Abstract
The objective of this study is to explore the preparation and application of sludge produced in plants of drinking water treatment, to remove methylene blue (MB) dye from polluted water. Adsorbate (methylene blue) is prepared in the lab. as a stock solution. To create new adsorbents, chemical activation of the used sludge has been carried out. The activation is performed by HCl and H2O2 and a mixture of HCl and H2O2. The experiments were carried out under different boundary conditions, like the initial concentration of dye, dosage of adsorbent, solution pH, contact time, and temperature of the adsorption, to determine the optimum boundary conditions for operation. All of the experiments in this study were carried out in a batch system. The results show that sludge activation by a mixture of 2:1 HCl/ H2O2 was selected as the best chemically activated adsorbent (removal of 99.9%). For the best MB adsorption results, the following criteria must be met: 50 ppm initial dye concentration, 90 min of contact time, pH 7, and a dosage of 0.25 g of the adsorbent. Adsorbents suit the Langmuir and Freundlich models well, according to applications of the Freundlich Isotherm and Langmuir models.
Similar content being viewed by others
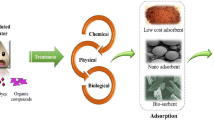
Avoid common mistakes on your manuscript.
1 Introduction
Plants of drinking water treatment produce a huge amount of sludge. In Egypt, the water treatment industry uses approximately 365,000 tonnes of aluminium sulphate as a coagulant and produces more than 100 million tonnes of semidried sludge per year (Abd El-Razak, et al., 2016). This large volume of sludge is typically considered waste and should be disposed of by friendly environmental methods. The most common method of sludge disposal is landfill. However, the reduction in required landfill sites, the increase in disposal costs, and the concept of achieving zero-waste have driven researchers to develop a new approach to sludge management and disposal (Minh et al., 2022). Drinking water treatment sludge (DWTS) can be reused in different applications, such as reused in land applications and agriculture fields as a soil conditioner to enhance the soil structure and its characteristics that lead to increased crop production (Lombi et al., 2010). Another application of DWTS is reused as an adsorbent of pollutants such as phosphorus, which can cause eutrophication in freshwater bodies and in turn cause several problems for the treatment plant of water, such as clogging of filters, odour, and taste of treated water, and habitat and human health issues (Karunanithi et al., 2015, and Tekile et al., 2015). Heavy metals are pollutants that can be removed using DWTS as an adsorbent. Yusuff et al. (2019) studied the pH dependency of heavy metal adsorption by DWTS. Other pollutants can be removed by DWTS like; fluoride (Sujana et al., 1998), perchlorate (Makris et al., 2006a, b), hydrogen sulphide (Ren et al., 2020), sulphur dioxide (Sirisha et al., 2012), and carbon dioxide (Yusuff et al., 2019). DWTS can also be reused in construction materials such as pavement, ceramic products, lightweight aggregates, and concrete (De Carvalho et al., 2019).
On the other hand, industrial dyes are one of the dominant chemicals that make water unsuitable for drinking. (Bouras et al., 2020) reported that more than 100,000 commercial dyes are used worldwide, which can be estimated at approximately by the amount of 1 × 109 kg/year. Dyes can be used in different fields such as textiles, printing, cosmetics, plastic, medicine, rubber, food, concrete, and paper industries for various purposes (Alencar et al., 2020, and Ahmad et al., 2021). Methylene blue (MB) is among these dyes and is considered one of the most consumed materials in the dye industry. MB is toxic, carcinogenic, and considered non-biodegradable, which in turn can cause a severe threat to human health and environmental safety (Khan et al., 2022).
Because of the negative environmental impact of MB, many researchers have investigated various methods for removing MB from wastewater. These methods include, but are not limited to, adsorption technique (Wang et al., 2020; Li et al., 2020); coagulation (Lau et al., 2015); electrocoagulation (Mahmoud et al., 2013, and Tir et al., 2015); ultrafiltration (Kim, et al., 2020; Zheng et al., 2009); nanofiltration (Kong et al., 2019, and Zhong et al., 2019); microwave treatment (Garcia et al., 2017); biodegradation (Eslami et al., 2017); and vacuum membrane distillation (Banat et al., 2005); Each of these treatment techniques has its advantages and its drawbacks. The optimum technique can be selected based on a comparison of efficiency, environmental impact, capital cost, and feasibility.
Sludge reclaimed from the drinking water treatment plant was used for the treatment of wastewater containing dyes. Rashed et al. (2016) used a modified adsorbent from drinking water treatment sludge to remove methylene blue (MB) dye from an aqueous solution. The sludge adsorbents were prepared by activating the sludge with nitric acid (0.25 M HNO3) and the other by pyrolysis at 700 °C. Cardoso et al. (2019) prepared an adsorbent from drinking water treatment plant sludge by chemical activation with K2CO3/CH3COOH. Poormand et al. (2017) used water treatment sludge modified with sodium alginate for the adsorption of methylene blue from aqueous solutions. Valério et al. (2020) prepared activated carbon adsorbent from water treatment plant sludge and used it for the removal of methylene blue (MB) from wastewater. Rashed et al. (2020) prepared drinking water treatment sludge @ TiO2 composite and apply it for photocatalytic degradation of methylene blue dye from a polluted solution.
In this paper, new adsorbents will be prepared from the waste of a drinking water treatment plant (sludge) by activation with HCl acid or H2O2 or a mixture of both. The best-prepared adsorbent will be used for the removal of methylene blue dye from industrial wastewater.
2 Materials and Methods
2.1 Sample Collection
Sludge from a drinking water treatment plant (DWTP) was collected from the drinking water treatment plant (alum was used) in Aswan governorate, Egypt. The samples of sludge were air-dried in a spotless area, ground to a uniform size of 0.2 mm using an agate mortar, and then dried in an oven at 105 °C for 24 h. The samples were stored in the desiccators after cooling until use.
2.2 Preparation of Sludge Adsorbents
2.2.1 Chemical Activation of the Sludge with Hydrochloric Acid
Ten grams of DWTS dried sample was mixed with 50 mL of different hydrochloric acid (HCl) concentrations (0.01, 0.05, 0.10, 0.15, 0.20, 0.25, 0.50, 1.00, 1.50 M), stirred for 1 h at room temperature, then filtered and left for 24 h. After that, the mixture labelled “chemically activated sludge” (AS) is washed with distilled water, then dried at 60 °C for 24 h and kept in desiccators for further uses (Rashed et al., 2022). The prepared adsorbents labelled AS-HCl 1, AS-HCl 2, AS-HCl 3, AS-HCl 4, AS-HCl 5, AS-HCl 6, AS-HCl 7, AS-HCl 8, and AS-HCl 9 for the HCl concentrations of 0.01, 0.05, 0.10, 0.15, 0.20, 0.25, 0.50, 1.00, and 1.50 M, respectively.
2.2.2 Chemical Activation of the Sludge with Hydrogen Peroxide
Five grams of DWTS dried sample was mixed with 40 mL of different hydrogen peroxide (H2O2) concentrations (0.01, 0.05, 0.10, 0.15, 0.20, 0.25, 0.30, 0.40, and 0.50 M), stirred at room temperature for 30 min, then filtrated, and washed with distilled water under vacuum filtration until the pH was \(\cong\) 7 finally. The leached reactants were dried in the oven at 105 °C.
The prepared adsorbents labelled AS- H2O2 1, AS- H2O2 2, AS- H2O2 3, AS- H2O2 4, AS- H2O2 5, AS- H2O2 6, AS- H2O2 7, AS- H2O2 8, and AS- H2O2 9 for the H2O2 concentrations of 0.01, 0.05, 0.10, 0.15, 0.20, 0.25, 0.30, 0.40, and 0.50 M, respectively.
2.2.3 Chemical Activation of the Sludge with Mixture of Hydrochloric Acid and Hydrogen Peroxide
Ten grams of DWTS dried sample was mixed with 50 mL mix (1:1, 1:2, 2:1) of (HCl (1 M)/ H2O2 (0.25 M)) for 1 h, then filtered and left for 24 h. After that, it was washed with distilled water, dried at 60 °C in an oven for 24 h and kept in desiccators for further use. The prepared adsorbents labelled ASm1 HC/H2O2, ASm2 HC/H2O2, ASm3 HC/H2O2, for the mixture HCl (1 M)/ H2O2 (0.25 M) 1:1, 1:2, 2:1, respectively.
2.3 Preparation of Adsorbate(Methylene Blue)
The tock solution of methylene blue (MB) dye was prepared by dissolving 1gm of (MB) in 1000 mL of distilled water (MB 1000 ppm). The standard calibration curve of known concentration of MB dye was plotted by finding out the absorbance at the characteristic wavelength (579.5 nm). A spectrophotometer was used for the calibration plot.
2.4 Morphological Features
SEM–EDX, JEOL JSM-5500LV electron microscopy instruments operating at five kV specimens on which a thin layer of gold or carbon had been evaporated were used to analyse the micro-morphology of raw sludge and treated sludge. Using a Philips X-ray diffraction device with filtered Cu K radiation (n = 1.5418) and operating at 40 kV and 40 mA, X-ray diffraction (XRD) of the adsorbents was obtained. From 1.5 to 50° 2 with a scanning rate of 0.02° 2 per second, the XRD pattern was captured. The surface area of the adsorbent was measured.
2.5 Adsorption Experiments
The adsorption study was carried out using the prepared adsorbents at constant contact time (90 min), initial concentration of MB dye (50 ppm), adsorbed dosage (0.25 gm), room temperature 25 °C, and pH = 7 at rpm = 250.
To adjust pH for the dye solution 0.1 M HCl or 0.1 M NaOH was used. All samples’ solutions were filtered through Goach. The concentrations of MB dye in treating samples were determined by a UV spectrophotometer at a wavelength (579.5 nm).
The percentage removal (R%) of MB dye was calculated by the equation:
where Co = initial concentration of dye (ppm), and Ct = the equilibrium concentration (ppm).
2.6 Selection of the Best Adsorbent
For selecting the best adsorbent for the removal of MB dye from its solution, the following experiments were applied. 0.5 g of each of the prepared chemically activated sludge samples (AS HCl, AS H2O2, AS HCl/H2O2) were stirred with 50 mL of 50 ppm MB dye for 12 h and the pH solution was adjusted at pH 6 using HNO3 or NaOH solution. The mixture was then filtered and the remaining MB dye in the filtrate was measured by atomic absorption spectrophotometer at 579.5 nm.
2.7 Batch Experiments
The effects of solution pH, initial MB concentration, adsorbent dose, and contact time on the MB dye adsorption were studied in batch tests.
2.7.1 Effect of Initial Dye Concentration
The initial concentration of the dye solution was 10, 30, 50, 70, and 90 ppm. A total of 0.25 g of adsorbent was placed in the Erlenmeyer flask with 100 mL of methylene blue solution. The mixture was agitated for 90 min at a temperature 25° C and pH 7. The reaction mixture was filtered by a Goach funnel, and the methylene blue concentration was analysed using a spectrophotometer.
2.7.2 Effect of pH
At different pH levels (3, 5, 7, 9, and 11), the impact of starting pH was examined. 0.25 gm of adsorbent was mixed with 100 mL 50 ppm methylene blue at 90 min contact time, filtrated, and the MB concentration was measured by spectrophotometer.
2.7.3 Effect of Contact Time
A total of 0.25 g of adsorbent was added to 100 mL of 50 ppm methylene blue at pH 7 and solution temperature 25 °C to determine the ideal contact time for MB dye removal. The mixture was shaken at different times (20, 40, 60, 90, and 120 min). After a specific time, the samples were filtered, and the equilibrium MB concentration was measured by spectrophotometer.
2.7.4 Effect of Dosage Adsorbent
A total of 100 mL of 100 ppm methylene blue solution was added to various dosages of adsorbent (0.05, 0.10, 0.15, 0.25, 0.30, and 0.40 g) at contact time 90 min, 25 °C, and pH 7. After filtration, the concentration of MB was measured by a spectrophotometer.
The following equation was used to calculate the amount of methylene blue adsorbed at equilibrium per unit mass of adsorbent qe (mg/g):
where Co is the initial concentration of methylene blue (ppm), Ce is the equilibrium concentration of the MB (ppm), V is the volume of the MB solution (L), m is the mass of the adsorbent (g), and qe is the methylene blue quantity adsorbed at equilibrium (mg/g).
The removal percentage of methylene blue dye was calculated as follows:
2.8 Analytical Technique
Using a UV–Vis spectrophotometer (model Thermo Scientific Nicolet Evolution 100), the equilibrium concentrations of the solution sample before and after adsorption were examined. The absorbance values of various concentrations of methylene blue dye at their maximum wavelength (579.5 nm) were recorded to create the methylene blue standard calibration curve. An Instrumental pH meter (Jenway 3510) and magnetic stirrer were used.
3 Results and Discussion
3.1 X-ray Diffraction of the Developed Adsorbent AS3 HC/H2O2 (ASm3)
Adsorbent ASm3 underwent XRD analysis both before and after activation to determine the structural changes of the generated adsorbent samples. X-ray diffraction (XRD) patterns of the adsorbents were obtained using Brucker D8 Discover instrument with filtered CU kɑ radiation (n = 1.5406A°). The XRD pattern recorded the values of 2ɵ from 0 to 80°2ɵ.
In a previous study, alum sludge before activation confirmed the presence of quartz (SiO2), albite (NaAlSi3O8), and microcline (KAlSi3O8) (Rashed et al. 2016).
The sludge after activation by mixing HCl(1 M):H2O2(0.25 M) (ratio 2:1) exhibited quartz, albite, microcline, cristobalite, and calcite. From Fig. 1, high intensity of peaks at 2ɵ = (19.11, 19.545, 20.98, 22.362°) while the lower intensity of beak at 2ɵ = (14.173, 14.686, 15.984) for cristobalite was observed, quartz has high intensity at 2ɵ = (21) but it has lower intensity peak than cristobalite, but albite and has lowered intensity peak at 2ɵ = (23, 25, 36, and 38°) as determined by (JCPDS:01–0739).
Moreover, it was noticed that the peak at 2ɵ = (15, 28, and 29°) refers to the presence of microcline and calcite shows a low-intensity peak at 2ɵ = (30, 36, and 50°). Cristobalite has the same chemical formula as quartz, SiO2, but a distinct crystal structure. It has a diamond structure but with linked tetrahedra of silicon and oxygen where the carbon atoms are in the diamond. Its crystal habit is octahedra or spherulites, which can reach a size of several centimeters.
3.2 Raman analysis of the Sludge Adsorbent (ASm3)
The vibrational modes of molecules, although rotational and other low-frequency methods. The instrument used in measurement is the LabRAM HR EVOLUTION Raman spectrometer.
A sample is exposed to a single wavelength of light during a Raman spectroscopy experiment, typically via a laser that emits light in the visible, infrared, or ultraviolet spectrum. The sample then scatters the laser light. Simply put, scattering is the process whereby light particles (photons) are compelled to diverge from their original course due to some interaction with the sample. The energy of the laser photons is shifted up or down in Raman spectroscopy as a result of the laser light’s interaction with molecular vibrations (e.g. Raman shift), and the shift in energy provided useful information about the vibrational modes in the system and can be used to identify the type of bonds present in the compound.
In this study, the Raman spectra of the prepared adsorbent (ASm3) was presented in Fig. 2, which showed that the characteristic band at 423.08 cm−1 corresponding to Hydroxyl chloride (Fe2(OH)3CL), at (700–1224) cm−1 corresponding to C–C stretching vibration due to highly mixed in complex molecule and ring breathing (tryptophan), also 1001.5 cm−1 corresponding to C–CH3 deformation, at 1092.48 cm−1 also corresponding to symmetric dioxy stretching vibration the phosphate indicative to Nucleic acid, at 1348 cm−1 corresponding to Ferrihydrite, at 1495.44 cm−1 corresponding to CH3 and CH2 deformations, at 1565.5 cm−1 corresponding to C–C stretching vibration, at 1600 cm−1 corresponding to C = C Aryling indicative to lignin, at (1630 and 1684) cm−1 corresponding to > C = O mixed with NH deformation indicative to Amidel, at 1731 cm−1 corresponding to > C = O remark change for Ketone, aldehyde and ester, at (1851.13–2032) cm−1 C cm−1 = C and C = O; amide I. (2110.6–2257.8) cm−1 corresponding to C–C, at ( 2725.7, 2752, 2794.2828, 2900.3 and 2917) cm−1 corresponding to CH peak regions. At 2968 cm−1 corresponding to CH3 asymmetric, at (3000.5–3280.13) cm−1 corresponding to OH stretching indicative to broadened and shifted by H-bond and CH stretching and water peaks (> 2700 cm−1).
3.3 Surface Area Measurement
Since surface area can affect a material’s effectiveness and reactivity, its measurement is crucial for comparing and evaluating materials for these purposes. Quantachrome company model Novatouch xl2 instrument is used for the surface area measurement. The Brunauer–Emmett–Teller (BET) method is the most common one for measuring the surface area of materials and is involved in measuring how much gas is adsorbed onto the surface of the material in question, normally nitrogen gas is used for this purpose.
The specific surface area of the prepared adsorbents AS-HCl(1 M), AS-H2O2(0.25 M), and ASm3 (2:1) were calculated from the N2 adsorption capacity using the Brunauer–Emmett–Teller (BET) equation. The results (Table 1) revealed that ASm3 exhibited a higher surface area (15.986 m2\g) than the other adsorbents. So, ASm3 (2:1) will be used for further experiments.
3.4 Scanning Electron Microscopy Images of the Developed Adsorbent ASm3 (2:1)
Scanning electron microscopy (SEM) is used to compare the surface structure of the developed adsorbent ASm3 (2:1) before and after chemical activation (SEM) (Fig. 3). The picture showed the presence of amorphous structures with a cylindrical shape and mesosize pores. During the activation, the morphological structure, pore size, and pore shape all stay unaltered.
3.5 Chemical Activation Processes of the Sludge
The chemical activation of the sludge with HCl (Table 2) revealed that the adsorption of MB was the highest (99.2%) using an adsorbent treated with 1 M HCl (AS-HCl 8). The chemical activation of the sludge with H2O2 (Table 3) revealed that the adsorption of MB was the highest (99.6%) using an adsorbent treated with 025 M H2O2 (AS—H2O2 8). The chemical activation of the sludge with the mixture HCl/H2O2 (Table 4) revealed that the adsorption of MB was the highest (99.9%) using adsorbent treated with mixture 2:1 HCl/H2O2 (ASm3).
So, the adsorbent ASm3 (2:1 HCl/H2O2) was selected as the best chemically activated sludge and used for further experiments.
3.6 Batch Adsorption
3.6.1 Initial MB Dye Concentration Effect on Adsorption
Figure 4 illustrates the adsorption data for the removal of MB by ASm3 (2:1 HCl/H2O2) adsorbent at various MB concentrations (10, 30, 50, 70, and 90 ppm) under fixed experimental conditions.
The adsorption data in Fig. 4 revealed that the removal percentage of MB dye with ASm3 adsorbent increased with increasing concentration from 10 to 50 ppm after that it decreased up to 90 ppm. So that the 50 ppm as a concentration of MB dye was optimum to achieve maximum adsorption of MB on the surface of the ASm3 adsorbent.
As a result, a rise in MB concentration leads to an increase in the adsorption capacity of MB on the ASm3 adsorbent surface. Thus, the effectiveness of the adsorption process was marginally influenced by the starting dye concentration. However, as the dye concentration increased at a fixed adsorbent dosage, the percentage of dye removal decreased. This might be explained by the concurrent rise in dye aggregation and/or the reduction of the coagulant's accessible hydrolysis products (Rashed et al., 2022). This suggests that the initial dye concentration has a significant impact on the dye's ability to adsorb.
According to Gurses et al. (2006), as the starting dye concentration increased from 10 to 100 mg/L, the adsorption capacity of MB dye on surface clay increased from 9.2 to 58.3 mg/g.
3.6.2 Effect of pH on Dye Adsorption
The findings showed that pH has a substantial impact on the adsorption process. Figure 5 illustrates the adsorption results for the removal of MB by ASm3 adsorbent at different pH levels (3, 5, 7, 9, and 11) under fixed experimental conditions. The result (Fig. 5) shows in demonstrated that an increase in pH from 3 to 7 marginally increased the removal percentage of MB by ASm3 adsorbent from 99.7 to 99.9%. As the pH increased from 7 to 11, the efficiency of the dye’s removal decreased. So, the optimum pH for the removal of MB dye was 7.
MB dye is a cationic dye that manifests as positively charged ions in aqueous solutions. It was proposed that the increase of MB adsorption at acidic pH was caused by extra H + ions, which are required for the dye cation to complete the adsorption site. Electrostatic attraction favours the adsorption of MB when there are fewer positively charged sites and more negatively charged sites present. Because of the formation of a soluble hydroxyl complex between the adsorbent and the dye, the adsorption rate decreases in solutions with higher pH values. According to Yasin et al. (2007), an increase in pH solution led to a greater removal of MB from treated activated carbon (in the acidic range). A similar result was reported for the adsorption of MB on wood shavings (Janos et al., 2009), spent tea leaves (Ihsan 2013), and sunflower seed hulls (Hameed 2008).
3.6.3 Effect of Adsorbent Dose on Dye Adsorption
The results (Fig. 6) showed that the removal percentage of MB dye with ASm3 adsorbent increased with increasing adsorbent dose from 0.05 to 0.25 gm, after that it decreased up to 0.4 gm. So that the 0.25 gm as dosage adsorbent was optimum to achieve maximum adsorption of MB on the surface of ASm3 adsorbent.
According to Ihsan (2013), increasing the dosage of the adsorbent resulted in an increase in the removal percentage of MB. This increase can be attributed to the adsorbent's increased surface area and the availability of additional adsorption sites, but the adsorption decreases with an increase in adsorbent dose because there is a split in concentration gradient between the dye concentration in solution and that on the surface of adsorbent (Kavitha and Sentamilselvi, 2015).
A similar result was observed by Mall et al. (2005) for the removal of malachite green dye from solution by bagasse fly ash and activated carbon. Also, the same was reported by Mane et al. (2011) for the adsorption of Brilliant green on treated sawdust. Gurses et al. (2006) reported that increasing the sorbent dosage (clay) related to an increase in the MB adsorption capacity; while the sorbent dosage increased from 0.1 to 0.3 gm, the adsorption capacity of dye (MB) on clay changed from 57.1 to 66.1 mg/g. Increasing the adsorbent dosage at a fixed MB concentration provided more available adsorption sites for MB and thus increased the extent of MB removal. According to Reham and Mahmud (2013), 0.4 gm of Ricinus Communis stem powder had the highest MB adsorption (94%). According to Saiful et al. (2005), increasing the adsorbent dosage of powdered activated carbon led to an increase in the percentage of MB dye removal.
3.6.4 Effect of Contact Time on Dye Adsorption
The results in Fig. 7 showed that the removal percentage of MB dye with ASm3 adsorbent increased with increasing contact time from 20 to 90 min, after that it decreased up to 120 min. So the 90 min contact time was optimum to achieve maximum adsorption of MB on the surface of ASm3 adsorbent.
The fast adsorption at the initial stage may be due to the higher driving force that causes the fast transfer of MB dye to the surface of adsorbent particles and the availability of the uncovered surface area and active sites on the adsorbent. With increasing time, the availability of the uncovered surface area and the remaining active sites decreased, which may be explained by the saturation of the adsorbent surface.
According to Yasin et al. (2007), the MB removal with treated activated carbon increased with an increase in contact time. According to Reham and Mahmud (2013), the equilibrium for the greatest amount of MB (76%) adsorption on Ricinus Communis Stem Powder was reached after 65 min.
3.6.5 General Removal of MB Dye from Polluted Samples
According to the experimental results of the optimum conditions for the adsorption of MB dye from polluted solution, polluted water with MB dye can be treated using the prepared adsorbent (chemically treated sludge of drinking water treatment plant) at contact time 90 min, 0.25 g adsorbent dose, pH 7, and solution temperature 25 °C.
3.7 Adsorption Isotherms
In this work, Langmuir and Freundlich models were used to fit the data. The equilibrium adsorption of adsorbate (qe) on the surface of the adsorbent is expressed as
where co is expressed as the initial concentration of adsorbate (ppm), ce is expressed equilibrium concentration of adsorbate (ppm), v is expressed volume of adsorbate solution in L, and x is expressed amount of adsorbent used in grams.
3.7.1 Langmuir Isotherm Model
According to the Langmuir model, a few sites on the solid surface exhibit the same amount of adsorption energy regardless of the degree of covering, indicating monolayer adsorption. The linearized form of the Langmuir equation is described by the following equation:
The equilibrium dye concentration is expressed as Ce (ppm), the equilibrium dye capacity is expressed as Qe (mg/g), the monolayer adsorption capacity is expressed as Qo (mg/g), and the Langmuir bonding energy coefficient is expressed as bL (mg/l).
The slope and intercept of the linear plot of Ce/qe against Ce can be used to compute the bL and Qo. According to data in Table 5, R2 = 0.96 and bL value range from 0 to 1, demonstrating that MB dye adsorbs well to the adsorbent (ASm3). The outcomes demonstrate that the Langmuir isotherm is the most suitable for MB dye adsorption on the produced adsorbents, indicating that the dye molecules are monolayer-coated on the surface of the adsorbent.
3.7.2 Freundlich Isotherm Model
The applicability of heterogeneous surface energy in the adsorption process is assessed using the Freundlich equation as follows:
where n is the adsorption intensity and Kf is the Freundlich isotherm constants denoting the adsorption capacity. Normal adsorption is indicated by 1/n values below one, whereas collaboration adsorption is indicated by 1/n values over one.
The intercept and slope of the linear plot of log qe against log Ce can be used to compute the Kf and n. The Kf and 1/n values listed in Table 5 show efficient adsorption. The 1/n value nearly ranged from 0 to 1, indicating the variability of the adsorbent surface. Higher values of kf indicate a higher affinity for MB adsorption. R2 values for Langmuir and Freundlich isotherms were given in Table 5. It can be concluded that the Langmuir and Freundlich adsorption isotherms can be explained by the presence of heterogeneous moieties that are uniformly distributed on the surface of the sludge adsorbent.
4 Conclusion
According to the results of the experimental works that were carried out to find the removal efficiency of sludge produced from drinking water treatment plants, after chemical activation, the following conclusions can be stated.
- The generated sludge from the drinking water treatment plant that was activated chemically by (2:1) HCl:H2O2 labelled as (ASm3) was suitable as an adsorbent to remove methylene blue (MB) dye from polluted water recording a high rate of efficiency that reaches 99.9%.
- The optimum parameters for the high removal of MB on the developed adsorbent were MB initial concentration 50 ppm, pH 7, adsorbent dosage 0.25 gm, contact time 90 min, and solution temperature 25 °C.
- The adsorption data of MB dye on ASm3 adsorbent fitted well with Langmuir and Freundlich Isotherm models.
Data Availability
All data generated or analysed during this study are included in this published article.
References
abd EL-Razek, T. M., Elgendy, A. S., & Fouad, M. M. (2016). UTILIZATION OF DRINKING WATER TREATMENT SLUDGE TO PRODUCE ALUMINUM SULFATE. Ain Shams University, Institute of environmental studies and research., V33(1), 87–104.
Ahmad, M., Rehman, W., Khan, M. M., Qureshi, M. T., Gul, A., Haq, S., Ullah, R., Rab, A., & Menaa, F. (2021). Phytogenic fabrication of ZnO and gold decorated ZnO nanoparticles for photocatalytic degradation of Rhodamine B. Journal of Environmental Chemical Engineering, 9, 104725.
Alencar, L. V. T. D., Passos, L. M. S., Soares, C. M. F., Lima, A. S., & Souza, R. L. (2020). Efficiency method for methylene blue recovery using aqueous two-phase systems based on cholinium-ionic liquids. Journal Fashion Technology Textile English, 6, 13–20.
Banat, F., Al-Asheh, S., & Qtaishat, M. (2005). Treatment of waters colored with methylene blue dye by vacuum membrane distillation. Desalination, 174, 87–96.
Bouras, H. D., Isik, Z., Arikan, E. B., Yeddou, A. R., Bouras, N., Chergui, A., Favier, L., Amrane, A., & Dizge, N. (2020). Biosorption characteristics of methylene blue dye by two fungal biomasses. International Journal of Environmental Studies, 78, 365–381.
Cardoso, C. M. M., Zavarize, D. G., Lago, P., & d. (2019). Evaluation adsorbent properties of drinking water treatment plant sludge-based carbos activated by K2CO3/CH3COOH: A low-cost materials for metal ion COOH: Remediation. SN Applied Sciences, 1, 686.
De Carvalho Gomes, S., Zhou, J. L., Li, W., & Long, G. (2019). Progress in manufacture and properties of construction materials incorporating water treatment sludge: A review. Resources Conservation and Recycling, 145, 148–159.
Eslami, H., Sedighi Khavidak, S., Salehi, F., Khosravi, R., Fallahzadeh, R. A., Peirovi, R., & Sadeghi, S. (2017). Biodegradation of methylene blue from aqueous solution by bacteria isolated from contaminated soil. Journal Advance Environment Health Research, 5, 10–15.
Garcia, M. C., Mora, M., Esquivel, D., Foster, J. E., Rodero, A., Jiménez-Sanchidrián, C., & Romero-Salguero, F. J. (2017). Microwave atmospheric pressure plasma jets for wastewater treatment: Degradation of methylene blue as a model dye. Chemosphere, 180, 239–246.
Gurses, A., Dogar, C., Yalcm, M., Acikyildiz, M., Bayrak, R., & Karaca, S. (2006). The adsorption Kinetics of the cationic dye, methylene blue, onto clay. Journal of Hazardous Materials, 131(1–3), 217–228.
Hameed, B. H., & Daud, F. B. M. (2008). Adsorption studies of basic dye on activated carbon derived from agricultural waste: Hevea Brasiliensis Seed Coat. Chemical Engineering Journal, 139, 48–55.
Hussein, Y., & Ahmed. (2007). Adsorption of methylene blue onto treated activated carbon. The Malysian Journal of Analytical Sciences, 11, 400–406.
Ihsan Habib Dakhil (2013). Adsorption of Methylene Blue dye from waste water by spent tea leaves, Journal of Kerbala University, V. 11 No. 3 Scientific.
Janos, P., Coskun, S., Pilarova, V., & Rejnek, J. (2009). Removal of Basic (Methylene Blue) and Acid (egacid orange) dyes from waters by sorption on chemically treated wood shavings. Bioresource Technology, 100, 1450–1453.
Karunanithi, R., Szogi, A.A., Bolan, N., Naidu, R., Loganathan, P., Hunt, P.G., Vanotti, M.B., Saint, C.P., Ok, Y.S., Krishnamoorthy, S., (2015). Phosphorus recovery and reuse from waste streams, vol. 131. In: Advances in Agronomy. Elsevier, pp. 173–250.
Kavitha, K., & Sentamilselvi, M. M. (2015). Removal of Malachite Green from aqueous solution usinglow cost adsorbent. International Journal of Current Research and Academic Review, 3(6), 97–104.
Khan, I., Saeed, K., Zekker, I., Zhang, B., Hendi, A. H., Ahmad, A., Ahmad, S., Zada, N., Ahmad, H., Shah, L. A., Shah, T., & Khan, I. (2022). Review on methylene blue: Its properties, uses, toxicity and photodegradation. Water, 2022(14), 242. https://doi.org/10.3390/w14020242
Kim, S., Yu, M., & Yoon, Y. (2020). Fouling and retention mechanisms of selected cationic and anionic dyes in a Ti3C2Tx MXene-ultrafiltration hybrid system. ACS Applied Materials & Interfaces, 12, 16557–16565.
Kong, G., Pang, J., Tang, Y., Fan, L., Sun, H., Wang, R., Feng, S., Feng, Y., Fan, W., & Kang, W. (2019). Efficient dye nanofiltration of a graphene oxide membrane: Via combination with a covalent organic framework by hot pressing. J. Mater. Chem. A, 7, 24301–24310.
Lau, Y. Y., Wong, Y. S., Teng, T. T., Morad, N., Rafatullah, M., & Ong, S. A. (2015). Degradation of cationic and anionic dyes in coagulationf locculation process using bi-functionalized silica hybrid with aluminum-ferric as auxiliary agent. RSC Advances, 5, 34206–34215.
Li, H., Liu, L., Cui, J., Cui, J., Wang, F., & Zhang, F. (2020). High-efficiency adsorption and regeneration of methylene blue and aniline onto activated carbon from waste edible fungus residue and its possible mechanism. RSC Advances, 10, 14262–14273.
Lombi, E., Stevens, D. P., & McLaughlin, M. J. (2010). Effect of water treatment residuals on soil phosphorus, copper and aluminium availability and toxicity. Environmental Pollution, 158(6), 2110–2116.
Mahmoud, M. S., Farah, J. Y., & Farrag, T. E. (2013). Enhanced removal of Methylene Blue by electrocoagulation using iron electrodes. Egyptian Journal of Petroleum, 22, 211–216.
Makris, K. C., Sarkar, D., & Datta, R. (2006a). Aluminum-based drinking-water treatment residuals: A novel sorbent for perchlorate removal. Environmental Pollution, 140(1), 9–12.
Makris, K. C., Sarkar, D., & Datta, R. (2006b). Aluminum-based drinking-water treatment residuals: A novel sorbent for perchlorate removal. Environmental Pollution, 140, 9–12.
Mall, I. D., Srivastava, V. C., Agarwal, N. K., & Mishra, I. M. (2005). Adsorptive removal of malachite green dye from aqueous solution by bagasse fly ash and activated carbon-kinetic study and equilibrium isotherm analyses”. Colloids and Surfaces, a: Physicochemical and Engineering Aspects, 264, 17–28.
Mane, V. S., & Babu, P. V. (2011). Studies on the adsorption of brilliant green dye from aqueous solution onto low-cost NaOH treated sawdust”. Desalination, 273, 321–329.
Minh Duc Nguyen, A., Michael Thomas, B., Aravind Surapaneni, C. D., Moona, E. M., & Milne, N. A. A. (2022). Beneficial reuse of water treatment sludge in the context of circular economy. Environmental Technology & Innovation, 28, 102651.
Nageeb, R. M., El-Daim El Taher, M. A., & Fadlalla, S. M. M. (2016). Adsorption of methylene blue using modified adsorbents from drinking water treatment sludge. Water Science & Technology, 74(8), 1885–1889.
Poormand Hamid, Mostafa Leili, Marzieh Khazaei (2017) Adsorption of methylene blue from aqueous solutions using water treatment sludge modified with sodium alginate as a low-cost adsorbent Water Science & Technology 75(2). https://doi.org/10.2166/wst.2016.510
Rashed, M. N., El Taher, M. A., & Fadlalla, S. M. M. (2020). Synthesis and photocatlytic application of drinking water treatment sludge @ TiO2 composite for degradation of methylene blue Dye. Pollution, 6(4), 785–799.
Rashed, M. N., El Taher, M.-D., & Fadlalla, S. M. M. (2022). Photocatalytic degradation of Rhodamine -B dye using composite prepared from drinking water treatment sludge and nano TiO2. Environmental Quality Management, 31(3), 175–185.
Reham, R., & Mahmud, T. (2013). Utilization of Ricinus communis stem powder for sorptive eradication of methylene blue dye from aqueous media in ecofriendly way. IWTC 2013 Seventeenth International Water Technology Conference. Istanbul, Turkey, 5–7.
Ren, B., Lyczko, N., Zhao, Y., & Nzihou, A. (2020). Alum sludge as an efficient sorbent for hydrogen sulfide removal: Experimental, mechanisms and modeling studies. Chemosphere, 248, 126010.
Saiful Azhar, A., Ghaniey Liew, D., Suhardy, K., Farizul Hafi, M. D., & Hatim, I. (2005). Dye removal from aqueous solution by using adsorption on treated sugarcane Bagasse, American Journal of applied Sciences 2(11). ISSN, 1499–1503, 1546–9239.
Sirisha, D., Mukkanti, K., & Gandhi, N. (2012). Adsorption studies on alum sludge. Advances in Applied Science Research, 3(5), 3362–3366.
Sujana, M. G., Thakur, R. S., & Rao, S. B. (1998). Removal of fluoride from aqueous solution by using alum sludge. Journal of Colloid and Interface Science, 206(1), 94–101.
Tekile, A., Kim, I., & Kim, J. (2015). Mini-review on river eutrophication and bottom improvement techniques, with special emphasis on the Nakdong River. Journal of Environmental Sciences, 30, 113–121.
Tir, M., Moulai-Mostefa, N., & Nedjhioui, M. (2015). Optimizing decolorization of methylene blue dye by electrocoagulation using Taguchi approach. Desalination and Water Treatment, 55, 2705–2710.
Valério Filho, A., Xavaré Kulman, R., Vaz Tholozan, L., Felkl de Almeida, A. R., & Silveira da Rosa, G. (2020). Preparation and characterization of activated carbon obtained from water treatment plant sludge for removal of cationic dye from wastewater. Processes, 8, 1549.
Wang, Z., Gao, M., Li, X., Ning, J., Zhou, Z., & Li, G. (2020). Efficient adsorption of methylene blue from aqueous solution by graphene oxide modified persimmon tannins. Materials Science and Engineering C, 108, 110196.
Yusuff, S. M., Khim, O. K., Md Zin Man Yunus, W., Fitrianto, A., Ahmad, M., Ibrahim, N., Siswoyo, E., Qoniah, I., Lestari, P., Fajri, J. A., Sani, R. A., Sari, D. G., & Boving, T. (2019). Development of a floating adsorbent for cadmium derived from modified drinking water treatment plant sludge. Environment Technology Innovations, 14, 100312.
Zheng, L., Su, Y., Wang, L., & Jiang, Z. (2009). Adsorption and recovery of methylene blue from aqueous solution through ultrafiltration technique. Purification Technology, 68, 244–249.
Zhong, F., Wang, P., He, Y., Chen, C., Li, H., Yu, H., & Chen, J. (2019). Preparation of stable and superior flux GO/LDH/PDA-based nanofiltration membranes through electrostatic self-assembly for dye purification. Polymers for Advanced Technologies, 30, 1644–1655.
Funding
Open access funding provided by The Science, Technology & Innovation Funding Authority (STDF) in cooperation with The Egyptian Knowledge Bank (EKB).
Author information
Authors and Affiliations
Corresponding author
Ethics declarations
Conflict of Interest
The authors declare no competing interests.
Additional information
Publisher's Note
Springer Nature remains neutral with regard to jurisdictional claims in published maps and institutional affiliations.
Rights and permissions
Open Access This article is licensed under a Creative Commons Attribution 4.0 International License, which permits use, sharing, adaptation, distribution and reproduction in any medium or format, as long as you give appropriate credit to the original author(s) and the source, provide a link to the Creative Commons licence, and indicate if changes were made. The images or other third party material in this article are included in the article's Creative Commons licence, unless indicated otherwise in a credit line to the material. If material is not included in the article's Creative Commons licence and your intended use is not permitted by statutory regulation or exceeds the permitted use, you will need to obtain permission directly from the copyright holder. To view a copy of this licence, visit http://creativecommons.org/licenses/by/4.0/.
About this article
Cite this article
Soliman, M.F., Rashed, M.N. & Ahmd, A.A. Chemical Activation of Sludge from Drinking Water Treatment Plant for Adsorption of Methylene Blue Dye. Water Air Soil Pollut 234, 228 (2023). https://doi.org/10.1007/s11270-023-06216-9
Received:
Accepted:
Published:
DOI: https://doi.org/10.1007/s11270-023-06216-9