Abstract
Although cranial bones reliably identify the features of mammals, these traits can be affected by sex, population size and seasonality. This paper biometrically analyses the cranial features of the common vole. The research sample consisted of adult (n = 134), subadult (n = 57), of western Slovakia. Overall, software based on the highlighted marks measured 29 cranial features. Significant sexual dimorphism was observed for three features in adult individuals (width of the maxillary tooth row – right side “WMTRR”, maximum height of the mandibula excluding coronoid process “AMdm” and coronoid height of the mandibula “AMd”) and four features in subadults (length of the tooth row in the maxilla “LOSD”, “WMTRR”, length of the molar “LoM” and alveolar length of the lower molars “ALLM”). Results showed a significant positive relationship between body weight and cranial size in 18 of the 29 features. They also suggested a weak but significant positive effect of common vole abundance on cranium size (Chitty effect) likewise in 6 of the 29 features. In several cases, this effect affects, in addition to the abundance, the onset of sexual maturity of individuals.
Similar content being viewed by others
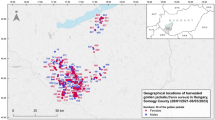
Avoid common mistakes on your manuscript.
Introduction
The use of morphological measurements has been widespread in studies of phylogenetic relationships (Zelditch et al. 2004), evolution (Lieberman 1998), reconstruction of history and structure of past populations (González-José et al. 2001), sexual dimorphism (Vincent et al. 2004), fluctuating asymmetry (Willmore et al. 2005), ecomorphology (Klingenberg and Ekau 1996), body condition (Green 2001), growth (Ackermann 2005), heritability (Kruuk et al. 2000), life histories (Bonner 1965), animal behaviour (Searcy 1979), community structure (Strong 1983) and ecological processes (Harper et al. 1970). The rodent cranium is a complex structure comprising and protecting many important organs and cranial bones easily measured and compared. Morphometric data from cranial features are an important source of information to understand many biological phenomena (Blem et al. 1993; Balčiauskiené et al. 2004).
The common vole Microtus arvalis (Pallas, 1778) is the most common and best-known of the vole species in Europe (Amori et al. 2008). The common vole is an animal native to the steppes and a key species in agricultural countries. Although mainly encountered in lowland regions, it also occurs in mountainous regions above the tree line, prefers low-growing vegetation or grassland in forests where trees have already been cut (Baláž and Tulis 2021). During the breeding season, they occupy secondary habitats such as cultivated areas where cereals, peas, rapeseed, carrots, and beans are grown. They are also found occasionally in potato and sugar beet fields (Anděra 2003; Brehm 2011; Jacob et al. 2014).
A characteristic of common voles is the extremely fast sexual and somatic development (Boyce and Boyce 1988; Bryja et al. 2005). Overbreeding is common in this species and population densities can reach more than two thousand individuals per hectare. While cyclical in some regions in Europe, in other areas the population fluctuates irregularly (Myllymäki 1977; Delattre et al. 1992; Veress 1996; Jacob et al. 2014).
More attention has been paid to the biometrics of the common vole’s somatic traits in relation to environmental variables such as seasonal changes, which have a great impact on its body proportions (Kratochvíl 1959; Reichstein 1964; Butet 1996; Devevey et al. 2008). The relationship between vole body mass and the season and altitude, and the influence of altitude on the changes in the biometry of its somatic characteristics have been studied by Baláž (2010a, b).
In the past, biometric analysis of the common vole’s cranial characteristics has been aimed at detecting sexual dimorphism. Csanády and Mošanský (2021a) by analysis in eastern Slovakia found higher values in adult females compared to males. Overall, sexual dimorphism was confirmed in seven cranial and dental traits. The results also showed a high variability of traits. Among the population in Germany, adult males had according to Bauer (2001) higher mean values than females in all cranial traits except for the length of the row of lower molars and the width of the first lower molar. In spite of this, no statistical significance was found in individually measured dental and cranial traits of males and females (Bauer 2001). Compared to the German population, common voles in Slovakia have lower mean values in almost all traits, although condylo-basal length LCb and zygomatic breadth LaZ were found to be greater in the Slovakian population (Csanády and Mošanský 2021a).
Balčiauskienė et al. (2004) observed dynamic growth in cranial characteristics and their relationship to body length, body mass, and the age of individual voles. They found the relationship between skull dimensions and body length to be closely linked, although the samples were not suitable enough to determine the age of the individuals. Differences in age and correlation with body weight and length were confirmed in the length of the maxillary and mandibular tooth rows (Balčiauskas and Balčiauskiené 2011).
Changes in the morphological and morphometric characteristics of animals may also be caused by geographic or temporal influences. Increases in weight and body length among populations of small mammals with cyclical dynamics (characteristic also in common voles) tend to occur during periods of high abundance, as they allocate their energy into the competition for resources. Conversely, in phases of lower population density, the body becomes smaller and more energy is invested in reproduction, which is known as the so-called Chitty effect (Yakushov and Sheftel 2020; Kshnyasev and Davydova 2021). This provides an explanation of natural selection which may favour genotypes with high reproduction and lower competitiveness at low densities and vice versa at high densities. The Chitty effect is a specific form of trade-off between components of fitness such as survival and reproductive success that cannot be maximised together, but only one of them can be at the cost of the other. Individuals in dense populations can be up to 20–30% larger than individuals in low population densities (Kapischke 2017; Boonstra and Krebs 1979; Krebs 1964). The effect has been documented, for example, in Microtus pennsylvanicus (Ord, 1815) (Boonstra and Boag 1987), and also in Microtus agrestis (Linnaeus, 1761) from analysis of its somatic traits (Agrell et al. 1992).
Based on these examples, the expectation was growth in the length of cranial characteristics as abundance rose, and so the presence of the Chitty effect. No investigation has ever been conducted on the impact of abundance on the size of the common vole’s cranial traits. While we have evidence of the link between abundance and body size and the link between body size and cranial traits, we do not know if this corresponds to a potential relationship between abundance and cranial traits. Thus, the objectives of our study became: 1) to analyse the cranial characteristics of the common vole; 2) to observe sexual dimorphism therein; 3) to describe the relationship between body weight and the cranial characteristics; and 4) to analyse the impact of abundance on the size of the cranial traits of the species under observation.
Material and methods
Research was conducted on a sample of skulls originating from 43 locations in western Slovakia, which had been gathered between 2011 and 2021 (Online resource 1). Material from 131 individuals came from years when species abundance culminated (2014 and 2019) and 60 individuals from years of common occurrence and species abundance (these are the years of collapse and phases between the peaks). In terms of seasonality, 34 individuals were collected in spring, 42 in summer, 63 in autumn and 52 in winter. Cranial characteristics from a total of 191 common voles were biometrically analysed, of which 99 were female (68 adults and 31 subadults) and 92 were male (66 adults and 26 sub-adults). Individuals not yet sexually active were categorised as subadults, namely males whose testes were in the abdominal position and females whose vaginas had not opened yet.
In order to obtain material for craniometrical analysis, necrophagous insects from the genus Dermestes were used to process the cadavers. The carefully cleaned common vole skulls were first photographed on millimetre paper and assigned the appropriate identification numbers. The images were then uploaded to a computer. Three categories of images were then created from tpsutil tpsUTIL (Rohlf 2004) according to the part of the skull to be examined: upper cranium, lower cranium and mandible. Individual cranial points were then marked, always in the same sequence, with tpsDIG2 (Rohlf 2008). The distances between the points located on the images were then calculated.
A total of 29 traits were observed in the skulls of the common vole (Markov et al. 2012; modified in Baláž et al. 2021), seven from the upper cranium, 15 from the lower cranium and seven characteristics from the lower jaw. These traits were measured on the upper cranium (Fig. 1): LN (length of the nasals), WR (width of the rostrum), IW (interorbital width), LaZ (zygomatic breadth), LaN (neurocranium breadth), WO (occipital width), and MW (mastoid width).
These traits were measured from a ventral view of the lower cranium (Fig. 2): BL (basal length), LPm (median palatal length), FI (length of the foramen incisivium), LD (length of the diastema), LOSD (length of the tooth row in the maxilla), WMTRL (width of the maxillary tooth row – left side), WMTRR (width of the maxillary tooth row – right side), LoM (length of the molar), LCb (condylo-basal length), PS (greatest palatal breadth), IS (breadth of the incisive bone), LFiP (length of the incisive foramen), IWAUI (incisor width across both upper incisors), LDl (length of the diastema—left), and LDr (length of the diastema – right) (Baláž et al. 2021).
The following mandibular traits were measured (Fig. 3): LMdD (length of the mandibular diastema), ML (mandible length excluding incisors), ALLM (alveolar length of the lower molars), AMdm (maximum height of the mandibula excluding coronoid process), LAP (length of the angular process), LMd (total length of the mandibula at the articular process), and AMd (coronoid height of the mandibula) (Baláž et al. 2021).
Measurement and statistical analysis
Descriptive statistics was used to evaluate the median, mean, range, standard deviation (SD) and coefficient of variation (CV) for each trait on the skull. The distribution of data was tested with the Shapiro-Wilk test. In case of a normal distribution of data, the t-test was used for the means between the sexes and the F-test was used for the equality of variances. In case the data were not normally distributed, the equality of medians was tested with the Mann-Whitney U test and the distribution was tested with the Kolmogorov-Smirnov test.
Linear regression was used to analyse the relation between the lengths of cranial traits and log-transformation of individual body weights. The analysis only covered individuals whose somatic trait data were available (because the bodies of some individuals had been compromised). For this reason, the sample size may vary for each monitored trait. PAST 4.12 was used for these statistical analyses (Hammer et al. 2001).
Linear regression was likewise used to measure the interaction of changing common vole abundances on the length of cranial traits. The impact of changing abundance and sex on the size of the observed traits was analysed with the general linear model (GLM) using R (R Core Team 2023). The analysis covered only those individuals captured at nine sites (Online resource 1) where multi-year research had been conducted to monitor changes in the abundance of the common vole population. Voles were caught in traps and their abundance was calculated per unit area. The size of the sample may vary for each observed trait (Baláž et al. 2019). We used the Bonferroni correction to adjust the p-values (new critical p-value = 0.001724) for multiple testing (Hochberg and Tamhane 1987).
Common vole abundance was calculated for different locations according to the methodology used by Zapletal et al. (2001). This was calculated on the basis of capture and assessment of abundance per area unit.
Results
The traits with the largest range of values among the adult individuals were “WMTRL” (SD = 0.11; CV = 13.87%), “WMTRR” (SD = 0.09; CV = 12.01%) and “FI” (SD = 0.49; CV = 10.37%). “LaN” (SD = 0.64; CV = 5.57%), “MW” (SD = 0.64; CV = 5.71%) and “WO” (SD = 0.77; CV = 6.04%) had the smallest variability. A demonstrably higher mean value for “WMTRR” was recorded for females (t-test: t = 2.22; p = 0.03) while, on the other hand, there were significantly higher values for “WO” in males in the median (Mann–Whitney test: U = 934.5; z = 2.71, p = 0.006) and distribution (Kolomogorov-Smirnov test: D = 0.35; p = 0.003). For “AMdm” (F-test: F = 1.66, p = 0.047) and “AMd” (F-test: F = 1.76, p = 0.041), there was a demonstrably higher variance of trait length among females. There were no differences in median or mean trait length in the remaining traits and no variance or distribution between sexes in adults (Table 1).
The traits with the largest range of values among the subadult individuals were “WMTRL” (SD = 0.10; CV = 12.80%), “WMTRR” (SD = 0.10; CV = 12.43%) and “FI” (SD = 0.53; CV = 12.25%). Traits “LaN” (SD = 0.65; CV = 5.53%), “MW” (SD = 0.64; CV = 5.65%) and “ML” (SD = 0.90; CV = 6.41%) had the smallest variability. “LOSD” (t-test: t = 2.32, p = 0.024) and “WMTRR” (F-test: t = 2.53, p = 0.014) and “LoM” (t-test: t = 2.09; p = 0.041) had demonstrable differences in mean length between the sexes with the females being longer. For “ALLM”, we observed a difference between the two sexes in the median length and the trait's distribution, where length tended to be greater in females: U = 246; z = 2.2, p = 0.028; Kolomogorov-Smirnov test: D = 0.41, p = 0.012 (Table 2).
Length–weight relationship for the cranial traits of Microtus arvalis
We tested whether the body weight interacted with cranial characteristics. In the length-weight regression analysis, we took into account only data of adult voles. The coefficient of determination (R2) indicates a relatively low dependency of weight on cranial characteristics (Table 3). R2 values around and greater than 0.30 were recorded only for “ML” (mandible length) and for traits located in the lower cranium—length of the diastema on the right and left side “LDl” and “LDr”.
Relationships of cranial trait size with abundance of adult common voles
The coefficient of determination (R2) indicates a weak relationship between abundance and the size of cranial characteristics. For only six traits – “LN”, “LD”, “LMdD”, “LD”, “LDr “and “WR” – was demonstrable effect of an increase in abundance on cranial trait length (Table 4), although R2 values greater than 0.18 were recorded only for “LN” (length of the nasals) and “LDl” (Fig. 4). The impact of the interaction between common vole abundance and sex in two age groups of individuals was documented only for “LAP”, “ML” and “LFiP” (Fig. 5; Online resource 2). In this case, however, only the cranial traits of adults grew as abundance rose. In contrast, the relationship in subadult individuals was negative.
Discussion
A common cause of sexual dimorphism in mammals is the selective advantage of those traits augmenting the ability of males to compete for females. Besides body weight, such traits include adaptations of specific components of the musculoskeletal system that enhance strength, stability and agility. The results of our study show sexual dimorphism evident in the cranial characteristics of both adult (four observed) and subadult common vole individuals in western Slovakia, specifically from the Danubian Lowlands. These findings are consistent with those of Csanády and Mošanský (2021a), whose results in eastern Slovakia likewise recorded sexual dimorphism in common voles with higher values found among the females. In eastern Slovakia, however, they also observed sexual dimorphism in different traits than what we did outside of "ALLM”. Unlike us, they did not assess dimorphism separately for adults and subadults.
Rodents are characterised by growth that, instead of slowing down as their bodies age, lasts almost to the end of their lives. The cause is the short life of rodents, with not enough time to reach a period of stabilisation (Haitlinger 1962).
As a species with cyclical dynamics, common voles additionally undergo faster sexual maturation during periods of population growth (Oli and Dobson 1999; Zapletal et al. 2001). For these reasons, cranial characteristics were measured for adults and subadults as separate categories. A single case was recorded (“WO”) of higher average lengths in adult males, which conflicted with studies of sexual dimorphism in common voles published to date. In studies of individuals from many areas within the common vole's distribution range, Stein (1957), Bauer (2001), Morris et al. (2020), and Csanády and Mošanský (2021b) described that females had higher values of cranial traits than males, showing sexual dimorphism. The larger skulls found in female common voles and other small mammal species are probably related to selection for more efficient processing of food during gestation and lactation, the generally lower quality of food available to voles, and the increased ability of a female to burrow or defend her offspring (Morris et al. 2020). Overall, however, no clear statement can be made about sexual dimorphism in the species based on cranial characteristics because some past studies have not confirmed it, e.g. Balčiauskienė et al. (2004). They noticed a larger tooth row length among females only in root voles without finding any differences between the sexes in common voles and field voles.
Several studies have mentioned a correlation of some craniological characteristics in either the body weight or other somatic traits of small mammals (Pagels and Blem 1984; Dickman et al. 1991; Goszcyński 1977; Blem et al. 1993; Trejo and Guthmann 2003; Borowski et al. 2008; Balčiauskas and Balčiauskiené 2011). Our results showed a demonstrable relationship in 18 of the 29 observed traits in both the maxilla and mandible. Nonetheless, except for “LN” and “LD”, the dependency of cranial trait length on body weight was low (R2 < 0.18). Conversely, the R2 value for the Pannonian root vole Alexandromys oeconomus mehelyi Éhik, 1928 at the three highest dependencies was greater than 0.59 (Baláž et al. 2021). Balčiauskas and Balčiauskienė (2011) noted dependency values of cranial and pelvic traits in the root vole A. o. stimmingi (Nehring, 1899) where R2 was greater than 0.7.
Balčiauskienė (2007) likewise documented a relationship between body weight and mandibular traits in bank voles. Cranial growth ex situ among bank vole individuals between the age of twelve and 724 days demonstrated dependence in all 17 cranial traits including sex. Stable growth among the bank voles continued until ten months along with body weight and length, which reached 45 g and 12 cm, respectively. Growth in a majority of cranial traits lasted 12 to 14 months while some dental features, such as the length of the first upper molar and the width of the incisors, continue growing throughout its life. Kapischke (2017) found the length of the upper row of teeth to be growing consistently with the age of the individual, where the older they were, the longer their dentition. Another study conducted by Balčiauskienė et al. (2004) confirmed the effect of age and body weight on the length of the tooth row on the upper jaw and palate in bank voles, common voles and field voles.
Population fluctuations among voles are characterised by a relatively repeatable pattern of changes in body weight and growth, age at sexual maturity and length of the breeding season (Boonstra and Krebs 1979; Krebs and Myers 1974; Boonstra and Boag 1987). Chitty (1971) postulated his own genetic behavioural hypothesis to explain it, where reciprocal interference is minimal at low and increasing densities with the selection of those non-aggressive genotypes that have high reproductive effort. At high population densities, reciprocal interference is intensive and aggressive genotypes are selected for their better performance in intraspecific competition. The presumption in Chitty's hypothesis is a genetic basis for many density-related changes. (Boonstra and Boag 1987). The Chitty effect is clearly noticeable in small mammal populations with cyclical dynamics, with the animals’ average body weight and length being greater when the population is either growing or at its peak than when it is declining (Kshnyasev and Davydova 2021; Yakushov and Sheftel 2020). The findings from our study demonstrated the impact of the growth in abundance on 6 of the common vole’s 29 cranial traits. Higher values of the coefficient of determination (R2) were recorded only in the cranial traits “LN” (length of the nasals) and “LDl (length of the diastema—left), associated with the common vole’s nasal part. In three traits, interaction between abundance and age was documented. However, growth in cranial traits in tandem with rising abundance was confirmed in these cases only among adult individuals. In subadult individuals, on the other hand, the length of traits fell with growing abundance. Balčiauskienė et al. (2004, 2009) reported smaller subadults in harsh winters than when the season was mild, while juveniles were larger. They further added that growth was markedly more inhibited and lasted longer in cranial characteristics than in body weight. Different patterns were visible in the growth of the skull itself. Some traits stopped growing over time and there were no changes in mean mandible length over a three-month period from December to February, whilst the average width at the widest part of the brain declined in January and February. The coronoid height of the mandible (AMd) grew in the period from November to December before its mean length fell in February. In both subadult and juvenile individuals, growth slowed dramatically. These findings could explain why the size of cranial traits would decrease during the winter, as more than 70% of subadult individuals originated during the winter or autumn.
To conclude, great variability can be seen in the common vole’s cranial traits, particularly in WMTRL, WMTRR, FI and WO. Differences in some traits were also found between male and female common voles as an expression of sexual dimorphism (WMTRR, AMdm and AMd in adults; LOSD, WMTRR, LoM, and ALLM in subadults). Based on the size of cranial traits (ML, LDl, LDr) and further analysis of this data could allow to predict the size of individual common voles, which may be useful in food ecology for determining the biomass of food consumed by raptors and owls from an analysis of their pellets. Our study confirms the positive impact of common vole abundance on the size of cranial characteristics, in accordance with the so-called Chitty effect. The findings from it could also be practically applied in agriculture as the larger average size of individuals would signal the onset of overbreeding as a characteristic sign of the common vole’s gradation.
References
Ackermann RR (2005) Ontogenetic integration of the hominoid face. J Hum Evol 48:175–197. https://doi.org/10.1016/j.jhevol.2004.11.001
Agrell J, Erlinge S, Sandell M (1992) Body weight and population dynamics: cyclic demography in a noncyclic population of the field vole (Microtus agrestis). Can J Zool 70:494–501. https://doi.org/10.1139/z92-074
Amori G et al (2008) Microtus arvalis. In: IUCN 2009: IUCN Red List of Threatened Species. Version 2009. 2. https://www.iucnredlist.org/species/13488/22351133
Anděra M (2003) Svět zvířat II – Savci 2. Albatros, Praha, pp 148
Baláž I (2010a) The influence of the altitude on somatic characteristics size of common vole (Microtus arvalis) in Slovakia. Ekológia (Bratislava) 29:174–181
Baláž I (2010b) Somatic characteristic and reproduction of common vole, Microtus arvalis (Mammalia: Rodentia) populations in Slovakia. Biologia (Bratislava) 65:1064–1071. https://link.springer.com/article/https://doi.org/10.2478/s11756-010-0122-7
Baláž I, Tulis F (2021) Hraboš poľný - kľúčový druh poľnohospodárskej krajiny. Naše Pole 3:32–34
Baláž I, Tulis F, Kovárová N, Šumichrast J (2019) Fluktuácia početnosti hraboša poľného na západnom Slovensku a možnosti jeho monitoringu. Ekologické Štúdie 10:25–40
Baláž I, Tulis F, Ševčík M (2021) Biometric analysis of cranial and somatic features in the Pannonian root vole. Animals 1:576. https://doi.org/10.3390/ani11020576
Balčiauskas L, Balčiauskiené L (2011) Estimation of Root Vole body mass using bone measurements from prey remains. North-West J Zool 7(1):143–147
Balčiauskiené L, Balčiauskas L, Mažeikyté JR (2004) Sex and age related differences in tooth row length of small mammals: voles. Acta Zool Lituan 14:48–57. https://doi.org/10.1080/13921657.2004.10512571
Balčiauskienė L (2007) Cranial growth of captive bred bank voles (Clethrionomys glareolus). Acta Zool Lituan 17:33–40. https://doi.org/10.1080/13921657.2007.10512813
Balčiauskienė L, Balčiauskas L, Čepukienė A (2009) Winter growth depression of common vole (Microtus arvalis). Acta Zool Lituan 19:85–92. https://doi.org/10.2478/v10043-009-0019-2
Bauer K (2001) Feldmaus Microtus arvalis (Pallas, 1778). In: Die Säugetierfauna Österreichs. Graz: Grüne Reihe des Bundesministeriums für Land- und Forstwirtschaft, Umwelt und Wasserwirtschaft, 13, pp 462–470. https://www.zobodat.at/publikation_volumes.php?id=42097
Blem CR, Blem LB, Felix JH, Holt DW (1993) Estimation of body mass of voles from crania in short-eared owl pellets. Am Midl Nat 129:282–287. https://doi.org/10.2307/2426509
Bonner JT (1965) Size and cycle: an essay on the structure of biology. Princeton University Press, Princeton. https://www.jstor.org/stable/27836213
Boonstra R, Boag PT (1987) A test of the Chitty hypothesis: inheritance of life-history traits in meadow voles Microtus pennsylvanicus. Evolution 41:929–947. https://doi.org/10.1111/j.1558-5646.1987.tb05868.x
Boonstra R, Krebs CJ (1979) Variability of large- and small sized adults in fluctuating vole populations. Ecology 60:567–573. https://doi.org/10.2307/1936077
Borowski Z, Keller M, Wlodarska A (2008) Applicability of cranial features for the calculation of vole body mass. Ann Zool Fenn 45:174–180. https://www.jstor.org/stable/23736797
Boyce CCK, Boyce JL (1988) Population biology of Microtus arvalis in lifetime reproductive success of solitary and grouped breeding females. J Anim Ecol 57:711–722. https://doi.org/10.2307/5088
Brehm A (2011) Az állatok világa 6. Kossuth kiadó, Budapest, pp 364
Bryja J et al (2005) Common vole (Microtus arvalis) population sex ratio: biases and process variation. Can J Zool 83:1391–1399. https://doi.org/10.1139/z05-133
Butet A (1996) Does food quality drive cycle in Microtus arvalis? Study on a French Atlantic marsh population. In: Mathias ML (eds.) Ecology & Behaviour, Proceedings of the European Congress of Mammalogy, Museu Bocage, Lisboa, pp 177–188
Chitty D (1971) The natural selection of self-regulatory behaviour in animal populations. Natural regulation of animal populations. p 35. https://doi.org/10.1007/978-1-349-05226-4_4
Csanády A, Mošanský L (2021a) Morphometric analysis of skull features and sexual size dimorphism in Microtus arvalis from Slovakia. Biologia 76:2913–2920. https://doi.org/10.1007/s11756-021-00764-3
Csanády A, Mošanský L (2021b) Sex and age differences in skull size in Myodes glareolus from Slovakia. Anim Biol 71:389–405. https://doi.org/10.1163/15707563-bja10061
Delattre P, Giraudoux P, Baudry J et al (1992) Land use patterns and types of common vole (Microtus arvalis) population kinetics. Agric Ecosyst Environ 39:153–168. https://doi.org/10.1016/0167-8809(92)90051-c
Devevey G, Niculita-Hirzel H, Biollaz F, Yvon C, Chapuisat M, Christe P (2008) Developmental, metabolic and immunological costs of flea infestation in the common vole. Funct Ecol 22:1091–1098. https://doi.org/10.1111/j.1365-2435.2008.01493.x
Dickman CR, Predavec M, Lynam AJ (1991) Differential predation of size and sex classes of mice by the barn owl, Tyto alba. Oikos 62:67–76. https://doi.org/10.2307/3545447
González-José R, Dahinten SL, Luis MA, Hernandez M, Pucciarelli HM (2001) Craniometric variation, and the settlement of the Americas: testing hypotheses by means of R-matrix and matrix correlation analyses. Am J Phys Anthropol 116:154–165. https://doi.org/10.1002/ajpa.1108
Goszcyński J (1977) Connection between predatory birds and mammals and their prey. Acta Theriol 22: 399–430 https://rcin.org.pl/ibs/dlibra/publication/edition/16354?id=16354&from=publication
Green AJ (2001) Mass/length residuals: measures of body condition or generators of spurious results? Ecology 82:1473–1483. https://doi.org/10.1890/0012-9658(2001)082[1473:mlrmob]2.0.co;2
Haitlinger R (1962) Morphological variability in Apodemus agrarius (Pallas 1771). Acta Theriol 6:239–255. https://doi.org/10.4098/AT.arch.62-8
Hammer Ø, Harper DAT, Ryan PD (2001) PAST: Paleontological statistics software package for education and data analysis. Palaeontol Electron 41:9. https://palaeo-electronica.org/2001_1/past/issue1_01.htm
Harper JL, Lovell PH, Moore KG (1970) The shapes and sizes of seeds. Annu Rev Ecol Syst 1:327–356. https://doi.org/10.1146/annurev.es.01.110170.001551
Hochberg Y, Tamhane AC (1987) Multiple comparison procedures. John Wiley & Sons, Inc., 605 Third Ave. New York, NY United States, p 450. https://doi.org/10.1002/9780470316672
Jacob J, Manson P, Barfknecht R, Fredericks T (2014) Common vole (Microtus arvalis) ecology and management: implications for risk assessment of plant protection products. Pest Manag Sci 70:869–878. https://doi.org/10.1002/ps.3695
Kapischke HJ (2017) Schädel- und Zahnmerkmale von Feldmäusen aus Waldohreulengewöllen, Teil 3 (Dresden im Winter 2009/2010 bis 2012/2013). In: Mitteilungen für sächsische Säugertierenfreunde, Naturschutzbund Deutschland (NABU), Leipzig, pp 22–34 https://sachsen.nabu.de/tiereundpflanzen/saeugetiere/34485.html
Klingenberg CP, Ekau W (1996) A combined morphometric and phylogenetic analysis of an ecomorphological trend: pelagization in Antarctic fishes (Perciformes: Nototheniidae). Biol J Linn Soc 59:143–177. https://doi.org/10.1006/bijl.1996.0059
Kratochvíl J (1959) Hraboš polní Microtus arvalis. Nakladatelství Československé akademie věd. Praha, pp 357
Krebs CJ (1964) Cyclic variation in skull–body regressions of lemmings. Can J Zool 42:631–643. https://doi.org/10.1139/z64-054
Krebs CJ, Myers JH (1974) Population cycles in small mammals. Adv Ecol Res 8:267–399. https://doi.org/10.1016/s0065-2504(08)60280-9
Kruuk LEB, Clutton-Brock TH, Slate J, Pemberton JM, Brotherstone S, Guinness FE (2000) Heritability of fitness in a wild mammal population. Proc Natl Acad Sci USA 97:698–703. https://doi.org/10.1073/pnas.97.2.698
Kshnyasev I, Davydova Y (2021) Population cycles and the Chitty syndrome. Russ J Ecol 521:70–75. https://doi.org/10.1134/S1067413621010082
Lieberman DE (1998) Sphenoid shortening and the evolution of modern human cranial shape. Nature 393:158–162. https://doi.org/10.1038/30227
Markov G, Csorba G, Kocheva M, Gospodina M (2012) Skull features of the common vole (Microtus arvalis sensu lato) from Hungary: craniometrical evidence for its taxonomic detachment. Turk J Zool 36:283–290. https://doi.org/10.3906/zoo-1002-49
Morris JS, Rogers N, Rogers AR, Carrier DR (2020) Sexual dimorphism in skeletal shape in voles (Arvicolinae): disparate selection on male bodies and female heads. J Mammal 101:951–957. https://doi.org/10.1093/jmammal/gyaa076
Myllymäki A (1977) Demographic mechanisms in the fluctuating populations of the field vole Microtus agrestis. Oikos 29:468–493. https://doi.org/10.2307/3543588
Oli MK, Dobson FS (1999) Population cycles in small mammals: the role of age at sexual maturity. Oikos 86:557–565. https://doi.org/10.2307/3546660
Pagels JF, Blem CR (1984) Prediction of body weights of small mammals from skull measurements. Acta Theriol 29:367–381 https://rcin.org.pl/ibs/dlibra/publication/edition/11104?id=11104&from=publication
R Core team (2023) R: A language and environment for statistical computing. R Foundation for Statistical Computing, Vienna. https://www.R-project.org/
Reichstein H (1964) Untersuchungen zum Körperwachstum und zum Reproductionspotential der Feldmaus, Microtus arvalis (Pallas, 1779). Z Wiss Zool 170:112–222
Rohlf FJ (2004) TpsUtil, Version 1.44. Department of ecology and Evolution, State University of New York at Stony Brook, New York, USA. http://life.bio.sunysb.edu/morph/index.html
Rohlf FJ (2008) TpsDig2, Version 2.10. Department of Ecology and Evolution, State University of New York at Stony Brook, New York. http://life.bio.sunysb.edu/morph
Searcy WA (1979) Morphological correlates of dominance in captive male red-winged blackbirds. Condor 81:417–420. https://doi.org/10.2307/1366971
Stein GHW (1957) Materialien zür Kenntnis der Feldmaus, Microtus arvalis. Z. Säugetierkunde 22:117–135
Strong DR Jr (1983) Natural variability and the manifold mechanisms of ecological communities. Am Natur 122:636–660. https://www.jstor.org/stable/2460846
Trejo A, Guthmann N (2003) Owl selection on size and sex classes of rodents: activity and microhabitat use of prey. J Mammal 84:652–658. https://doi.org/10.1644/1545-1542(2003)084%3c0652:OSOSAS%3e2.0.CO;2
Veress I (1996) Pannon Enciklopédia – Magyarország állatvilága. Dunakanyar 2000 Könyvkiadó. Budapest, pp 336
Vincent SE, Herrel A, Irschick DJ (2004) Sexual dimorphism in head shape and diet in the cottonmouth snake (Agkistrodon piscivorus). J Zool 264:53–59. https://doi.org/10.1017/S0952836904005503
Willmore KE, Klingenberg CP, Hallgrimsson B (2005) The relationship between fluctuating asymmetry and environmental variance in rhesus macaque skulls. Evolution 59:898–909. https://doi.org/10.1111/j.0014-3820.2005.tb01763.x
Yakushov VD, Sheftel BI (2020) Is there a relationship between the Chitty effect and the types of population dynamics? Dokl Biol Sci 492:89–92. https://doi.org/10.1134/S0012496620030084
Zapletal M, Obdržálková D, Pikula J, Zejda J, Pikula J, Beklová M, Heroldová M (2001) Hraboš polní, Microtus arvalis (Pallas, 1778) v České republice. Akademické nakladatelství CERM, Brno, p 128
Zelditch ML, Swiderski DL, Sheets HD, Fink WL (2004) Geometric Morphometrics for biologists: a primer. Elsevier Academic Press, New York. https://www.sciencedirect.com/book/9780127784601/geometric-morphometrics-for-biologists
Acknowledgements
The study has been conducted with the support of grant project VEGA 1/0080/23. We thank anonymous reviewers for valuable comments and suggestions to an earlier version of the manuscript.
Funding
Open access funding provided by The Ministry of Education, Science, Research and Sport of the Slovak Republic in cooperation with Centre for Scientific and Technical Information of the Slovak Republic.
Author information
Authors and Affiliations
Corresponding author
Ethics declarations
Ethics approval
The study was approved by the institutional research ethics committee (The Ethics Committee of the Constantine the Philosopher University in Nitra) and the authors certify that the study was performed in accordance with the ethical standards as laid down in the 1964 Declaration of Helsinki and its later amendments or comparable ethical standards. Trapping of small mammals was approved by the Ministry of Environment of the Slovak republic in accordance with permission MŽP SR no. 4850/2019-6.3.
Conflict of interest
The authors have no relevant financial or non-financial interests to disclose.
Additional information
Publisher's Note
Springer Nature remains neutral with regard to jurisdictional claims in published maps and institutional affiliations.
Supplementary information
Below is the link to the electronic supplementary material.
Rights and permissions
Open Access This article is licensed under a Creative Commons Attribution 4.0 International License, which permits use, sharing, adaptation, distribution and reproduction in any medium or format, as long as you give appropriate credit to the original author(s) and the source, provide a link to the Creative Commons licence, and indicate if changes were made. The images or other third party material in this article are included in the article's Creative Commons licence, unless indicated otherwise in a credit line to the material. If material is not included in the article's Creative Commons licence and your intended use is not permitted by statutory regulation or exceeds the permitted use, you will need to obtain permission directly from the copyright holder. To view a copy of this licence, visit http://creativecommons.org/licenses/by/4.0/.
About this article
Cite this article
Baláž, I., Csákiová, Ž. & Tulis, F. Biometric analysis of the cranial features of common voles. Biologia (2024). https://doi.org/10.1007/s11756-024-01784-5
Received:
Accepted:
Published:
DOI: https://doi.org/10.1007/s11756-024-01784-5