Abstract
The present study investigates the aminothermal synthesis of SAPO-34 using various templates, including morpholine, diethylamine, triethylamine, triethanolamine, diglycolamine, and diisopropylamine, under static conditions. The catalyst's characteristics were assessed through XRD, SEM, EDS and FTIR analyses, and the catalytic behavior of the samples was studied in the MTO reaction. The results indicate that only morpholine leads to the formation of pure SAPO-34, and it is essential to regulate the crystallization time to prevent the formation of SAPO-20. Furthermore, the impact of the order of reactant addition and the intensity of stirring during aging on catalyst characteristics was investigated, and the outcomes were compared to those of the hydrothermally synthesized sample. The findings reveal that the aminothermal method reduces the crystallization time and enhances silica incorporation. However, this technique is recommended for the synthesis using polar templates or in the presence of polar additives. The utilization of non-polar templates such as DEA and TEA resulted in very thick pastes. The catalytic performance of the aminothermal samples was comparable to that of the hydrothermal samples, with a methanol conversion of over 98% and a selectivity to light olefins of approximately 80%. Nevertheless, the impact of preparation, aging, and post-treatment conditions on the hydrogen transfer index and alkanes production was significant.
Graphical abstract

Similar content being viewed by others
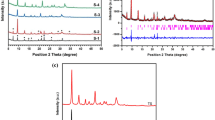
Data Availability
The authors confirm that the data supporting the findings of this study are available within the article and its supplementary materials.
References
Yu H, Wang C, Lin T et al (2022) Direct production of olefins from syngas with ultrahigh carbon efficiency. Nat Commun 13:5987. https://doi.org/10.1038/s41467-022-33715-w
Dimian AC, Bildea CS (2018) Energy efficient methanol-to-olefins process. Chem Eng Res Des 131:41–54. https://doi.org/10.1016/j.cherd.2017.11.009
Rimaz S, Kosari M, Zarinejad M, Ramakrishna S (2022) A comprehensive review on sustainability-motivated applications of SAPO-34 molecular sieve. J Mater Sci 57:848–886. https://doi.org/10.1007/s10853-021-06643-1
Siliveri S, Pinnepalli SSK, Joshi D et al (2021) Investigation on the Promoter-Induced Rapid Non-Aqueous Media Synthesis of SAPO-35 and Methanol-to-Olefin Reaction. ACS Omega 6:5661–5669. https://doi.org/10.1021/acsomega.0c06109
Venkatathri N (2005) Synthesis of high silica content silicoaluminophosphate-5 (SAPO-5) from non-aqueous medium using hexamethyleneimine template. 64:509–514
Han L, Yan X, Guo L et al (2021) Ionothermal synthesis of triclinic sapo-34 zeolites. Catalysts 11:616. https://doi.org/10.3390/catal11050616
Azim MM, Stark A (2018) The First Systematic Study on the Conditions Affecting the Ionothermal Synthesis of Silicoaluminophosphates. ChemistrySelect 3:12495–12503. https://doi.org/10.1002/slct.201802959
Chen SL, Li ZH, Li XF et al (2021) A green and cost-effective synthesis of hierarchical SAPO-34 through dry gel conversion and its performance in a methanol-to-olefin reaction. Ind Eng Chem Res 60:15380–15390. https://doi.org/10.1021/acs.iecr.1c02782
Nazari M, Moradi G, Behbahani RM, Ghavipour M (2016) Dry gel conversion as a suitable method for increasing the lifetime of SAPO-18 in MTO process. J Nat Gas Sci Eng 29:337–344. https://doi.org/10.1016/j.jngse.2016.01.022
Liu Z, Fan D, Tian P et al (2012) A novel solvothermal approach to synthesize SAPO molecular sieves using organic amines as the solvent and template. J Mater Chem 22:6568. https://doi.org/10.1039/c2jm15281a
Fan D, Tian P, Su X et al (2013) Aminothermal synthesis of CHA-type SAPO molecular sieves and their catalytic performance in methanol to olefins (MTO) reaction. J Mater Chem A 1:14206–14213. https://doi.org/10.1039/c3ta12829f
Wang D, Tian P, Yang M et al (2014) Synthesis of SAPO-34 with alkanolamines as novel templates and their application for CO2 separation. Microporous Mesoporous Mater 194:8–14. https://doi.org/10.1016/j.micromeso.2014.03.028
Wang D, Yang M, Zhang W et al (2016) Hollow nanocrystals of silicoaluminophosphate molecular sieves synthesized by an aminothermal co-templating strategy. CrystEngComm 18:1000–1008. https://doi.org/10.1039/C5CE01798J
Gao B, Fan D, Sun L et al (2017) Insights into the aminothermal crystallization process of SAPO-34 and its comparison with hydrothermal system. Microporous Mesoporous Mater 248:204–213. https://doi.org/10.1016/j.micromeso.2017.04.035
Sun C, Wang Y, Chen H, et al (2019) Seed-assisted synthesis of hierarchical SAPO-18 / 34 intergrowth and SAPO- 34 zeolites and their catalytic performance for the methanol-to-ole fi n reaction. Catal Today 0–1. https://doi.org/10.1016/j.cattod.2019.04.038
Sun L, Zhang W, Li Z et al (2021) Dual-template directed aminothermal syntheses and characterization of silicoaluminophosphates SAPO-CLO and ECR-40. Microporous Mesoporous Mater 315:110915. https://doi.org/10.1016/j.micromeso.2021.110915
Young D, Davis ME (1991) Studies on SAPO-5: synthesis with higher silicon contents. Zeolites 11:277–281. https://doi.org/10.1016/S0144-2449(05)80232-5
Nazari M, Yaripour F, Shifteh S (2021) Systematic evaluation and optimization of crystallization conditions for an ethanol-templated ZSM-5 zeolite using response surface methodology. Adv Powder Technol 32:4621–4634. https://doi.org/10.1016/j.apt.2021.10.018
Nazari M, Moradi G, Behbahani RM et al (2015) Preparation and Evaluation of the Modified Nanoparticle SAPO-18 for Catalytic Conversion of Methanol to Light Olefins. Catal Letters 145:1893–1903. https://doi.org/10.1007/s10562-015-1607-3
Grandjean D, Beale AM, Petukhov AV, Weckhuysen BM (2005) Unraveling the crystallization mechanism of CoAPO-5 molecular sieves under hydrothermal conditions. J Am Chem Soc 127:14454–14465. https://doi.org/10.1021/ja054014m
Nguyen B-TH (2013) Amine Volatility in CO2 Capture. The University of Texas at Austin
Sun Q, Xie Z, Yu J (2018) The state-of-the-art synthetic strategies for SAPO-34 zeolite catalysts in methanol-to-olefin conversion. Natl Sci Rev 5:542–558. https://doi.org/10.1093/nsr/nwx103
Graetsch H (2000) Two forms of aluminium phosphate tridymite from X-ray powder data. Acta Crystallogr Sect C Cryst Struct Commun 56:401–403. https://doi.org/10.1107/S0108270199015164
Nieminen V, Kumar N, Heikkilä T et al (2004) Isomerization of 1-butene over SAPO-11 catalysts synthesized by varying synthesis time and silica sources. Appl Catal A Gen 259:227–234. https://doi.org/10.1016/j.apcata.2003.09.038
Hajiashrafi T, Nemati Kharat A, Nemati A et al (2012) Study of preparation methods and their effect on the morphology and texture of SAPO-34 for the methanol to olefin reaction. React Kinet Mech Catal 108:417–432. https://doi.org/10.1007/s11144-012-0520-7
Alfonzo M, Goldwasser J, Lpez CM et al (1995) Effect of the synthesis conditions on the crystallinity and surface acidity of SAPO- 11. J Mol Catal A Chem 98:35–48
Lopez CM, Machado FJ, Rodriguez K, Ran-k-ez-agudelo MM (1997) The successive crystallization and characterization of SAPO-31 and SAPO-11 from the same synthesis gel: Influence on the selectivity for I-butene isomerization. 2449:. https://doi.org/10.1016/S0144-2449(97)00058-4
Rui P, Wang B, Chen F et al (2021) The hydrothermal synthesis of hierarchical SAPO-34 with improved MTO performance. New J Chem 45:11093–11100. https://doi.org/10.1039/d1nj01066b
Zhang L, Huang Y (2015) Crystallization and catalytic properties of molecular sieve SAPO-34 by a vapor-phase transport method. J Mater Chem A 3:4522–4529. https://doi.org/10.1039/c4ta06775d
Shalmani FM, Halladj R, Askari S (2012) Effect of contributing factors on microwave-assisted hydrothermal synthesis of nanosized SAPO-34 molecular sieves. Powder Technol 221:395–402. https://doi.org/10.1016/j.powtec.2012.01.036
Roldán R, Sánchez-Sánchez M, Sankar G et al (2007) Influence of pH and Si content on Si incorporation in SAPO-5 and their catalytic activity for isomerisation of n-heptane over Pt loaded catalysts. Microporous Mesoporous Mater 99:288–298. https://doi.org/10.1016/j.micromeso.2006.09.035
Morris RE, Weigel SJ (1997) The synthesis of molecular sieves from non-aqueous solvents. Chem Soc Rev 26:309. https://doi.org/10.1039/cs9972600309
Akhundzadeh Tezerjani A, Halladj R, Askari S (2021) Different view of solvent effect on the synthesis methods of zeolitic imidazolate framework-8 to tuning the crystal structure and properties. RSC Adv 11:19914–19923. https://doi.org/10.1039/d1ra02856a
Dumitriu E, Azzouz A, Hulea V et al (1997) Synthesis, characterization and catalytic activity of SAPO-34 obtained with piperidine as ternplating agent. Microporous Mater 10:1–12. https://doi.org/10.1016/S0927-6513(96)00107-1
Qiao Y, Yang M, Gao B et al (2016) Creation of hollow SAPO-34 single crystals: Via alkaline or acid etching. Chem Commun 52:5718–5721. https://doi.org/10.1039/c5cc10070d
Chen Z, Liu S, Wang H et al (2018) Synthesis and characterization of bundle-shaped ZSM-22 zeolite via the oriented fusion of nanorods and its enhanced isomerization performance. J Catal 361:177–185. https://doi.org/10.1016/j.jcat.2018.02.019
Abdollahi S, Ghavipour M, Nazari M et al (2015) Effects of static and stirring aging on physiochemical properties of SAPO-18 and its performance in MTO process. J Nat Gas Sci Eng 22:245–251. https://doi.org/10.1016/j.jngse.2014.11.036
Sastre G, Lewis DW, Catlow CR, a. (1996) Structure and Stability of Silica Species in SAPO Molecular Sieves. J Phys Chem 100:6722–6730. https://doi.org/10.1021/jp953362f
Yang J, Kikhtyanin OV, Wu W et al (2012) Influence of the template on the properties of SAPO-31 and performance of Pd-loaded catalysts for n-paraffin isomerization. Microporous Mesoporous Mater 150:14–24. https://doi.org/10.1016/j.micromeso.2011.09.020
Wang P, Lv A, Hu J et al (2012) The synthesis of SAPO-34 with mixed template and its catalytic performance for methanol to olefins reaction. Microporous Mesoporous Mater 152:178–184. https://doi.org/10.1016/j.micromeso.2011.11.037
Zhao D, Zhang Y, Li Z et al (2017) Synthesis of SAPO-18/34 intergrowth zeolites and their enhanced stability for dimethyl ether to olefins. RSC Adv 7:939–946. https://doi.org/10.1039/c6ra25080g
Xu L, Liu Z, Du A et al (2004) Synthesis, characterization, and MTO performance of MeAPSO-34 molecular sieves. Stud Surf Sci Catal 147:445–450
Zhang Y, Ding H, Li L et al (2022) Generating nanocrystalline SAPO-34 through bead-milling and porogen-assisted recrystallization: Structural evolution and catalytic consequence in dimethyl ether-to-olefin conversion. Appl Catal A Gen 632:118483. https://doi.org/10.1016/j.apcata.2022.118483
Hamidzadeh M, Nazari M, Rahimi Fard M (2021) MOR/DEA/TEA mixed-template synthesis of CHA-type SAPO with different silica and alumina sources. New J Chem 45:22113–22122. https://doi.org/10.1039/d1nj03836b
Marzpour Shalmani F, Halladj R, Askari S (2017) Physicochemical characterization to assess Ni and Zn incorporation into zeotype SAPO-34 nanoparticles synthesized with different mixing methods through ultrasound-promoted crystallization. RSC Adv 7:26756–26769. https://doi.org/10.1039/c7ra00272f
Ouyang J, Mu D, Zhang Y, Yang H (2018) Mineralogy and physico-chemical data of two newly discovered Halloysite in China and their contrasts with some typical minerals. Minerals 8:1–16. https://doi.org/10.3390/min8030108
Liping L, Xiaojing C, Junfen L, Jianguo W (2015) Synthesis of sapo-34/zsm-5 composite and its catalytic performance in the conversion of methanol to hydrocarbons. J Braz Chem Soc 26:290–296. https://doi.org/10.5935/0103-5053.20140279
Wang J, Yang M, Shang W et al (2017) Synthesis, characterization, and catalytic application of hierarchical SAPO-34 zeolite with three-dimensionally ordered mesoporous-imprinted structure. Microporous Mesoporous Mater 252:10–16. https://doi.org/10.1016/j.micromeso.2017.06.012
Izadbakhsh A, Farhadi F, Khorasheh F et al (2009) Key parameters in hydrothermal synthesis and characterization of low silicon content SAPO-34 molecular sieve. Microporous Mesoporous Mater 126:1–7. https://doi.org/10.1016/j.micromeso.2008.12.009
Liu Z, Li H, Zhang T et al (2020) Mother liquor induced preparation of SAPO-34 zeolite for MTO reaction. Catal Today 358:109–115. https://doi.org/10.1016/j.cattod.2020.03.034
Knyazeva EE, Kasnerik VI, Konnov SV et al (2018) Effect of Synthesis Temperature Formation of the Structure and Properties of Silicoaluminophosphate with the AEI Structure. Pet Chem 58:1245–1254. https://doi.org/10.1134/S0965544118140050
Auerbach S, Carrado K, Dutta P (2003) Hand book of Zeolite Science and Technology. CRC Press, New Tork
Wang D, Zhang L, Kamasamudram K, Epling WS (2013) In situ-DRIFTS study of selective catalytic reduction of NOx by NH3 over Cu-exchanged SAPO-34. ACS Catal 3:871–881. https://doi.org/10.1021/cs300843k
Teagarden DL, Kozlowski JF, White JL, Hem SL (1981) Aluminum Chlorohydrate I: Structure Studies. J Pharm Sci 70:758
Potter ME, Kezina J, Bounds R et al (2018) Investigating the role of framework topology and accessible active sites in silicoaluminophosphates for modulating acid-catalysis. Catal Sci Technol 8:5155–5164. https://doi.org/10.1039/c8cy01370e
Marchese L, Chen J, Wright PA, Thomas JM (1993) Formation of H3O+ at the Brönsted site in SAPO-34 catalysts. J Phys Chem 97:8109–8112. https://doi.org/10.1021/j100133a001
Ghavipour M, Goncalves TJ, Al Hussami R et al (2023) Uncovering the role of Lewis and Brønsted acid sites in perforated SAPO-34 with an enhanced lifetime in methanol conversion to light olefins. New J Chem 47:15907–15921. https://doi.org/10.1039/D3NJ02514D
Bahrami H, Darian JT, Sedighi M (2018) Simultaneous effects of water, TEAOH and morpholine on SAPO-34 synthesis and its performance in MTO process. Microporous Mesoporous Mater 261:111–118. https://doi.org/10.1016/j.micromeso.2017.11.011
Bordiga S, Regli L, Lamberti C et al (2005) FTIR adsorption studies of H2O and CH3OH in the isostructural H-SSZ-13 and H-SAPO-34: Formation of H-bonded adducts and protonated clusters. J Phys Chem B 109:7724–7732. https://doi.org/10.1021/jp044324b
Borodina E, Sharbini Harun Kamaluddin H, Meirer F et al (2017) Influence of the Reaction Temperature on the Nature of the Active and Deactivating Species during Methanol-to-Olefins Conversion over H-SAPO-34. ACS Catal 7:5268–5281. https://doi.org/10.1021/acscatal.7b01497
Deluca M, Janes C, Hibbitts D (2020) Contrasting Arene, Alkene, Diene, and Formaldehyde Hydrogenation in H-ZSM-5, H-SSZ-13, and H-SAPO-34 Frameworks during MTO. ACS Catal 10:4593–4607. https://doi.org/10.1021/acscatal.9b04529
Jie ZHU, Yu CUI, Zeeshan N et al (2010) In situ Synthesis of SAPO-34 Zeolites in Kaolin Microspheres for a Fluidized Methanol or Dimethyl Ether to Olefins Process. Chinese J Chem Eng 18:979–987. https://doi.org/10.1016/S1004-9541(09)60156-7
Kong C, Zhu J, Liu S, Wang Y (2017) SAPO-34 with a low acidity outer layer by epitaxial growth and its improved MTO performance. RSC Adv 7:39889–39898. https://doi.org/10.1039/c7ra06488h
Gao B, Yang M, Qiao Y et al (2016) A low-temperature approach to synthesize low-silica SAPO-34 nanocrystals and their application in the methanol-to-olefins (MTO) reaction. Catal Sci Technol 6:7569–7578. https://doi.org/10.1039/c6cy01461e
Zhong J, Han J, Wei Y et al (2017) Recent advances of the nano-hierarchical SAPO-34 in the methanol-to-olefin (MTO) reaction and other applications. Catal Sci Technol 7:4905–4923. https://doi.org/10.1039/c7cy01466j
Xing A, Yuan D, Tian D, Sun Q (2019) Controlling acidity and external surface morphology of SAPO-34 and its improved performance for methanol to olefins reaction. Microporous Mesoporous Mater 288:109562. https://doi.org/10.1016/j.micromeso.2019.109562
Omojola T, Logsdail AJ, Van Veen AC, Nastase SAF (2021) A quantitative multiscale perspective on primary olefin formation from methanol. Phys Chem Chem Phys 23:21437–21469. https://doi.org/10.1039/d1cp02551a
Wang B, Rui P, Cai X et al (2022) Insights into the methanol to olefins (MTO) performance of SAPO-34 under the stripper conditions of fluid catalytic cracking (FCC). Microporous Mesoporous Mater 345:1–8. https://doi.org/10.1016/j.micromeso.2022.112244
Müller S, Liu Y, Kirchberger FM et al (2016) Hydrogen Transfer Pathways during Zeolite Catalyzed Methanol Conversion to Hydrocarbons. J Am Chem Soc 138:15994–16003. https://doi.org/10.1021/jacs.6b09605
Bailleul S, Yarulina I, Hoffman AEJ et al (2019) A Supramolecular View on the Cooperative Role of Brønsted and Lewis Acid Sites in Zeolites for Methanol Conversion. J Am Chem Soc 141:14823–14842. https://doi.org/10.1021/jacs.9b07484
Li G, Foo C, Yi X et al (2021) Induced Active Sites by Adsorbate in Zeotype Materials. J Am Chem Soc 143:8761–8771. https://doi.org/10.1021/jacs.1c03166
Chen W, Han J, Wei Y, Zheng A (2022) Frustrated Lewis Pair in Zeolite Cages for Alkane Activations. Angew Chemie - Int Ed 61:1–7. https://doi.org/10.1002/anie.202116269
Zhou X, Wang C, Chu Y et al (2022) Mechanistic Insight into Ethanol Dehydration over SAPO-34 Zeolite by Solid-state NMR Spectroscopy. Chem Res Chinese Univ 38:155–160. https://doi.org/10.1007/s40242-022-1450-1
Acknowledgements
The authors gratefully acknowledge the support from National Petrochemical Company, Research and Technology Company (NPC-RT).
Funding
This research was supported by National Petrochemical Company, Research and Technology Company (NPC-RT) under grant number 0860240003.
Author information
Authors and Affiliations
Contributions
R.M. Behbahani and M. Nazari conceptualized and designed the study. M. Zare conducted the experiments and collected the data. M. Nazari and M. Zare analyzed the data. M. Hamidzadeh provided fundamental guidance in conducting the characteristic analysis and reactor test setup. M. Nazari wrote the draft of the manuscript. M. Behbahani and M. Hamidzadeh provided critical feedback on the manuscript. All authors have read and approved the final version of the manuscript for publication.
Corresponding authors
Ethics declarations
Ethics Approval
Not applicable.
Consent to Participate
Not applicable.
Consent for Publication
Not applicable.
Competing Interests
The authors declare no competing interests.
Additional information
Publisher's Note
Springer Nature remains neutral with regard to jurisdictional claims in published maps and institutional affiliations.
Supplementary Information
Below is the link to the electronic supplementary material.
Rights and permissions
Springer Nature or its licensor (e.g. a society or other partner) holds exclusive rights to this article under a publishing agreement with the author(s) or other rightsholder(s); author self-archiving of the accepted manuscript version of this article is solely governed by the terms of such publishing agreement and applicable law.
About this article
Cite this article
Zare, M., Nazari, M., Behbahani, R.M. et al. Comparison of Aminothermal and Hydrothermal Synthesis of SAPO-34: Impact of Synthesis Conditions on Catalyst Characteristics and MTO Catalytic Performance. Silicon 16, 177–188 (2024). https://doi.org/10.1007/s12633-023-02669-z
Received:
Accepted:
Published:
Issue Date:
DOI: https://doi.org/10.1007/s12633-023-02669-z