Abstract
An enormous number of wireless sensing nodes (WSNs) are of great significance for the Internet of Things (IoT). It is tremendously prospective to realize the in-situ power supply of WSNs by harvesting unutilized mechanical vibration energy. A harmonic silicone rubber triboelectric nanogenerator (HSR-TENG) is developed focusing on ubiquitous constant working frequency machinery. The unique design of the strip serving as a flexible resonator realizes both soft contact and high and broadband output. The significant factors influencing the 1st-order vibration mode of the strip are developed for realizing the harmonic frequency adaptation to external vibration. The surface treatment of the strip improves the output performance of HSR-TENG by 49.1% as well as eliminates the adhesion effect. The HSR-TENG is able to achieve a voltage output bandwidth of 19 Hz under a vibration strength of 3.0, showing its broadband capability. The peak power density of 153.9 W/m3 is achieved and 12 × 0.5 W light-emitting diodes (LEDs) are successfully illuminated by the HSR-TENG. It can continuously power a temperature sensor by harvesting the actual compressor vibration energy. In brief, the HSR-TENG provides a promising way for constant frequency vibration energy harvesting, so as to achieve in-situ power supply for the WSNs in the vicinity.

Similar content being viewed by others
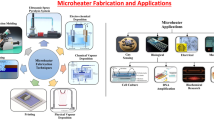
References
Mori, H.; Kundaliya, J.; Naik, K.; Shah, M. IoT technologies in smart environment: Security issues and future enhancements. Environ. Sci. Pollut. Res. 2022, 29, 47969–47987.
Liu, L.; Guo, X. G.; Lee, C. Promoting smart cities into the 5G era with multi-field Internet of Things (IoT) applications powered with advanced mechanical energy harvesters. Nano Energy 2021, 88, 106304.
Abadía, J. J. P.; Walther, C.; Osman, A.; Smarsly, K. A systematic survey of Internet of Things frameworks for smart city applications. Sust. Cities Soc. 2022, 83, 103949.
Deng, X. J.; Jiang, Y. L.; Yang, L. T.; Yi, L. Z.; Chen, J. Y.; Liu, Y.; Li, X. Y. Learning-automata-based confident information coverage barriers for smart ocean Internet of Things. IEEE Internet Things J. 2020, 7, 9919–9929.
Qiu, T.; Zhao, Z.; Zhang, T.; Chen, C.; Chen, C. L. P. Underwater Internet of Things in smart ocean: System architecture and open issues. IEEE Trans. Ind. Inform. 2020, 16, 4297–4307.
Aslam, S.; Michaelides, M. P.; Herodotou, H. Internet of ships: A survey on architectures, emerging applications, and challenges. IEEE Internet Things J. 2020, 7, 9714–9727.
Zhu, F. H.; Lv, Y. S.; Chen, Y. Y.; Wang, X.; Xiong, G.; Wang, F. Y. Parallel transportation systems: Toward IoT-enabled smart urban traffic control and management. IEEE Trans. Intell. Transp. Syst. 2020, 21, 4063–4071.
Chopade, S. S.; Gupta, H. P.; Dutta, T. Survey on sensors and smart devices for IoT enabled intelligent healthcare system. Wirel. Pers. Commun. 2023, 131, 1957–1995.
Guiloufi, A. B.; El Khediri, S.; Nasri, N.; Kachouri, A. A comparative study of energy efficient algorithms for IoT applications based on WSNs. Multimed. Tools Appl. 2023, 82, 42239–42275.
Du, T. L.; Dong, F. Y.; Xi, Z. Y.; Zhu, M. X.; Zou, Y. J.; Sun, P. T.; Xu, M. Y. Recent advances in mechanical vibration energy harvesters based on triboelectric nanogenerators. Small 2023, 19, 2300401.
Dogra, R.; Rani, S.; Babbar, H.; Krah, D. Energy-efficient routing protocol for next-generation application in the Internet of Things and wireless sensor networks. Wirel. Commun. Mob. Comput. 2022, 2022, 8006751.
Alaerjan, A. Towards sustainable distributed sensor networks: An approach for addressing power limitation issues in WSNs. Sensors 2023, 23, 975.
Liazid, H.; Lehsaini, M.; Liazid, A. Data transmission reduction using prediction and aggregation techniques in IoT-based wireless sensor networks. J. Netw. Comput. Appl. 2023, 211, 103556.
Singla, J.; Mahajan, R.; Bagai, D. An energy-efficient technique for mobile-wireless-sensor-network-based IoT. ETRI J. 2022, 44, 389–399.
Jafari, M.; Khan, K.; Gauchia, L. Deterministic models of Li-ion battery aging: It is a matter of scale. J. Energy Storage 2018, 20, 67–77.
Hayyat, M. U.; Nawaz, R.; Siddiq, Z.; Shakoor, M. B.; Mushtaq, M.; Ahmad, S. R.; Ali, S.; Hussain, A.; Irshad, M. A.; Alsahli, A. A. et al. Investigation of lithium application and effect of organic matter on soil health. Sustainability 2021, 13, 1705.
Harrop, P. Battery elimination in electronics and electrical engineering 2018–2028. GlobeNewswire: United Kingdom, 2017.
Chen, X. G.; Li, Y. M. Design, modeling, and testing of a vibration absorption device with energy harvesting based on force amplifier and piezoelectric stack. Energy Conv. Manag. 2022, 255, 115305.
Yang, T.; Zhou, S. X.; Fang, S. T.; Qin, W. Y.; Inman, D. J. Nonlinear vibration energy harvesting and vibration suppression technologies: Designs, analysis, and applications. Appl. Phys. Rev. 2021, 8, 031317.
Bansal, S.; Choi, C.; Hardwick, J.; Bagchi, B.; Tiwari, M. K.; Subramanian, S. Transmissive labyrinthine acoustic metamaterial-based holography for extraordinary energy harvesting. Adv. Eng. Mater. 2023, 25, 2201117.
Xiao, H. F.; Pan, M.; Chu, J. Y. H.; Bowen, C. R.; Bader, S.; Aranda, J.; Zhu, M. L. Hydraulic pressure ripple energy harvesting: Structures, materials, and applications. Adv. Energy Mater. 2022, 12, 2103185.
Chang, C.; He, X. Y.; Han, Z. Y.; Pei, L. L.; Wang, Z. Y.; Ji, Y. L. Harvesting thermal energy via tube-based triboelectric nanogenerators within an oscillating heat pipe. Sustain. Energy Fuels 2022, 6, 693–699.
Roundy, S.; Wright, P. K.; Rabaey, J. A study of low level vibrations as a power source for wireless sensor nodes. Comput. Commun. 2003, 26, 1131–1144.
Prajwal, K. T.; Manickavasagam, K.; Suresh, R. A review on vibration energy harvesting technologies: Analysis and technologies. Eur. Phys. J. Spec. Top. 2022, 231, 1359–1371.
Basaran, S. Hybrid energy harvesting system under the electromagnetic induced vibrations with non-rigid ground connection. Mech. Syst. Signal Proc. 2022, 163, 108198.
Zabek, D.; Pullins, R.; Pearson, M.; Grzebielec, A.; Skoczkowski, T. Piezoelectric-silicone structure for vibration energy harvesting: Experimental testing and modelling. Smart Mater. Struct. 2021, 30, 035002.
Zhao, C. Y.; Yang, Y. W.; Upadrashta, D.; Zhao, L. Y. Design, modeling, and experimental validation of a low-frequency cantilever triboelectric energy harvester. Energy 2021, 214, 118885.
Fan, F. R.; Tian, Z. Q.; Wang, Z. L. Flexible triboelectric generator. Nano Energy 2012, 1, 328–334.
Guo, X.; Shao, J. J.; Willatzen, M.; Yang, Y.; Wang, Z. L. Theoretical model and optimal output of a cylindrical triboelectric nanogenerator. Nano Energy 2022, 92, 106762.
Wang, Y.; Liu, X. Y.; Wang, Y. W.; Wang, H.; Wang, H.; Zhang, S. L.; Zhao, T. C.; Xu, M. Y.; Wang, Z. L. Flexible seaweed-like triboelectric nanogenerator as a wave energy harvester powering marine Internet of Things. ACS Nano 2021, 15, 15700–15709.
Feng, J. R.; Zhou, H. L.; Cao, Z.; Zhang, E. Y.; Xu, S. X.; Li, W. T.; Yao, H. L.; Wan, L. Y.; Liu, G. L. 0.5 m triboelectric nanogenerator for efficient blue energy harvesting of all-sea areas. Adv. Sci. 2022, 9, 2204407.
Ning, H.; Zhou, W. Y.; Tuo, L.; Liang, C. J.; Chen, C. J.; Li, S. Y.; Qu, H.; Wan, L. Y.; Liu, G. L. Tensegrity triboelectric nanogenerator for broadband blue energy harvesting in all-sea areas. Nano Energy 2023, 117, 108906.
Tcho, I. W.; Kim, W. G.; Kim, J. K.; Kim, D. W.; Yun, S. Y.; Son, J. H.; Choi, Y. K. A flutter-driven triboelectric nanogenerator for harvesting energy of gentle breezes with a rear-fixed fluttering film. Nano Energy 2022, 98, 107197.
Hu, X. Y.; Feng, J. R.; Liang, C. J.; Ning, H.; Chen, C. J.; Li, J. Y.; Wen, H. G.; Yao, H. L.; Wan, L. Y.; Liu, G. L. Round-trip oscillation triboelectric nanogenerator with high output response and low wear to harvest random wind energy. Nano Res. 2023, 16, 11259–11268.
Taghavi, M.; Sadeghi, A.; Mazzolai, B.; Beccai, L.; Mattoli, V. Triboelectric-based harvesting of gas flow energy and powerless sensing applications. Appl. Surf. Sci. 2014, 323, 82–87.
Gao, Y. Y.; Xu, B. G.; Tan, D.; Li, M. Q.; Wang, Y. T.; Yang, Y. J. Asymmetric-elastic-structure fabric-based triboelectric nanogenerators for wearable energy harvesting and human motion sensing. Chem. Eng. J. 2023, 466, 143079.
Shan, C. C.; He, W. C.; Wu, H. Y.; Fu, S. K.; Li, K. X.; Liu, A. P.; Du, Y.; Wang, J.; Mu, Q. J.; Liu, B. Y. et al. Dual mode TENG with self-voltage multiplying circuit for blue energy harvesting and water wave monitoring. Adv. Funct. Mater., in press, DOI: https://doi.org/10.1002/adfm.202305768.
Fang, L.; Zheng, Q. W.; Hou, W. C.; Gu, J. Y.; Zheng, L. A self-powered tilt angle sensor for tall buildings based on the coupling of multiple triboelectric nanogenerator units. Sens. Actuators A: Phys. 2023, 349, 114015.
He, L. X.; Zhang, C. G.; Zhang, B. F.; Yang, O.; Yuan, W.; Zhou, L. L.; Zhao, Z. H.; Wu, Z. Y.; Wang, J.; Wang, Z. L. A dual-mode triboelectric nanogenerator for wind energy harvesting and self-powered wind speed monitoring. ACS Nano 2022, 16, 6244–6254.
Wang, S. Y.; Xu, P.; Wang, X. Y.; Zheng, J. X.; Liu, X. Y.; Liu, J. H.; Chen, T. Y.; Wang, H.; Xie, G. M.; Tao, J. et al. Underwater bionic whisker sensor based on triboelectric nanogenerator for passive vortex perception. Nano Energy 2022, 97, 107210.
Zhao, H. F.; Shu, M. R.; Ai, Z. H.; Lou, Z. R.; Sou, K. W.; Lu, C. Y.; Jin, Y. C.; Wang, Z. H.; Wang, J. Y.; Wu, C. S. et al. A highly sensitive triboelectric vibration sensor for machinery condition monitoring. Adv. Energy Mater. 2022, 12, 2201132.
Zhang, X. H.; Zhao, J. Q.; Fu, X. P.; Lin, Y.; Qi, Y. C.; Zhou, H.; Zhang, C. Broadband vibration energy powered autonomous wireless frequency monitoring system based on triboelectric nanogenerators. Nano Energy 2022, 98, 107209.
Bang, J.; Moon, I. K.; Jeon, Y. P.; Ki, B.; Oh, J. Fully wood-based green triboelectric nanogenerators. Appl. Surf. Sci. 2021, 567, 150806.
Xu, M. Y.; Wang, P. H.; Wang, Y. C.; Zhang, S. L.; Wang, A. C.; Zhang, C. L.; Wang, Z. J.; Pan, X. X.; Wang, Z. L. A soft and robust spring based triboelectric nanogenerator for harvesting arbitrary directional vibration energy and self-powered vibration sensing. Adv. Energy Mater. 2018, 8, 1702432.
Yang, W. Q.; Chen, J.; Zhu, G.; Wen, X. N.; Bai, P.; Su, Y. J.; Lin, Y.; Wang, Z. L. Harvesting vibration energy by a triple-cantilever based triboelectric nanogenerator. Nano Res. 2013, 6, 880–886.
Bhatia, D.; Hwang, H. J.; Huynh, N. D.; Lee, S.; Lee, C.; Nam, Y.; Kim, J. G.; Choi, D. Continuous scavenging of broadband vibrations via omnipotent tandem triboelectric nanogenerators with cascade impact structure. Sci. Rep. 2019, 9, 8223.
Qi, Y. C.; Liu, G. X.; Gao, Y. Y.; Bu, T. Z.; Zhang, X. H.; Xu, C. Q.; Lin, Y.; Zhang, C. Frequency band characteristics of a triboelectric nanogenerator and ultra-wide-band vibrational energy harvesting. ACS Appl. Mater. Interfaces 2021, 13, 26084–26092.
Wardhana, E. M.; Mutsuda, H.; Tanaka, Y.; Nakashima, T.; Kanehira, T.; Taniguchi, N.; Maeda, S.; Yonezawa, T.; Yamauchi, M. Harvesting contact-separation-compression vibrations using a flexible and compressible triboelectric generator. Sustain. Energy Technol. Assess. 2020, 42, 100869.
Scuciato, R. F.; Carrer, J. A. M.; Mansur, W. J. Dynamic analysis of Euler–Bernoulli beams by the time-dependent boundary element method formulation. Eng. Anal. Bound. Elem. 2016, 63, 134–153.
Wang, C. Y.; Wang, C. M. Structural Vibration: Exact Solutions for Strings, Membranes, Beams, and Plates; CRC Press: Boca Raton, 2014.
Narimani, A.; Golnaraghi, M. E.; Jazar, G. N. Frequency response of a piecewise linear vibration isolator. J. Vib. Control 2004, 10, 1775–1794.
Qi, Y. C.; Liu, G. X.; Kuang, Y.; Wang, L.; Zeng, J. H.; Lin, Y.; Zhou, H.; Zhu, M. L.; Zhang, C. Frequency band broadening and charge density enhancement of a vibrational triboelectric nanogenerator with two stoppers. Nano Energy 2022, 99, 107427.
Du, T. L.; Ge, B.; Mtui, A. E.; Zhao, C.; Dong, F. Y.; Zou, Y. J.; Wang, H.; Sun, P. T.; Xu, M. Y. A robust silicone rubber strip-based triboelectric nanogenerator for vibration energy harvesting and multifunctional self-powered sensing. Nanomaterials 2022, 12, 1248.
Acknowledgments
The work was supported by the National Natural Science Foundation of China (Nos. 52101345 and 52101400), the Scientific Research Fund of Liaoning Provincial Education Department (No. LJKZ0055), the Dalian Outstanding Young Scientific and Technological Talents Project (No. 2021RJ11), and the Open Fund of National Center for International Research of Subsea Engineering Technology and Equipment (No. 3132023354).
Author information
Authors and Affiliations
Corresponding authors
Electronic Supplementary Material
Supplementary material, approximately 64.9 MB.
12274_2023_6309_MOESM4_ESM.pdf
Highly adaptive and broadband triboelectric energy harvester with stretching silicone rubber strip for variable harmonic frequency vibration
Rights and permissions
About this article
Cite this article
Du, T., Shen, D., Xi, Z. et al. Highly adaptive and broadband triboelectric energy harvester with stretching silicone rubber strip for variable harmonic frequency vibration. Nano Res. 17, 4089–4099 (2024). https://doi.org/10.1007/s12274-023-6309-3
Received:
Revised:
Accepted:
Published:
Issue Date:
DOI: https://doi.org/10.1007/s12274-023-6309-3