Abstract
Stress variations around wellbores and in the reservoirs are of much interest in subsequent drilling operations, future production, and petroleum reservoir development. Stress variations induced by in situ stresses, pore pressure, and temperature changes during drilling operations may lead to various modes of instabilities in forms of induced fractures and borehole breakouts. Previous studies of thermally induced stresses were primarily based on either assumptions of heat conduction through rock matrix or heat convection, in this case, without considering the effect of solid grain thermal conductivity. To analyze wellbore stability, in the present work, a thermo-poro-mechanical model that is fully coupled to conductive and convective transport processes is employed. Then using a two-dimensional finite element method, the stress distribution around an open borehole is investigated. Further, four rock strength criteria, Mohr–Coulomb, Drucker–Prager, modified Lade, and Mogi–Coulomb, are used to examine wellbore stability of both vertical and inclined boreholes. Results show that there are considerable differences between radial and tangential stresses simulated from models containing coupled conductive and conductive-convective transport processes. Shear stress distribution around borehole, resulted from interaction of borehole inclination and in situ stresses, is found to be an important factor affecting stability conditions of inclined wellbore. In the case of rock failure criteria, Mohr–Coulomb results in the highest values of the minimum mud weight required for wellbore stability; Drucker–Prager gives the lowest values, modified Lade, and Mogi–Coulomb result in mean values for the minimum mud weight required for wellbore stability.













Similar content being viewed by others
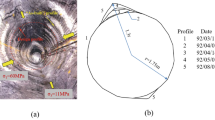
References
Al-Ajmi AM, Zimmerman RW (2004) Relation between the Mogi and the Coulomb failure criteria. Int J Rock Mech Min 42:431–439. doi:10.1016/j.ijrmms.2004.11.004
Al-Ajmi AM, Zimmerman RW (2006) Stability analysis of vertical boreholes using the Mogi-Coulomb failure criteria. Int J Rock Mech Min 43:1200–1211. doi:10.1016/j.ijrmms.2006.04.001
Bai M, Abousoleiman Y (1997) Thermoporoelastic coupling with application to consolidation. Int J Numer Anal Meth Geomech 21:121–132. doi:10.1002/(SICI)1096-9853(199702)21:2<121::AID-NAG861>3.0.CO;2-W
Bell JS, Gough DI (1979) Northeast-Southwest compressive stress in Alberta: evidence from oil wells. Earth planet Sc Lett 45:475–482. doi:10.1016/0012-821X(79)90146-8
Biot A (1941) General theory of three dimensional consolidations. J Appl Phys 12:155–164
Carr WJ (1974) Summary of tectonic and structural evidence for stress orientation at the Nevada Test Site. Open file rep, US Geological Survey 74–176. http://pubs.usgs.gov/of/1974/0176/report.pdf
Cook NGW (1979) Rock fracture: Observations and interpretations. 4th Tewksbury Symposium Melbourne Australia
Coussy O, Charlez P, Heugas O (1991) Thermoporoelastic response of a borehole. Transport Porous Med 21:121–146. doi:10.1016/0148-9062(93)91179-M
Cox JW (1970) The high resolution dipmeter reveals dip-related borehole and formation characteristics. Trans 11th SPWLA Logging Symposium D1-D26
Cui L, Cheng AHD, Abousleiman Y (1997) Poroelastic solution for an inclined borehole. J Appl Mech 64:32–38. doi:10.1115/1.2787291
Cui L, Ekbote S, Abousleiman Y, Zaman M, Roegiers JC (1998) Borehole stability analysis in fluid-saturated formations with impermeable walls. Int J Rock Mech Min 35:582–583. doi:10.1016/S0148-9062(98)00077-1
Cui L, Cheng AHD, Abousleiman Y, Roegiers JC (1999) Time-dependent failure analysis of inclined boreholes in fluid-saturated formations. J Energy Res Tech 121:31–39. doi:10.1115/1.2795057
Detournay E, Cheng AHD (1988) Poroelastic response of a borehole in a non-hydrostatic stress field. Int J Rock Mech Min 25:171–182. doi:10.1016/0148-9062(88)92299-1
Ewy R (1999) Wellbore-stability predictions by use of a modified Lade criterion. SPE Drill and Completion 14:85–91
Haimson B (2007) Micromechanisms of borehole instability leading to breakouts in rocks. Int J Rock Mech Min 44:157–173. doi:10.1016/j.ijrmms.2006.06.002
Haimson BC, Herrick CG (1985) In situ stress evaluation from borehole breakouts-experimental studies. 26th US Rock Mechanic Symposium, Rotterdam Balkema 1207–18
Haimson BC, Herrick CG (1986) Borehole breakouts-a new tool for estimating in situ stress? 1st Int Symp on Rock Stress and Rock Stress Measurement Lulea 271–81
Leeman ER (1964) The treatment of stress in rock: I. the rock stress measurement: II. Borehole rock stress measuring instrument: III. The results of some rock stress investigations. J S Afr Inst Min Meth 65(45–114):254–284
Maria VS (1997) In situ stress from breakouts in the Danish sector of the North Sea. Mar Petrol Geol 14:231–243. doi:10.1016/S0264-8172(97)00007-X
McLean M, Addis M (1990) Wellbore stability: the effect of strength criteria on mud weight recommendations. Int Proceedings of the 65th annual tech conf and exhibition SPE: 20405
McTigue DF (2003) Flow to a heated borehole in porous thermoelastic rock. Water Resour Res 26:1763–1774. doi:10.1029/WR026i008p01763
Palciauskas V, Domenico PA (1982) Characterization of drained and undrained response of thermally loaded repository rocks. Water Resour Res 18:281–290. doi:10.1029/WR018i002p00281
Papamichos E (2010) Borehole failure analysis in a sandstone under anisotropic stresses. Int J Numer Anal Meth Geomech 34:581–603. doi:10.1002/nag.824
Rice JR, Cleary MP (1976) Some basic stress diffusion solution for fluid-saturated elastic porous media with compressible constituents. Review Geophys Space Phys 14:227–241. doi:10.1029/RG014i002p00227
Santarelli FJ, Brown ET, Maury V (1986) Analysis of borehole stresses using pressure-dependent linear elasticity. Int J Rock Mech Min 23:445–449. doi:10.1016/0148-9062(86)92310-7
Song I, Haimson BC (1997) Poly axial strength criteria and their use in estimating in situ stress magnitudes from borehole breakout dimensions. Int J Rock Mech Min 34:116.e1–116.e16. doi:10.1016/S1365-1609(97)00240-2
Tang L, Luo P (1997) The effect of thermal stress on wellbore stability. India Oil and Gas Conference and Exhibition SPE: 39505
Wang Y, Dusseault MB (1995) Response of a circular opening in a friable low-permeability medium to temperature and pore pressure changes. Int J Numer Anal Meth Geomech 19:157–179. doi:10.1002/nag.1610190302
Wang Y, Dusseault MB (2003) A coupled conductive-convective thermo-poroelastic solution and implications for wellbore stability. J Petrol Sci Eng 38:187–198. doi:10.1016/S0920-4105(03)00032-9
Wang YL, Papamichos E (1994) Conductive heat flow and thermally induced fluid flow around a wellbore in a poroelastic medium. Water Resour Res 30:3375–3384. doi:10.1029/94WR01774
Yaghoubi A, Zeinali M (2009) Determination of magnitude and orientation of the in-situ stress from borehole breakout and effect of pore pressure on borehole stability—case study in Cheshmeh Khush oil field of Iran. J Petrol Sci Eng 67:116–126. doi:10.1016/j.petrol.2009.05.008
Yuan SC, Harrison JP (2006) Modeling breakout and near-well fluid flow of a borehole in an anisotropic stress field. 41st ARMA/USRMS 06–1157
Zhang L, Ping C, Radha KC (2010) Evaluation of rock strength criteria for wellbore stability analysis. Int J Rock Mech Min 47:1304–1316. doi:10.1016/j.ijrmms.2010.09.001
Zheng Z, Kemeny J, Cook NGW (1989) Analysis of borehole breakouts. J Geophys Res-Sol EA 94:7171–7182. doi:10.1029/JB094iB06p07171
Zobak MD, Moos DL, Mastin L, Anderson RN (1985) Wellbore breakout and in situ stress. J Geophys Res-Sol EA 90:5523–5538. doi:10.1029/JB090iB07p05523
Author information
Authors and Affiliations
Corresponding author
Appendix
Appendix
Rights and permissions
About this article
Cite this article
Gomar, M., Goodarznia, I. & Shadizadeh, S.R. A transient fully coupled thermo-poroelastic finite element analysis of wellbore stability. Arab J Geosci 8, 3855–3865 (2015). https://doi.org/10.1007/s12517-014-1461-4
Received:
Accepted:
Published:
Issue Date:
DOI: https://doi.org/10.1007/s12517-014-1461-4