Abstract
Microbial-derived metabolites are the intermediate or end products of bacterial digestion. They are one of the most important molecules for the gut to connect with the brain. Depending on the levels of specific metabolites produced in the host, it can exert beneficial or detrimental effects on the brain and have been linked to several neurodegenerative and neuropsychiatric disorders. However, the underlying mechanisms remain largely unexplored. Insight into these mechanisms could reveal new pathways or targets, resulting in novel treatment approaches targeting neurodegenerative diseases. We have reviewed selected metabolites, including short-chain fatty acids, aromatic amino acids, trimethylamine-N-oxide, urolithin A, anthocyanins, equols, imidazole, and propionate to highlight their mechanism of action, underlying role in maintaining intestinal homeostasis and regulating neuro-immunoendocrine function. Further discussed on how altered metabolite levels can influence the gut–brain axis could lead to new prevention strategies or novel treatment approaches to neural disorders.
Similar content being viewed by others
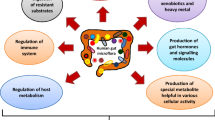
Avoid common mistakes on your manuscript.
Introduction
The gastrointestinal tract (GIT) hosts more than 100 trillion microbes that together harbour more than 3 million genes [1]; however, the predominant bacteria consist mainly of phyla Firmicutes, Bacteroidetes, Actinobacteria, Proteobacteria, and Verrucomicrobia [2]. The inhabitant bacteria in the GIT carry out an assortment of activities that benefit the host, including the digestion of vitamins, and metabolites (bile acids, amino acids, lipids), regulating pH change and peptide production, and influencing cell signalling pathways. Some of these metabolites are commonly altered due to environmental factors and have further been correlated with neurodegenerative symptoms [3].
Though the microbiota composition is shaped before birth, factors such as the birthing method, feeding method, age, diet, geographic location, genetics, and physical activity determine the core microbiome of an individual [4]. The human microbiome project has shown that human microbiota is more similar across individuals than across body sites and is unique to specific organs [5]. Further, the gut microbiota composition can be altered by factors like pH, bacterial load, oxygen, medicines like antibiotics, and supplements like prebiotics and probiotics [6]. Deviations from normal gut microbiome composition can lead to dysbiosis that can prove detrimental to an individual’s health. Further, it can also act as a biomarker for exposure or treatment response [7]. The microbiota gut-brain axis (MGBA) is a term used to describe the link between the gut and the brain involving the interplay of several systems, mainly the autonomic, central, and enteric nervous systems and the hypothalamic-pituitary axis. Several mechanisms are implicated in MGBA signalling, including the involvement of the vagus nerve, the immune system, vitamins, and bacterial metabolites.
Gut metabolites are the intermediates or end products of bacterial metabolic processes. Some important metabolites produced include aromatic amino acids and short-chain fatty acids (SCFAs) derived from dietary sources such as fibres and fruits; endogenous metabolites derived from bile acids and cholesterol. Their primary signalling pathway is through the vagal and spinal nerves of the autonomic nervous system (ANS). These metabolites also can enter the bloodstream; several of them can permeate the blood–brain barrier (BBB) and influence regulatory mechanisms in the central nervous system (CNS) [8]. The ANS also controls gut permeability, motility, and immuno-modulatory mechanisms, determining the bacterial composition and the metabolites produced. These metabolites have been linked to neurodegenerative, neuroinflammatory, and neuropsychiatric disorders such as Alzheimer’s disease (AD), Parkinson’s disease (PD), and autism spectrum disorders (ASD) [9,10,11]. They have been found to alleviate/exacerbate various neuronal symptoms. Further insight into their underlying mechanisms could shed light on the neuromodulatory pathways and lead to practical novel therapeutic approaches. This review briefly summarizes the mechanisms of various metabolites like SCFAs, aromatic amino acids, and other less explored metabolites and their effects on brain health and neural activities.
Gut-associated neurological disorders
Neurological disorders are characterized by a progressive decrease in nervous system function. Gut microbiome changes in humans have been reported to be one of the many causes of various- psychological and neurological conditions [12]. Using specific pathogen-free (SPF) or germ-free (GF) murine models, researchers hope to unravel the molecular pathways from the gut to the brain [13, 14]. Cross-sectional studies have found that the bacterial taxa Escherichia and Shigella, which are associated with causing inflammation, are elevated in faecal samples from Alzheimer’s patients compared to healthy people [15]. Additionally, another study showed AD progression might not affect the brain directly but through amyloid deposition in the APPswe/PS1∆E9 transgenic mice intestine, where they found altered gut microbiome, lowered SCFAs, and altered metabolic pathways [16]. It has been reported that alteration in the composition of bacteria-producing metabolites may initiate and sustain the pathogenic processes of neurodegeneration [17]. So, dysbiosis of the gut contributes to neuroinflammation and injury, which can result in neurodegeneration, key factors associated with AD. Similarly, Parkinson’s disease (PD) is another motor-impaired disorder. Preclinical research suggests that gut microbiota can enhance the aggregation of alpha-synuclein, hamper the elimination process [18], and via the vagus nerve, these proteins can reach from the gut to the brain [19, 20]. The alpha-synuclein can aggregate in various neurons to form Lewy bodies and Lewy neurites, leading to disease pathology. In another study, researchers orally introduced bacterial-derived SCFAs to alpha-synuclein overexpressing mice to test the hypothesis that SCFAs induced alpha-synuclein production in the brain and showed a positive association between SCFAs and PD [21].
In addition, microbial metabolites have been associated with neurological disorders like multiple sclerosis (MS), dementia, and epilepsy [22]. A study comparing the microbiome of 71 untreated MS patients to healthy controls reported a significant increase in Akkermansia muciniphila and Acinetobacter calcoaceticus, which are associated with MS [23]. Further, the transplantation of gut microbiota of MS patients into germ-free mice indicates the potentially causal role of gut microbiota in MS [20, 21]. These findings suggest that gut microbiota could be linked to the increased susceptibility of MS in humans.
Microbe-derived metabolites are linked to the MGBA
The gut microbiota produces a variety of metabolites that have a diverse array of functions and play a significant role in signalling pathways in the MGBA (Fig. 1). Among these, metabolites like short-chain fatty acids, aromatic amino acids, and trimethylamine-N-oxide, which could influence brain function, have gained wide attention (Table 1). Gut microbiota-derived metabolites can enter host circulation through the gut barrier and can be beneficial or toxic. However, the effects of metabolites are influenced by the epithelial barrier intactness, dietary components like fibres and fruits, geographic location, and ethnicity to which the host belongs [24].
Gut bacteria-derived metabolites and their neuronal effects. Both extrinsic and intrinsic factors can cause dysbiosis of the gut, characterised by increased gut permeability or “leaky gut” and shifts in bacterial taxonomy groups, leading to altered levels of various metabolites. These metabolites can enter the bloodstream and get transported through the ANS to the CNS to alter neuronal activities and modulate the expression of neurotransmitters.
Amino acids also can undergo microbial fermentation to yield ammonia, phenol, and indole. Signalling molecules derived from tryptophan, like serotonin (5-HT), is responsible for regulating mood, behaviour, sleep, and appetite [25]. 5-HT binds to aryl hydrocarbon receptors (AhR) on astrocytes and can activate microglia modulating neuroinflammation. Their role in depressive disorders and anxiety is being explored [26, 27]. Indole is a tryptophan derivative with neuroactive properties and is reported to cross the blood–brain barrier to regulate the levels in the brain [28]. Neurotransmitters like dopamine are produced from bacteria like Escherichia, which metabolizes phenylalanine, an aromatic amino acid. Another metabolite, choline, is essential for neurotransmission and methylation and is metabolized to Trimethylamine N-oxide (TMAO), which is involved in the MGBA and can be detected in the CNS [29].
Microglial activation can be influenced by the circulation of microbe-derived neurotransmitters such as acetylcholine (Lactobacillus), gamma-aminobutyric acid (GABA) (Bifidobacteria and Lactobacillus), and serotonin (Enterococcus and Streptococcus). According to studies, the gut produces 90% of the serotonin required to regulate mood, behavior, sleep, and other processes in the CNS and GI tract. Serotonin binding to 5-HT receptors on microglia causes the release of cytokine-carrying exosomes, offering yet another pathway for gut-induced neuroinflammation control [30].
The effects of SCFAs have recently been extended to microglia. The immature genetic and morphological phenotype of microglia in GF mice was recovered by supplementing drinking water with three major SCFAs (acetic acid, propionic acid, and butyric acid). Microglial dysfunction is assumed to play a role in the progression of neurological disorders, including ASD and PD. Once in the bloodstream, these chemicals trigger a pro-inflammatory immune response mediated by peripheral T cells and macrophages, compromising the BBB’s integrity [31]. The microbial-derived metabolites are also crucial for neuronal homeostasis and controlling inflammation [32]. Rothhammer et al. [33] highlighted the role of metabolites in regulating the microglia and astrocytes in the CNS, which plays a role in neuroinflammation and neurodegeneration. Increased microglial activation and generation of pro-inflammatory cytokines in the brain may be due to increased circulation of BBB-permeable pro-inflammatory cytokines.
Alteration of microbial-derived metabolites by oral administration of Bacteroides fragilis to the maternal immune activation (MIA) C57BL/6N mouse model that exhibited symptoms relevant to ASD, ameliorated communicative defects and sensorimotor defects; decreased anxiety-like behaviour [22]. These observations indicate that the microbial-derived metabolites influence diverse signalling pathways that control brain functions (Fig. 2). They have been considered promising candidates for ameliorating neurodegenerative disorders. However, mechanisms of action for most of these metabolites remain to be investigated.
Mechanism of action of gut microbiota-derived metabolites on the CNS. The gut microbiota composition varies across the intestinal lumen and depends on several factors, including dietary sources. The illustration depicts a few relevant metabolites like isoflavones, SCFAs, and AAAs that serve as precursors for secondary metabolites like equols, propionate, butyrate, and neurotransmitters like epinephrine and dopamine. These metabolites participate in MGBA signaling, binding to receptors or agonists and crossing the epithelial barrier to exert their effects in the CNS. Here, they influence several neural functions that can be detrimental or beneficial to the host (↑ increase, ↓decrease). SCFAs short chain fatty acids, AAA aromatic amino acids, FFA2/3 Free fatty acid receptors 2 and 3, BA butyrate, PA propionate, Epi epinephrine, Dop dopamine, Nor norepinephrine, Phe phenylalanine, Tyr tyrosine, Trp tryptophan, ArH aryl hydrocarbon, BBB blood brain barrier
Short chain fatty acids (SCFAs)
SCFAs are gut microbiota-derived metabolites produced by fermentation of non-digestible carbohydrates. The major SCFAs produced in comparatively large ratios are acetate, propionate, and butyrate, whereas other SCFAs like isovalerate and isobutyrate are produced in trace amounts [34, 35]. SCFAs play a significant role in maintaining the intestinal barrier, regulating inflammatory response, and mucus production [36]. SCFAs are also natural ligands for receptors like free fatty acid receptors 2 and 3 (FFA2/FFA3) attached to immune cells and enteroendocrine cells [37], allowing them to modulate the levels of several pro-inflammatory cytokines that can induce neuroinflammation. Further, SCFAs, when coupled with G-protein receptors, can modulate the production of pro-inflammatory cytokines, activate inflammasome pathways like the NLRP3 pathway, and cause inflammation [24]. SCFAs are also one of the primary metabolites implicated in the MGBA, and several studies have highlighted their importance in influencing several other body organs, including the brain [38].
Clear evidence of uptake of SCFAs by the brain was demonstrated by Oldendorf et al. [39], where SCFAs were injected into the common carotid artery of Wistar rats, and all the three significant SCFAs were detected in the Cerebrospinal fluid (CSF) in varying ratios. Supplementation with SCFAs has altered several functions related to the brain. Li et al. [40] demonstrated that SCFAs prevented neuroinflammation in fructose-fed mice by reducing the pro-inflammatory cytokines like IL-1β, TNF-α, IL-6 mRNA levels, and microglial activation. One of the major systems involved in the GBA- the Hypothalamic Pituitary Axis, a core stress axis, is also affected by SCFAs. Various studies have correlated altered gut microbiota composition with varying stress levels. One study demonstrated that when mice were administered a mixture of sodium acetate, propionate, and sodium butyrate, they exhibited altered anhedonia and increased stress-responsiveness [41].
Due to the increasing evidence supporting SCFA’s role in affecting neural functions, they are being investigated for their role in neurodegenerative disorders. Marrizzoni et al. [42] reported a link between SCFAs (acetate and valerate) and endothelial damage, which correlates to the regulation of the BBB and, in turn, contributes to AD pathogenesis. A critical activity of SCFAs is their ability to act as histone deacetylase inhibitors (HDACi). They can alter histone acetylation during memory formation. Considering that SCFAs can act as HDACi, it has been hypothesized that they can be used as a therapeutic in treating AD to correct aberrant histone acetylation, which is associated with the pathogenesis of AD [42].
Further investigation could reveal their more profound role in learning and memory. Evidence shows that SCFAs are altered in individuals with ASD. A study on GI disorders and pathogenesis of ASD reported decreased levels of faecal acetic acid, butyrate, and propionic acid comparatively increased levels of valeric acids in ASD subjects [43]. In a study conducted by Zeng et al. [44], an analysis of faecal samples of Amyotrophic Lateral Sclerosis (ALS) patients revealed an increase in butyrate levels to reduce the accumulation of abnormal proteins. Similarly, in a G93A transgenic mice model of ALS, animals fed with butyrate were shown to have improved gut homeostasis and a longer life span [45]. However, the causal role between ALS and metabolites is still not well established. Further investigations need to be carried out to understand whether the alteration in levels of various gut metabolites is the cause or affects ALS.
Chen et al. [46] demonstrated that supplementing butyric acid to rat middle cerebral artery occlusion models reduced neurological impairment, lipid levels, and risk of thrombosis. Butyric acid treatment has been shown to increase the microbial alpha diversity with an increase in Lactobacillus, which has been reported to have beneficial effects like preventing neuronal cell death, reducing oxidative stress, and repairing abnormal neurobehavior [46]. Supplementing sodium butyrate to rats with ischemic brain showed upregulated Brain-Derived Neurotrophic Factor (BDNF) levels, increased neurogenesis, and neural proliferation [47] played a role in the memory process [48].
In another study, Erny et al. [49] demonstrated that the microglia of GF mice showed defects that were partially restored into a mature phenotype when recolonized with complex gut microbiota or fed with SCFA (a mixture of acetate, butyrate, and propionate). They can also affect brain neurochemistry by regulating the expression of 5-HT enzymes, which are serotonin precursors. Increased SCFA levels were also associated with increased growth of human progenitor cells, implying that they could influence early neural development. Further, physiologically relevant levels of acetate, propionate, and butyrate enhanced the growth rate of cells and induced mitosis [50]. Studies have also demonstrated the ability of SCFAs to affect the sleep and appetite signals of the brain by modulating neurons like orexigenic neurons and receptors such as the ghrelin receptor [51]. This mechanism is revealed to be brought about by coupling SCFAs to FFA2/3 receptors. Overall, SCFAs tend to regulate GI function, circadian rhythm, and other neuroimmune functions; however, the studies mentioned above have indicated a role in lowering neuroinflammation, regulating the blood–brain barrier, and, most importantly, ameliorating memory loss associated with neuroinflammatory disorders.
Aromatic amino acid (AAA) metabolites
All three aromatic amino acids—phenylalanine, tyrosine, and tryptophan, play a significant role in the MGBA and are a product of gut microbial metabolism. These three amino acids serve as precursors to several secondary metabolites, some of which act as neurotransmitters and play a role in brain health [52]. For example, phenylalanine is the starting product that produces dopamine, norepinephrine, and epinephrine, whereas tryptophan is the precursor to 5-HT and serotonin, vitamin B3, and redox co-factors like NAD(P)+. Even kynurenine pathway metabolites like indole and its derivatives are produced from the AAA metabolism [53]. Tryptophan metabolite- kynurenine was shown to reduce the activity of Natural Killer (NK) and dendritic cells in the CNS, and accumulation of this metabolite in the brain is associated with depression and schizophrenia [50]. Phenylalanine affects neurotransmission by producing amino acids like glutamate and aspartate, which act as excitatory neurotransmitters [53]. Another amino acid, glycine, also made from phenylalanine, is an inhibitory neurotransmitter [54]. Serotonin is a neurotransmitter that regulates gastrointestinal secretion motility and pain perception, regulates mood and cognition behaviour in the brain, and is produced by the gut mucosal enterochromaffin cells [25]. These studies suggest that AAAs should be investigated further for their role in influencing neural disorders.
Tryptophan metabolites
The gut microbiota plays an essential role in tryptophan metabolism, a precursor to serotonin production. More than half of serotonin is produced in the gut and is responsible for activating several receptors on the enteric neurons and immune cells. Serotonin also binds to 5-HT receptors in the microglia and mediates neuroinflammation in the CNS. Furthermore, tryptophan gives rise to bacterial metabolites that can control CNS inflammation through receptors like the AhR [55]. Tryptophan metabolites have been shown to modulate metalloproteinases that control the degradation of amyloid-beta (Aβ)peptides through AhR in the CNS. A recent review indicated that 5-hydroxy indole acetic acid and kynurenic acid could prevent the formation of Aβ plaques [10]. Tryptophan levels can also reduce anxiety-like behaviour. For example, when restoring microbiota to germ-free mice, they exhibited reduced anxiety levels, 5-HT, and 5-Hydroxindoleacetic acid levels compared to conventionally colonized mice [25]. In mice, a tryptophan-rich diet slows brain aging by decreasing oxidative stress and inflammation by regulating AMP-activated protein kinase (AMPK) and Nf-kB pathways [56]. In a high level of cognitive strain, the injection of 5-HT2A/2C or 5-HT4 receptor agonists or 5-HT1A, 5HT3, and 5-HT1B receptor antagonists avoids memory loss and improves learning [57]. This indicates the beneficial effects of tryptophan in modulating neuroinflammation and neurogenesis.
Indole, another metabolite produced from precursor tryptophan by an enzyme tryptophanase A (tnA), is said to induce depression and impact normal emotional behavior. Treating rats with indole led to the accumulation of its oxidized derivatives in the brain, negatively affecting the rats by reducing motor activity and inducing a higher anxiety-like behaviour [58]. P-cresol, derived from tyrosine, plays a major role in several neurodegenerative disorders like ASD. In a BTBR ASD mice model, increased doses of P-cresol were said to worsen certain symptoms of ASD and activate dopamine levels in specific brain regions like the amygdala [59]. Another study reported how p-cresol inhibited the formation of oligodendrocytes, which might hamper the formation of myelinated neurons in the mice CNS [60]. As the studies reported, microbial-derived amino acids and tryptophan metabolites tend to induce oxidative stress and inflammation, reducing motor activity and inducing a higher anxiety-like behavior. Studies showed that 5-hydroxy indole acetic acid and kynurenic acid could prevent the formation of Aβ-peptide plaques [10]. Recent reports suggest that P-cresol sulfate and 4-ethyl phenyl sulfate, two microbiota-derived host metabolites, are elevated in ASD patients and animal models such as BTBR and MIA mice [59]. However, further research is required to understand the link between these metabolites and ASD. Overall, the studies indicate the potential beneficial effects of tryptophan in modulating neuroinflammation and neurogenesis.
Trimethylamine N-oxide (TMAO)
TMAO is another microbe-derived molecule produced by several bacterial species from dietary constituents such as choline and L-choline. They can be detected in the CSF, implying that it is involved in the CNS and has been investigated for its pathophysiological role in neurodegenerative disorders [29]. TMAO has been implicated in numeral cerebrovascular diseases like atherosclerosis [61], a known risk factor for dementia. It also induces CD68 expression, which is a marker associated with dementia. It causes neuronal aging, disrupts mitochondrial functions, and increases oxidative stress.
A study conducted by Vogt et al. [29] to investigate the role of TMAO in AD showed that elevated levels of TMAO were detected in CSF collected from individuals with clinical AD and individuals with mild cognitive impairment (MCI) when compared to cognitively unimpaired individuals. The elevated levels were also linked to phosphorylated tau and Aβ. Further studies to understand the specific role of TMAO in neurodegenerative diseases like Parkinson’s could shed further light on the pathophysiology of the disease. TMAO can induce brain aging and cognitive dysfunction. The link between TMAO and brain aging was analyzed by Li et al. [62], who showed that plasma levels of TMAO were increased in senescence-accelerated prone mouse strain 8 (SAMP8) compared to control mice. These mice also exhibited cognitive dysfunction with more senescent cells in the hippocampal CA3 region. The observed neuronal damage and reduced synaptic plasticity were associated with increased superoxide production and impaired mitochondria. These changes mainly involved mammalian target of rapamycin (mTOR) signaling. This activity relates to spatial learning, object recognition, and memory by regulating receptors like N-Methyl D-Aspartate receptor subunit 1.
In an AD (3xTg-AD) model, increased levels of TMAO were associated with altered presynaptic and reduced postsynaptic receptor expression, which was brought about by the stress signaling pathway [63]. Brunt et al. [64] provided evidence linking TMAO levels, neuroinflammation, and cognitive decline. The study comparing mice of different ages (27 months vs. 6 months) showed that with aging, TMAO concentrations increased, which correlated to higher pro-inflammatory cytokines and astrocyte activation. Additionally, when 6-months old mice were supplemented with TMAO, they performed poorly in the novel object recognition test and exhibited astrocyte activation. A reduction of TMAO levels in APP/PS1 mice led to a decrease in cognitive deterioration, Aβ and beta-related enzymes like beta-secretase in the hippocampus, and hippocampal neuroinflammation [65]. Overall, studies indicate that TMAO is linked to the development of age-related cognitive deterioration and is associated with AD, diabetes mellitus, and cardiovascular illnesses. However,the mechanisms leading to observed effects remain unknown.
Other metabolites
Urolithin A is a significant gut-derived metabolite derived from ellagic acid, whose sources include pomegranates, berries, and nuts. In-vivo and in-vitro studies have demonstrated the antioxidant and anti-inflammatory effects of these metabolites; however, the underlying molecular mechanisms still need to be elaborated upon. Gong et al. [66] investigated the effects of urolithin A in alleviating AD symptoms and showed improved cognitive functions, inhibited neural apoptosis, induced neurogenesis, and decreased the pro-inflammatory cytokines IL-1β and TNF-α in the mice cortex and hippocampus. Thus, Urolithin A is a promising therapeutic target for improving the pathophysiology of AD.
Anthocyanins are microbial-derived metabolites whose dietary sources include berries, grapes, plums, and other foods containing high natural colorants. They prevent oxidative stress, have neuroprotective effects, reduce neuroinflammation and regulate cell signaling pathways [67]. Ali et al. [68] described the role of Korean black bean anthocyanin in reducing neural apoptosis in an APP/PS1 transgenic mouse model of AD. Several Bifidobacterium species and Lactobacillus produce metabolites like GABA and inhibitory neurotransmitters.
Equols (EQ) are gut-derived metabolites obtained by metabolizing isoflavones. They protect microglia against oxidative stress, prevent neural apoptosis, and induce neural generation. They have also been extensively studied for their bioactive role in selected bone and cardiovascular disorders. Johnson et al. [69] demonstrated that EQs could permeate the blood–brain barrier and reduce the production of pro-inflammatory cytokines, preventing neuroinflammation. In another study, SOD1-Tg mice were administered with Akkermansia muciniphila, which resulted in the accumulation of nicotinamide in the CNS, ameliorating several symptoms of ALS, improved motor symptoms, and altered gene expression levels in the spinal cord [70].
The microbial metabolite imidazole propionate has been found to disrupt insulin signaling and lead to type II diabetes, which can negatively affect cognitive functions of the brain and has been known to be a major risk factor in the development of Alzheimer’s [71, 72]. Other metabolites such as dihydrosphingosine, phytosphingosine, inosine, and hypoxanthine have also alleviated AD symptoms. When Xanthoceraside, a compound proven to have anti-Alzheimer’s activity, was administered, altered levels of these metabolites accompanied by a change in the gut bacterial taxa were reported, thus providing new potential treatment approaches [73]. Polyphenol metabolites like gallic acid derivatives have also demonstrated neuroprotective targets [74]. These findings provide further impetus to look into novel pathways and therapeutic approaches for treating numerous neurodegenerative diseases.
Conclusion
Gut-derived metabolites play a significant role in various functions of the host. They modulate the immune system by influencing the levels of several pro-inflammatory cytokines; they can exert neuro-inhibitory and neuro-excitatory effects; they possess anti-oxidant properties and influence epigenetic mechanisms. Factors such as the permeability of the gut and mucus secretion levels determine the type of bacteria present and the metabolites they produce, so when dysbiosis occurs, these factors are altered, which in turn change the levels of metabolites produced by the bacteria, thereby exerting beneficial or detrimental effects on the host. For example, supplementing metabolites like SCFAs has been shown to alleviate symptoms related to neurodegenerative disorders, such as inducing neurogenesis, preventing neural death, and reducing oxidative stress. The exact underlying mechanisms of most of these metabolites are not understood; however, evidence of their effects on the brain has been reported in multiple studies. Approaches such as modulating the levels of metabolites either by controlling dietary sources or supplementing them externally have been shown to demonstrate significant changes, be it ameliorating or exacerbating symptoms, making them promising targets for novel treatment approaches.
Data availability
Not applicable.
References
Rinninella E, Raoul P, Cintoni M et al (2019) What is the healthy gut microbiota composition? a changing ecosystem across age, environment, diet, and diseases. Microorganisms 7:14. https://doi.org/10.3390/microorganisms7010014
Zhang S, Chen D-C (2019) Facing a new challenge. Chin Med J (Engl) 132:1135–1138. https://doi.org/10.1097/CM9.0000000000000245
Bai J, Barandouzi ZA, Rowcliffe C et al (2021) Gut microbiome and its associations with acute and chronic gastrointestinal toxicities in cancer patients with pelvic radiation therapy: a systematic review. Front Oncol 11:5237. https://doi.org/10.3389/fonc.2021.745262
Cresci GAM, Izzo K (2019) Gut Microbiome. Adult Short Bowel Syndrome. Elsevier, Amsterdam, pp 45–54
Human Microbiome Project Consortium (2012) Structure, function and diversity of the healthy human microbiome. Nature 486:207–214. https://doi.org/10.1038/nature11234
Kennedy MS, Chang EB (2020) The microbiome: composition and locations. Prog Mol Biol Transl Sci 176:1–42. https://doi.org/10.1016/bs.pmbts.2020.08.013
Joseph NT, Shankar SR, Narasimhamurthy RK et al (2020) Bi-directional interactions between microbiota and ionizing radiation in head and neck and pelvic radiotherapy – clinical relevance. Int J Radiat Biol 96:961–971. https://doi.org/10.1080/09553002.2020.1770361
Braniste V, Al-Asmakh M, Kowal C et al (2014) The gut microbiota influences blood-brain barrier permeability in mice. Sci Transl Med 6:263. https://doi.org/10.1126/scitranslmed.3009759
Singh S, senRai Birla, SNH et al (2020) NF-κB-mediated neuroinflammation in Parkinson’s disease and potential therapeutic effect of polyphenols. Neurotox Res 37:491–507. https://doi.org/10.1007/s12640-019-00147-2
Maitre M, Klein C, Patte-Mensah C, Mensah-Nyagan A-G (2020) Tryptophan metabolites modify brain Aβ peptide degradation: a role in Alzheimer’s disease? Prog Neurobiol 190:101800. https://doi.org/10.1016/j.pneurobio.2020.101800
Pulikkan J, Mazumder A, Grace T (2019) Role of the gut microbiome in autism spectrum disorders. Adv Exp Med Biol 1118:253–269. https://doi.org/10.1007/978-3-030-05542-4-13
Cenit MC, Sanz Y, Codoñer-Franch P (2017) Influence of gut microbiota on neuropsychiatric disorders. World J Gastroenterol 23:5486. https://doi.org/10.3748/wjg.v23.i30.5486
Zhang C, Franklin CL, Ericsson AC (2021) Consideration of gut microbiome in murine models of diseases. Microorganisms 9:1062. https://doi.org/10.3390/microorganisms9051062
Park JC, Im S-H (2020) Of men in mice: the development and application of a humanized gnotobiotic mouse model for microbiome therapeutics. Exp Mol Med 52:1383–1396. https://doi.org/10.1038/s12276-020-0473-2
Cryan JF, O’Riordan KJ, Sandhu K et al (2020) The gut microbiome in neurological disorders. Lancet Neurol 19:179–194. https://doi.org/10.1016/S1474-4422(19)30356-4
Zhang L, Wang Y, Xiayu X et al (2017) Altered gut microbiota in a mouse model of Alzheimer’s disease. J Alzheimer’s Dis 60:1241–1257. https://doi.org/10.3233/JAD-170020
Friedland RP (2015) Mechanisms of molecular mimicry involving the microbiota in neurodegeneration. J Alzheimers Dis 45:349–362. https://doi.org/10.3233/JAD-142841
Chen SG, Stribinskis V, Rane MJ et al (2016) Exposure to the functional bacterial amyloid protein curli enhances alpha-synuclein aggregation in aged fischer 344 rats and caenorhabditis elegans. Sci Rep 6:34477. https://doi.org/10.1038/srep34477
Kim S, Kwon S-H, Kam T-I et al (2019) Transneuronal propagation of pathologic α-synuclein from the gut to the brain models Parkinson’s disease. Neuron 103:627-641.e7. https://doi.org/10.1016/j.neuron.2019.05.035
Holmqvist S, Chutna O, Bousset L et al (2014) Direct evidence of Parkinson pathology spread from the gastrointestinal tract to the brain in rats. Acta Neuropathol 128:805–820. https://doi.org/10.1007/s00401-014-1343-6
Sampson TR, Debelius JW, Thron T et al (2016) Gut microbiota regulate motor deficits and neuroinflammation in a model of Parkinson’s disease. Cell 167:1469-1480.e12. https://doi.org/10.1016/j.cell.2016.11.018
Cryan JF, O’Riordan KJ, Cowan CSM et al (2019) The microbiota-gut-brain axis. Physiol Rev 99:1877–2013. https://doi.org/10.1152/physrev.00018.2018
Cekanaviciute E, Yoo BB, Runia TF et al (2017) Gut bacteria from multiple sclerosis patients modulate human T cells and exacerbate symptoms in mouse models. Proc Natl Acad Sci 114:10713–10718. https://doi.org/10.1073/pnas.1711235114
Mohajeri MH, la Fata G, Steinert RE, Weber P (2018) Relationship between the gut microbiome and brain function. Nutr Rev 76:481–496. https://doi.org/10.1093/nutrit/nuy009
Clarke G, Grenham S, Scully P et al (2013) The microbiome-gut-brain axis during early life regulates the hippocampal serotonergic system in a sex-dependent manner. Mol Psychiatry 18:666–673. https://doi.org/10.1038/mp.2012.77
Cervenka I, Agudelo LZ, Ruas JL (2017) Kynurenines: tryptophan’s metabolites in exercise, inflammation, and mental health. Science 357(6349):eaaf9794. https://doi.org/10.1126/science.aaf9794
Berer K, Gerdes LA, Cekanaviciute E et al (2017) Gut microbiota from multiple sclerosis patients enables spontaneous autoimmune encephalomyelitis in mice. Proc Natl Acad Sci 114:10719–10724. https://doi.org/10.1073/pnas.1711233114
Needham BD, Kaddurah-Daouk R, Mazmanian SK (2020) Gut microbial molecules in behavioural and neurodegenerative conditions. Nat Rev Neurosci 21:717–731. https://doi.org/10.1038/s41583-020-00381-0
Vogt NM, Romano KA, Darst BF et al (2018) The gut microbiota-derived metabolite trimethylamine N-oxide is elevated in Alzheimer’s disease. Alzheimers Res Ther 10:124. https://doi.org/10.1186/s13195-018-0451-2
Abdel-Haq R, Schlachetzki JCM, Glass CK, Mazmanian SK (2019) Microbiome–microglia connections via the gut–brain axis. J Exp Med 216:41–59. https://doi.org/10.1084/jem.20180794
Daneman R, Prat A (2015) The blood-brain barrier. Cold Spring Harb Perspect Biol 7:a020412. https://doi.org/10.1101/cshperspect.a020412
Krautkramer KA, Fan J, Bäckhed F (2021) Gut microbial metabolites as multi-kingdom intermediates. Nat Rev Microbiol 19:77–94. https://doi.org/10.1038/s41579-020-0438-4
Rothhammer V, Borucki DM, Tjon EC et al (2018) Microglial control of astrocytes in response to microbial metabolites. Nature 557:724–728. https://doi.org/10.1038/s41586-018-0119-x
Caspani G, Swann J (2019) Small talk: microbial metabolites involved in the signaling from microbiota to brain. Curr Opin Pharmacol 48:99–106. https://doi.org/10.1016/j.coph.2019.08.001
Blaak EE, Canfora EE, Theis S et al (2020) Short chain fatty acids in human gut and metabolic health. Benef Microbes 11:411–455. https://doi.org/10.3920/BM2020.0057
Carabotti M, Scirocco A, Maselli MA, Severi C (2015) The gut-brain axis: interactions between enteric microbiota, central and enteric nervous systems. Ann Gastroenterol 28:203–209
Morrison DJ, Preston T (2016) Formation of short chain fatty acids by the gut microbiota and their impact on human metabolism. Gut Microbes 7:189–200. https://doi.org/10.1080/19490976.2015.1134082
Silva YP, Bernardi A, Frozza RL (2020) The role of short-chain fatty acids from gut microbiota in gut-brain communication. Front Endocrinol (Lausanne) 11:25. https://doi.org/10.3389/fendo.2020.00025
Oldendorf W (1973) Carrier-mediated blood-brain barrier transport of short-chain monocarboxylic organic acids. Am J Physiol-Leg Content 224:1450–1453. https://doi.org/10.1152/ajplegacy.1973.224.6.1450
Li J-M, Yu R, Zhang L-P et al (2019) Dietary fructose-induced gut dysbiosis promotes mouse hippocampal neuroinflammation: a benefit of short-chain fatty acids. Microbiome 7:98. https://doi.org/10.1186/s40168-019-0713-7
van de Wouw M, Boehme M, Lyte JM et al (2018) Short-chain fatty acids: microbial metabolites that alleviate stress-induced brain-gut axis alterations. J Physiol 596:4923–4944. https://doi.org/10.1113/JP276431
Marizzoni M, Cattaneo A, Mirabelli P et al (2020) Short-chain fatty acids and lipopolysaccharide as mediators between gut dysbiosis and amyloid pathology in Alzheimer’s disease. J Alzheimer’s Dis 78:683–697. https://doi.org/10.3233/JAD-200306
Liu S, Li E, Sun Z et al (2019) Altered gut microbiota and short chain fatty acids in Chinese children with autism spectrum disorder. Sci Rep 9:287. https://doi.org/10.1038/s41598-018-36430-z
Zeng Q, Shen J, Chen K et al (2020) The alteration of gut microbiome and metabolism in amyotrophic lateral sclerosis patients. Sci Rep 10:12998. https://doi.org/10.1038/s41598-020-69845-8
Zhang Y, Wu S, Yi J et al (2017) Target intestinal microbiota to alleviate disease progression in amyotrophic lateral sclerosis. Clin Ther 39:322–336. https://doi.org/10.1016/j.clinthera.2016.12.014
Chen R, Xu Y, Wu P et al (2019) Transplantation of fecal microbiota rich in short chain fatty acids and butyric acid treat cerebral ischemic stroke by regulating gut microbiota. Pharmacol Res 148:104403. https://doi.org/10.1016/j.phrs.2019.104403
Kim HJ, Leeds P, Chuang D-M (2009) The HDAC inhibitor, sodium butyrate, stimulates neurogenesis in the ischemic brain. J Neurochem 110:1226–1240. https://doi.org/10.1111/j.1471-4159.2009.06212.x
Levenson JM, O’Riordan KJ, Brown KD et al (2004) Regulation of histone acetylation during memory formation in the hippocampus. J Biol Chem 279:40545–40559. https://doi.org/10.1074/jbc.M402229200
Erny D, Hrabě de Angelis AL, Jaitin D et al (2015) Host microbiota constantly control maturation and function of microglia in the CNS. Nat Neurosci 18:965–977. https://doi.org/10.1038/nn.4030
Yang LL, Millischer V, Rodin S et al (2020) Enteric short-chain fatty acids promote proliferation of human neural progenitor cells. J Neurochem 154:635–646. https://doi.org/10.1111/jnc.14928
Byrne CS, Chambers ES, Morrison DJ, Frost G (2015) The role of short chain fatty acids in appetite regulation and energy homeostasis. Int J Obes 39:1331–1338. https://doi.org/10.1038/ijo.2015.84
Parthasarathy A, Cross PJ, Dobson RCJ et al (2018) A three-ring circus: metabolism of the three proteogenic aromatic amino acids and their role in the health of plants and animals. Front Mol Biosci 5:29. https://doi.org/10.3389/fmolb.2018.00029
Tran SM-S, Mohajeri MH (2021) The role of gut bacterial metabolites in brain development. Aging Dis Nutr 13:732. https://doi.org/10.3390/nu13030732
He W, Wu G (2020) Metabolism of amino acids in the brain and their roles in regulating food intake. Adv Exp Med Biol 1265:167–185. https://doi.org/10.1007/978-3-030-45328-2-10
Rutsch A, Kantsjö JB, Ronchi F (2020) The gut-brain axis: how microbiota and host inflammasome influence brain physiology and pathology. Front Immunol 11:604179. https://doi.org/10.3389/fimmu.2020.604179
Yin J, Zhang B, Yu Z et al (2021) Ameliorative effect of dietary tryptophan on neurodegeneration and inflammation in <scp>d</scp> -galactose-induced aging mice with the potential mechanism relying on ampk/sirt1/pgc-1α pathway and gut microbiota. J Agric Food Chem 69:4732–4744. https://doi.org/10.1021/acs.jafc.1c00706
Buhot M-C, Martin S, Segu L (2000) Role of serotonin in memory impairment. Ann Med 32:210–221. https://doi.org/10.3109/07853890008998828
Jaglin M, Rhimi M, Philippe C et al (2018) Indole, a signaling molecule produced by the gut microbiota, negatively impacts emotional behaviors in rats. Front Neurosci 12:216. https://doi.org/10.3389/fnins.2018.00216
Pascucci T, Colamartino M, Fiori E et al (2020) P-cresol alters brain dopamine metabolism and exacerbates autism-like behaviors in the BTBR mouse. Brain Sci 10:233. https://doi.org/10.3390/brainsci10040233
Gacias M, Gaspari S, Santos P-MG et al (2016) Microbiota-driven transcriptional changes in prefrontal cortex override genetic differences in social behavior. Elife 5:e13442. https://doi.org/10.7554/eLife.13442
Wang Z, Klipfell E, Bennett BJ et al (2011) Gut flora metabolism of phosphatidylcholine promotes cardiovascular disease. Nature 472:57–63. https://doi.org/10.1038/nature09922
Li D, Ke Y, Zhan R et al (2018) Trimethylamine- N -oxide promotes brain aging and cognitive impairment in mice. Aging Cell 17:e12768. https://doi.org/10.1111/acel.12768
Govindarajulu M, Pinky PD, Steinke I et al (2020) Gut metabolite TMAO induces synaptic plasticity deficits by promoting endoplasmic reticulum stress. Front Mol Neurosci 13:138. https://doi.org/10.3389/fnmol.2020.00138
Brunt VE, LaRocca TJ, Bazzoni AE et al (2021) The gut microbiome–derived metabolite trimethylamine N-oxide modulates neuroinflammation and cognitive function with aging. Geroscience 43:377–394. https://doi.org/10.1007/s11357-020-00257-2
Gao Q, Wang Y, Wang X et al (2019) Decreased levels of circulating trimethylamine N-oxide alleviate cognitive and pathological deterioration in transgenic mice: a potential therapeutic approach for Alzheimer’s disease. Aging 11(19):8642–8663
Gong Z, Huang J, Xu B et al (2019) Urolithin A attenuates memory impairment and neuroinflammation in APP/PS1 mice. J Neuroinflamm 16:62. https://doi.org/10.1186/s12974-019-1450-3
Mattioli R, Francioso A, Mosca L, Silva P (2020) Anthocyanins: a comprehensive review of their chemical properties and health effects on cardiovascular and neurodegenerative diseases. Molecules 25:3809. https://doi.org/10.3390/molecules25173809
Ali T, Kim T, Rehman SU et al (2018) Natural dietary supplementation of anthocyanins via PI3K/Akt/Nrf2/HO-1 pathways mitigate oxidative stress, neurodegeneration, and memory impairment in a mouse model of Alzheimer’s disease. Mol Neurobiol 55:6076–6093. https://doi.org/10.1007/s12035-017-0798-6
Johnson SL, Kirk RD, DaSilva NA et al (2019) Polyphenol microbial metabolites exhibit gut and blood-brain barrier permeability and protect murine microglia against lps-induced inflammation. Metabolites 9:78. https://doi.org/10.3390/metabo9040078
Blacher E, Bashiardes S, Shapiro H et al (2019) Potential roles of gut microbiome and metabolites in modulating ALS in mice. Nature 572:474–480. https://doi.org/10.1038/s41586-019-1443-5
Jayaraman A, Pike CJ (2014) Alzheimer’s disease and type 2 diabetes: multiple mechanisms contribute to interactions. Curr Diab Rep 14:476. https://doi.org/10.1007/s11892-014-0476-2
Koh A, Molinaro A, Ståhlman M et al (2018) Microbially produced imidazole propionate impairs insulin signaling through mTORC1. Cell 175:947-961.e17. https://doi.org/10.1016/j.cell.2018.09.055
Zhou H, Tai J, Xu H et al (2019) Xanthoceraside could ameliorate Alzheimer’s disease symptoms of rats by affecting the gut microbiota composition and modulating the endogenous metabolite levels. Front Pharmacol 10:1035. https://doi.org/10.3389/fphar.2019.01035
Figueira I, Garcia G, Pimpão RC et al (2017) Polyphenols journey through blood-brain barrier towards neuronal protection. Sci Rep 7:11456. https://doi.org/10.1038/s41598-017-11512-6
Govindarajan N, Agis-Balboa RC, Walter J et al (2011) Sodium butyrate improves memory function in an Alzheimer’s disease mouse model when administered at an advanced stage of disease progression. J Alzheimer’s Dis 26:187–197. https://doi.org/10.3233/JAD-2011-110080
Kratsman N, Getselter D, Elliott E (2016) Sodium butyrate attenuates social behavior deficits and modifies the transcription of inhibitory/excitatory genes in the frontal cortex of an autism model. Neuropharmacology 102:136–145. https://doi.org/10.1016/j.neuropharm.2015.11.003
Wu G, Jiang Z, Pu Y et al (2022) Serum short-chain fatty acids and its correlation with motor and non-motor symptoms in Parkinson’s disease patients. BMC Neurol 22:13. https://doi.org/10.1186/s12883-021-02544-7
Reisenauer CJ, Bhatt DP, Mitteness DJ et al (2011) Acetate supplementation attenuates lipopolysaccharide-induced neuroinflammation. J Neurochem 117:264–274. https://doi.org/10.1111/j.1471-4159.2011.07198.x
Soliman ML, Combs CK, Rosenberger TA (2013) Modulation of inflammatory cytokines and mitogen-activated protein kinases by acetate in primary astrocytes. J Neuroimmune Pharmacol 8:287–300. https://doi.org/10.1007/s11481-012-9426-4
Sun M-F, Zhu Y-L, Zhou Z-L et al (2018) Neuroprotective effects of fecal microbiota transplantation on MPTP-induced Parkinson’s disease mice: gut microbiota, glial reaction and TLR4/TNF-α signaling pathway. Brain Behav Immun 70:48–60. https://doi.org/10.1016/j.bbi.2018.02.005
Curran CP, Marczinski CA (2017) Taurine, caffeine, and energy drinks: reviewing the risks to the adolescent brain. Birth Defects Res 109:1640–1648. https://doi.org/10.1002/bdr2.1177
Jakaria Md, Azam S, Haque MdE et al (2019) Taurine and its analogs in neurological disorders: focus on therapeutic potential and molecular mechanisms. Redox Biol 24:101223. https://doi.org/10.1016/j.redox.2019.101223
Kang D-W, Ilhan ZE, Isern NG et al (2018) Differences in fecal microbial metabolites and microbiota of children with autism spectrum disorders. Anaerobe 49:121–131. https://doi.org/10.1016/j.anaerobe.2017.12.007
Fujii Y, Nguyen TTT, Fujimura Y et al (2019) Fecal metabolite of a gnotobiotic mouse transplanted with gut microbiota from a patient with Alzheimer’s disease. Biosci Biotechnol Biochem 83:2144–2152. https://doi.org/10.1080/09168451.2019.1644149
Delgado PL, Charney DS, Price LH et al (1990) Serotonin function and the mechanism of antidepressant action. Reversal of antidepressant-induced remission by rapid depletion of plasma tryptophan. Arch Gen Psychiatry 47:411–418. https://doi.org/10.1001/archpsyc.1990.01810170011002
Lapin IP, Oxenkrug GF (1969) Intensification of the central serotoninergic processes as a possible determinant of the thymoleptic effect. Lancet 293:132–136. https://doi.org/10.1016/S0140-6736(69)91140-4
O’Mahony SM, Clarke G, Borre YE et al (2015) Serotonin, tryptophan metabolism and the brain-gut-microbiome axis. Behav Brain Res 277:32–48. https://doi.org/10.1016/j.bbr.2014.07.027
Kaur H, Bose C, Mande SS (2019) Tryptophan metabolism by gut microbiome and gut-brain-axis: an in silico analysis. Front Neurosci 13:1365. https://doi.org/10.3389/fnins.2019.01365
Kim D-C, Quang TH, Yoon C-S et al (2016) Anti-neuroinflammatory activities of indole alkaloids from kanjang (Korean fermented soy source) in lipopolysaccharide-induced BV2 microglial cells. Food Chem 213:69–75. https://doi.org/10.1016/j.foodchem.2016.06.068
Rothhammer V, Mascanfroni ID, Bunse L et al (2016) Type I interferons and microbial metabolites of tryptophan modulate astrocyte activity and central nervous system inflammation via the aryl hydrocarbon receptor. Nat Med 22:586–597. https://doi.org/10.1038/nm.4106
van Kessel SP, el Aidy S (2019) Bacterial metabolites mirror altered gut microbiota composition in patients with Parkinson’s disease. J Parkinsons Dis 9:S359–S370. https://doi.org/10.3233/JPD-191780
Furukawa S, Usuda K, Abe M, Ogawa I (2005) Effect of indole-3-acetic acid derivatives on neuroepithelium in rat embryos. J Toxicol Sci 30:165–174. https://doi.org/10.2131/jts.30.165
Hatano T, Saiki S, Okuzumi A et al (2016) Identification of novel biomarkers for Parkinson’s disease by metabolomic technologies. J Neurol Neurosurg Psychiatry 87:295–301. https://doi.org/10.1136/jnnp-2014-309676
Zhu W, Gregory JC, Org E et al (2016) Gut microbial metabolite TMAO enhances platelet hyperreactivity and thrombosis risk. Cell 165:111–124. https://doi.org/10.1016/j.cell.2016.02.011
Janeiro M, Ramírez M, Milagro F et al (2018) Implication of Trimethylamine N-Oxide (TMAO) in disease: potential biomarker or new therapeutic target. Nutrients 10:1398. https://doi.org/10.3390/nu10101398
Subramaniam S, Fletcher C (2018) Trimethylamine N-oxide: breathe new life. Br J Pharmacol 175:1344–1353. https://doi.org/10.1111/bph.13959
Getter T, Zaks I, Barhum Y et al (2015) A chemical chaperone-based drug candidate is effective in a mouse model of amyotrophic lateral sclerosis (ALS). ChemMedChem 10:850–861. https://doi.org/10.1002/cmdc.201500045
Acknowledgements
The authors would like to thank Manipal School of Life Sciences, Manipal Academy of Higher Education (MAHE), Manipal, India for support and infrastructure facilities. Venkidesh BS is thankful to the Manipal Academy of Higher Education for Dr. T M A Pai PhD fellowship. Kamalesh DM is grateful to the Manipal Academy of Higher Education for the intramural grant.
Funding
Open access funding provided by Manipal Academy of Higher Education, Manipal. This work was supported by the MAHE Intramural Grant (2019).
Author information
Authors and Affiliations
Contributions
KDM the idea for the article, NMS performed the literature search and drafted the manuscript; VBS, KDM, and MTS critically revised the work.
Corresponding author
Ethics declarations
Conflict of interest
The authors declare no competing interests.
Ethical approval
This article does not contain any studies with human participants performed by any authors.
Consent to participate
Not applicable.
Additional information
Publisher's Note
Springer Nature remains neutral with regard to jurisdictional claims in published maps and institutional affiliations.
Rights and permissions
Open Access This article is licensed under a Creative Commons Attribution 4.0 International License, which permits use, sharing, adaptation, distribution and reproduction in any medium or format, as long as you give appropriate credit to the original author(s) and the source, provide a link to the Creative Commons licence, and indicate if changes were made. The images or other third party material in this article are included in the article's Creative Commons licence, unless indicated otherwise in a credit line to the material. If material is not included in the article's Creative Commons licence and your intended use is not permitted by statutory regulation or exceeds the permitted use, you will need to obtain permission directly from the copyright holder. To view a copy of this licence, visit http://creativecommons.org/licenses/by/4.0/.
About this article
Cite this article
Swer, N.M., Venkidesh, B., Murali, T.S. et al. Gut microbiota-derived metabolites and their importance in neurological disorders. Mol Biol Rep 50, 1663–1675 (2023). https://doi.org/10.1007/s11033-022-08038-0
Received:
Accepted:
Published:
Issue Date:
DOI: https://doi.org/10.1007/s11033-022-08038-0