Abstract
This paper addresses the issue of uncertain parameters in the case of the control of nonlinear systems which are exact linearizable by state feedback. It is shown that the linearizing feedback may be complemented by an additional robustifying compensator, designed to ensure robust stability and performance against the uncertainty of some model parameters. This allows to bridge two state-of-the-art design methodologies such as exact linearization and robust control synthesis. Exact linearization allows the transformation of nonlinear dynamics into linear ones by an eventually dynamic state feedback and by a change of coordinates. However, due to the uncertain nature of some model parameters, their nominal values used in the transformation may be different from their real values. This parameter misfit implies that the resulting transformed dynamics may still include non-linearities or may be a linear system, but different from the one that results for the nominal parameter values. The paper proposes a procedure to cover the uncertainties remaining after exact linearization and to design an additional linear compensator, denoted by K(s), to ensure robust performance and stability. The design of the compensator K(s) involves standard \(\mathcal{H}_\infty\) techniques, based on an output multiplicative uncertainty structure. The weighting matrices of the output multiplicative structure are obtained such that they cover a model set obtained by linearizing the transformed nonlinear system over a sufficiently fine grid above the uncertain parameter range. The suggested approach is illustrated by multiple (SISO and MIMO) examples, including a two-degrees-of-freedom robotic arm. It is shown by simulation that the additional robustifying compensator may stabilize the system for parameter values that would result in unstable behavior without its application and may also result in a better tracking performance.
Similar content being viewed by others
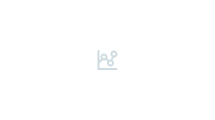
References
G. Rigatos, P. Siano, and G. Raffo, “A nonlinear H-infinity control method for multi-DOF robotic manipulators,” Nonlinear Dynamics, vol. 88, no. 1, pp. 329–348, 2017.
L. Baratchart, M. Chyba, and J.-B. Pomet, “A Grobman-Hartman theorem for control systems,” Journal of Dynamics and Differential Equations, vol. 19, no. 1, pp. 75–107, 2007.
Y. Xia, H. Huang, and K. I. Kou, “Hartman-Grobman theorem for the impulsive system with unbounded nonlinear term.” Qualitative Theory of Dynamical Systems, vol. 16, no. 3, pp. 705–730, 2017.
J. Lévine, Analysis and Control of Nonlinear Systems, Springer, 2009.
M. Fliess, J. Lévine, P. Martin, and P. Roucon, “A Lie-Backlund approach to equivalence and flatness of nonlinear systems,” IEEE Trans. on Automatic Control, vol. 44, no. 5, pp. 922–937, 1999.
D.-W. Gu, P. Petkov, and M. M. Konstantinov, Robust Control Design with MATLAB, Springer, 2013.
L. Wei-Min and J. C. Doyle, “Robustness analysis and synthesis for nonlinear uncertain systems,” IEEE Trans. on Automatic Control, vol. 42, no. 12, pp. 1654–1662, 1997.
E. M. Aylward, P. A. Parrilo, and S. J.-J. E., “Stability and robustness analysis of nonlinear systems via contraction metrics and SOS programming,” Automatica, vol. 44, no. 8, pp. 2163–2170, 2008.
H.-T. Le, S.-R. Lee, and C.-Y. Lee, “Integration model reference adaptive control and exact linearization with disturbance rejection for control of robot manipulators,” International Journal of Innovative Computing, Information and Control, vol. 7, no. 6, pp. 3255–3267, 2011.
Y Chen, G. Mei, G. Ma, S. Lin, and J. Gao, “Robust adaptive inverse dynamics control for uncertain robot manipulator,” International Journal of Innovative Computing, Information and Control, vol. 10, no. 2, pp. 575–587, 2014.
S. S. Sastry and A. Isidori, “Adaptive control of lineariz-able systems,” IEEE Trans. on Automatic Control, vol. 34, no. 11, pp. 1123–1131, 1989.
C. P. Bechlioulis and G. A. Rovithakis, “Robust adaptive control of feedback linearizable MIMO nonlinear systems with prescribed performance,” IEEE Trans. on Automatic Control, vol. 53, no. 9, pp. 2090–2099, 2008.
J. de Jesús Rubio, “Robust feedback linearization for nonlinear processes control,” ISA Transactions, vol. 74, pp. 155–164, 2018.
S. E. Li, F. Gao, D. Cao, and K. Li, “Multiple-model switching control of vehicle longitudinal dynamics for platoon-level autmation,” IEEE Trans. Veh. Technol., vol. 65, no. 6, pp. 4480–4492, 2016.
Q. Wang and R. F. Stengel, “Robust control of nonlinear systems with parametric uncertainty,” Automatica, vol. 38, no. 9, pp. 1591–1599, 2002.
M. J. Kim, Y Choi, and W. K. Chung, “Bringing nonlinear \(\mathcal{H}_\infty\) optimality to robot controllers,” IEEE Trans. on Robotics, vol. 31, no. 3, pp. 682–698, 2015.
Y.-F. Peng, “Development of robust intelligent tracking control system for uncertain nonlinear systems using \(\mathcal{H}_\infty\) control technique,” Applied Soft Computing, vol. 11, no. 3, pp. 3135–3146, 2011.
M. Lashin, M. Fanni, A. M. Mohamed, and T. Miyashita, “Dynamic modeling and inverse optimal PID with feedforward control in \(\mathcal{H}_\infty\) framework for a novel 3D pantograph manipulator,” International Journal of Control, Automation and Systems, vol. 16, no. 1, pp. 39–54, 2018.
V. Hagenmayer and E. Delaleau, “Robustness analysis of exact feedforward linearization based on differential flatness,” Automatica, vol. 39, no. 11, pp. 1941–1946, 2003.
M. Zerar, F. Cazaurang, and A. Zolghadri, “Robust tracking of nonlinear MIMO uncertain flat systems,” Proc. of the 2004 Int. Conf. on Systems, Man and Cybernetics, pp. 536–541, 2004.
L. Chen and J. Yingmin, “Output feedback tracking control of flat systems via exact feedforward linearization and LPV techniques,” International Journal of Control, Automation and Systems, vol. 17, no. 3, pp. 606–616, 2019.
B. Kiss, J. Lévine, and P. Müllhaupt, “Modelling, flatness and simulation of a class of cranes,” Periodica Polytechnica Electrical Engineering, vol. 43, no. 3, pp. 215–225, 1999.
N. Sun, Y. Fang, H. Chen, and B. Lu, “Amplitude-saturated bonlinear output feedback antiswing control for underactu-ated cranes with double-pendulum cargo dynamics,” IEEE Transactions on Industrial Electronics, vol. 64, no. 3, pp. 2135–2146,2017.
P. M. Hof, C. Scherer, and P. S. Heuberger, Model-Based Control: Bridging Rigorous Theory and Advanced Technology, Springer, 2009.
G. Balas, R. Chiang, A. Packard, and M. Safonov, Robust Control Toolbox User’s Guide, Mathworks, 2017.
M. G. Ortega, M. Vargas, C. Vivas, and F. R. Rubio, “Robustness improvement of a nonlinear \(\mathcal{H}_\infty\) controller for robot manipulators via saturation functions,” Journal of Field Robotics, vol. 22, no. 8, pp. 421–437, 2005.
H. Wang and Y. Xie, “Adaptive inverse dynamics control of robots with uncertain kinematics and dynamics,” Auto-matica, vol. 45, no. 9, pp. 2114–2119, 2009.
B. Kiss and N. Wang, “Robust exact linearization of a 2D overhead crane,” Proc. of the 12th IFAC Symposium on Robot Control, pp. 354–359, 2018.
F. Gao, D. Dang, S. E. Li, and M. Zhou, “Control of large model mismatch systems using multiple models,” International Journal of Control, Automation and Systems, vol. 15, no. 4, pp. 1494–1506, 2017.
Author information
Authors and Affiliations
Corresponding author
Additional information
Publisher’s Note Springer Nature remains neutral with regard to jurisdictional claims in published maps and institutional affiliations.
Recommended by Associate Editor Bin Jiang under the direction of Editor Milton John. The research reported in this paper was supported by the BME-Artificial Intelligence FIKP grant of EMMI (BME FIKP-MI/SC).
Na Wang received her M.S. degree in Control Engineering from Xi’an Technological University (China), in 2007. She is currently pursuing her Ph.D. studies at the Department of Control Engineering and Information Technology of the Budapest University of Technology and Economics, Hungary. Her research interests include robotics, autonomous vehicles, and non-linear control.
Bálint Kiss received his M.S. degree in electrical engineering at the Budapest University of Technology and Economics (BME, Hungary) in 1996, followed by a DEA in 1997 at Université Paris XI and by a PhD at the École des Mines de Paris (France) in 2001. He is currently an associate professor at the Department of Control Engineering and Information Technology at BME. His research interests include nonlinear control and robotics.
Rights and permissions
About this article
Cite this article
Wang, N., Kiss, B. A Method to Robustify Exact Linearization Against Parameter Uncertainty. Int. J. Control Autom. Syst. 17, 2441–2451 (2019). https://doi.org/10.1007/s12555-018-0330-4
Received:
Revised:
Accepted:
Published:
Issue Date:
DOI: https://doi.org/10.1007/s12555-018-0330-4