFC-GT-ST triple hybrid power systems with carbon capture and lng cold energy utilization. Energy Convers. Manag. 256, 115385 (2022). https://doi.org/10.1016/j.enconman.2022.115385
Liang, W.; Yu, Z.; Bai, S.; Li, G.; Wang, D.: Study on a near-zero emission SOFC-based multi-generation system combined with organic rankine cycle and transcritical co2 cycle for lng cold energy recovery. Energy Convers. Manag. 253, 115188 (2022). https://doi.org/10.1016/j.enconman.2021.115188
Tan, J.; Xie, S.; Wu, W.; Qin, P.; Ouyang, T.: Evaluating and optimizing the cold energy efficiency of power generation and wastewater treatment in LNG-fired power plant based on data-driven approach. J. Clean. Prod. 334, 130149 (2022)
Alsagri, A.S.; Chiasson, A.; Shahzad, M.W.: Geothermal energy technologies for cooling and refrigeration systems: an overview. Arab. J. Sci. Eng. 47, 5859 (2021). https://doi.org/10.1007/s13369-021-06296-x
Franco, A.; Casarosa, C.: Thermodynamic analysis of direct expansion configurations for electricity production by LNG cold energy recovery. Appl. Therm. Eng. 78, 649–657 (2015). https://doi.org/10.1016/j.applthermaleng.2014.11.062
Taqvi, S.A.; Tufa, L.D.; Muhadizir, S.: Optimization and dynamics of distillation column using aspen plus®. Proced. Eng. 148, 978–984 (2016). https://doi.org/10.1016/j.proeng.2016.06.484
Darvish, K.; Ehyaei, M.A.; Atabi, F.; Rosen, M.A.: Selection of optimum working fluid for organic rankine cycles by exergy and exergy-economic analyses. Sustainability 7(11), 15362–15383 (2015). https://doi.org/10.3390/su71115362
Guoguan, M.; Hongfang, L.; Guobiao, C.; Kun, H.: Multi-stage rankine cycle (MSRC) model for LNG cold-energy power generation system. Energy 165, 673–688 (2018)
Calm, J.M.; Hourahan, G.: Refrigerant data update. HPAC Eng. 79(1), 50–64 (2007)
Author information
Authors and Affiliations
Corresponding author
Ethics declarations
Conflict of interest
On behalf of all authors, the corresponding author states that there is no conflict of interest.
Rights and permissions
Springer Nature or its licensor (e.g. a society or other partner) holds exclusive rights to this article under a publishing agreement with the author(s) or other rightsholder(s); author self-archiving of the accepted manuscript version of this article is solely governed by the terms of such publishing agreement and applicable law.
About this article
Cite this article
Shingan, B., Vijay, P. & Pandian, K. Advanced Design of Power Generation Cycle with Cold Utilization from LNG. Arab J Sci Eng 48, 16973–16988 (2023). https://doi.org/10.1007/s13369-023-08301-x
Received:
Accepted:
Published:
Issue Date:
DOI: https://doi.org/10.1007/s13369-023-08301-x