Abstract
Until the demonstration little more than 20 years ago that glycogenolysis occurs during normal whisker stimulation glycogenolysis was regarded as a relatively uninteresting emergency procedure. Since then, a series of important astrocytic functions has been shown to be critically dependent on glycogenolytic activity to support the signaling mechanisms necessary for these functions to operate. This applies to glutamate formation and uptake and to release of ATP as a transmitter, stimulated by other transmitters or elevated K+ concentrations and affecting not only other astrocytes but also most other brain cells. It is also relevant for astrocytic K+ uptake both during the period when the extracellular K+ concentration is still elevated after neuronal excitation, and capable of stimulating glycogenolytic activity, and during the subsequent undershoot after intense neuronal activity, when glycogenolysis may be stimulated by noradrenaline. Both elevated K+ concentrations and several transmitters, including the β-adrenergic agonist isoproterenol and vasopressin increase free cytosolic Ca2+ concentration in astrocytes, which stimulates phosphorylase kinase so that it activates the transformation of the inactive glycogen phosphorylase a to the active phosphorylase b. Contrary to common belief cyclic AMP plays at most a facilitatory role, and only when free cytosolic Ca2+ concentration is also increased. Cyclic AMP is not increased during activation of glycogenolysis by either elevated K+ concentrations or the stimulation of the serotonergic 5-HT2B receptor. Not all agents that stimulate glycogenolysis do so by directly activating phophorylase kinase—some do so by activating processes requiring glycogenolysis, e.g. for synthesis of glutamate.













Similar content being viewed by others
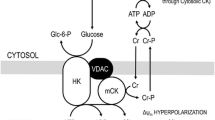
References
Abbracchio MP, Ceruti S (2006) Roles of P2 receptors in glial cells: focus on astrocytes. Purinergic Signal 2:595–604
Andersen B, Rassov A, Westergaard N, Lundgren K (1999) Inhibition of glycogenolysis in primary rat hepatocytes by 1, 4-dideoxy-1,4-imino-D-arabinitol. Biochem J 342:545–550
Bay V, Butt AM (2012) Relationship between glial potassium regulation and axon excitability: a role for glial Kir4.1 channels. Glia 60:651–660
Bekar LK, Walz W (2002) Intracellular chloride modulates A-type potassium currents in astrocytes. Glia 39:207–216
Bernard PA, Makin CE, Werner HA (2009) Hypoglycemia associated with dexmedetomidine overdose in a child? J Clin Anesth 21:50–53
Bonhaus DW, Bach C, DeSouza A, Salazar FH, Matsuoka BD, Zuppan P, Chan HW, Eglen RM (1995) The pharmacology and distribution of human 5-hydroxytryptamine2B (5-HT2B) receptor gene products: comparison with 5-HT2A and 5-HT2C receptors. Br J Pharmacol 115:622–628
Brown AM, Sickmann HM, Fosgerau K et al (2005) Astrocyte glycogen metabolism is required for neural activity during aglycemia or intense stimulation in mouse white matter. J Neurosci Res 79:74–80
Butt AM (2011) ATP: a ubiquitous gliotransmitter integrating neuron-glial networks. Semin Cell Dev Biol 22:205–213
Cai L, Du T, Song D, Li B, Hertz L, Peng L (2011) Astrocyte ERK phosphorylation precedes K+-induced swelling but follows hypotonicity-induced swelling. Neuropathology 31:250–264
Chen Y, Hertz L (1999) Noradrenaline effects on pyruvate decarboxylation: correlation with calcium signaling. J Neurosci Res 58:599–606
Chen Y, McNeill JR, Hajek I, Hertz L (1992) Effect of vasopressin on brain swelling at the cellular level: do astrocytes exhibit a furosemide–vasopressin-sensitive mechanism for volume regulation? Can J Physiol Pharmacol 70:S367–S373
Chen Y, Zhao Z, Hertz L (2000) Vasopressin increases [Ca2+]i in differentiated astrocytes by activation of V1b/V3 receptors but has no effect in mature cortical neurons. J Neurosci Res 60:761–766
Chever O, Djukic B, McCarthy KD, Amzica F (2010) Implication of Kir4.1 channel in excess potassium clearance: an in vivo study on anesthetized glial-conditional Kir4.1 knock-out mice. J Neurosci 30:15769–15777
Choi DS, Maroteaux L (1996) Immunohistochemical localisation of the serotonin 5-HT2B receptor in mouse gut, cardiovascular system, and brain. FEBS Lett 391:45–51
Choi HB, Gordon GR, Zhou N, Tai C, Rungta RL, Martinez J, Milner TA, Ryu JK, McLarnon JG, Tresquerres M, Levin LR, Buck J, MacVicar BA (2012) Metabolic communication between astrocytes and neurons via bicarbonate-responsive soluble adenylyl cyclase. Neuron 75:1094–1104
Cruz NF, Dienel GA (2002) High glycogen levels in brains of rats with minimal environmental stimuli: implications for metabolic contributions of working astrocytes. J Cereb Blood Flow Metab 22:1476–1489
Daisley JN, Gruss M, Rose SP, Braun K (1998) Passive avoidance training and recall are associated with increased glutamate levels in the intermediate medial hyperstriatum ventrale of the day-old chick. Neural Plast 6:53–61
D’Ambrosio R, Gordon DS, Winn HR (2002) Differential role of KIR channel and Na+/K+-pump in the regulation of extracellular K+ in rat hippocampus. J Neurophysiol 87:87–102
Diaz SL, Doly S, Narboux-Nême N, Fernández S, Mazot P, Banas SM, Boutourlinsky K, Moutkine I, Belmer A, Roumier A, Maroteaux L (2012) 5-HT2B receptors are required for serotonin-selective antidepressant actions. Mol Psychiatry 17:154–163
Dietzel I, Heinemann U, Hofmeier G, Lux HD (1982) Stimulus-induced changes in extracellular Na+ and Cl− concentration in relation to changes in the size of the extracellular space. Exp Brain Res 46:73–84
Dietzel I, Heinemann U, Lux HD (1989) Relations between slow extracellular potential changes, glial potassium buffering, and electrolyte and cellular volume changes during neuronal hyperactivity in cat brain. Glia 2:25–44
DiNuzzo M (2013) Kinetic analysis of glycogen turnover: relevance to human brain 13C NMR spectroscopy. J Cereb Blood Flow Metab 33:1540–1548
DiNuzzo M, Mangia S, Maraviglia B, Giove F (2012) The role of astrocytic glycogen in supporting the energetics of neuronal activity. Neurochem Res 37:2432–2438
Du T, Li B, Li H, Li M, Hertz L, Peng L (2010) Signaling pathways of isoproterenol-induced ERK1/2 phosphorylation in primary cultures of astrocytes are concentration-dependent. J Neurochem 115:1007–1023
Du T, Liang C, Li B, Hertz L, Peng L (2014) Chronic fluoxetine administration increases expression of the L-channel gene Cav1.2 in astrocytes from the brain of treated mice and in culture and augments K+-induced increase in [Ca2+]i. Cell Calcium. doi:10.1016/j.ceca.2014.01.002
Dufour S, Dufour P, Chever O, Vallee R, Amzica F (2011) In vivo simultaneous intra- and extracellular potassium recordings using a micro-optrode. J Neurosci Methods 194:206–217
Egawa K, Yamada J, Furukawa T, Yanagawa Y, Fukuda A (2013) Cl− homeodynamics in gap junction-coupled astrocytic networks on activation of GABAergic synapses. J Physiol 591:3901–3917
Elekes O, Venema K, Postema F, Dringen R, Hamprecht B, Korf J (1996) Evidence that stress activates glial lactate formation in vivo assessed with rat hippocampus lactography. Neurosci Lett 208:69–72
Enkvist MO, Hämäläinen H, Jansson CC, Kukkonen JP, Hautala R, Courtney MJ, Akerman KE (1996) Coupling of astroglial alpha 2-adrenoreceptors to second messenger pathways. J Neurochem 66:2394–2401
Eriksen JL, Gillespie RA, Druse MJ (2000) Effects of in utero ethanol exposure and maternal treatment with a 5-HT1A agonist on S100B-containing glial cells. Brain Res Dev Brain Res 121:133–143
Garrison JC, Borland MK (1979) Regulation of mitochondrial pyruvate carboxylation and gluconeogenesis in rat hepatocytes via an alpha-adrenergic, adenosine 3′:5′-monophosphate-independent mechanism. J Biol Chem 254:1129–1133
Gibbs M, Hertz L (2014) Serotonin mediation of early memory formation via 5-HT2B receptor-induced glycogenolysis in the day-old chick. Front Pharmacol. doi:10.3389/fphar.2014.00054
Gibbs M, Lloyd H, Santa T, Hertz L (2007) Glycogen is a preferred glutamate precursor during learning in 1-day-old chick: biochemical and behavioral evidence. J Neurosci Res 85:3326–3333
Gibbs ME, Hutchinson D, Hertz L (2008) Astrocytic involvement in learning and memory consolidation. Neurosci Biobehav Rev 32(5):927–944
Grisar T, Franck G, Schoffeniels E (1980) Glial control of neuronal excitability in mammals: II. Enzymatic evidence: two molecular forms of the Na+, K+-ATPase in brain. Neurochem Int 2C:311–320
Håberg A, Qu H, Haraldseth O, Unsgård G, Sonnewald U (2000) In vivo effects of adenosine A1 receptor agonist and antagonist on neuronal and astrocytic intermediary metabolism studied with ex vivo 13C NMR spectroscopy. J Neurochem 74:327–333
Hajek I, Subbarao KV, Hertz L (1996) Acute and chronic effects of potassium and noradrenaline on Na+, K+-ATPase activity in cultured mouse neurons and astrocytes. Neurochem Int 28:335–342
Hamann S, Herrera-Perez JJ, Zeuthen T, Alvarez-Leefmans FJ (2010) Cotransport of water by the Na+-K+-2Cl− cotransporter NKCC1 in mammalian epithelial cells. J Physiol 588:4089–4101
Hamilton N, Vayro S, Kirchhoff F, Verkhratsky A, Robbins J, Gorecki DC, Butt AM (2008) Mechanisms of ATP- and glutamate-mediated calcium signaling in white matter astrocytes. Glia 56:734–749
Harik SI, Busto R, Martinez E (1982) Norepinephrine regulation of cerebral glycogen utilization during seizures and ischemia. J Neurosci 2:409–414
Henn FA, Haljamae H, Hamberger A (1972) Glial cell function: active control of extracellular K+ concentration. Brain Res 43:437–443
Hertz L (2008) Bioenergetics of cerebral ischemia: a cellular perspective. Neuropharmacology 55:289–309
Hertz L, Peng L, Dienel GA (2007) Energy metabolism in astrocytes: high rate of oxidative metabolism and spatiotemporal dependence on glycolysis/glycogenolysis. J Cereb Blood Flow Metab 27:219–249
Hertz L, Li B, Song D, Ren J, Dong L, Chen Y, Peng L (2012) Astrocytes as a 5-HT2B-mediated SERT-independent SSRI target, slowly altering depression-associated genes and function. Curr Signal Transduct Ther 7:65–80
Hertz L, Xu J, Song D, Du T, Yan E, Peng L (2013a) Brain glycogenolysis, adrenoceptors, pyruvate carboxylase, Na+, K+-ATPase and Marie E. Gibbs’ pioneering learning studies. Front Integr Neurosci 7:20
Hertz L, Xu J, Peng L (2013b) Glycogenolysis and purinergic signaling. In: Parpura V, Schousboe A, Verkhratsky A (eds) Glutamate and ATP at interface of metabolism and signaling in the brain, in Advances in neurobiology series, series editor A. Springer-Verlag, Berlin Heidelberg, Schousboe, in press
Hertz L, Song D, Li Baoman, Du T, Xu J, Gu L, Chen Y, Peng L (2014) Signal transduction in astrocytes during chronic or acute treatment with drugs (SSRIs; anti-bipolar drugs; GABA-ergic drugs; benzodiazepines) ameliorating mood disorders. J Signal Transduct. doi:10.1155/2014/593934
Hof PR, Pascale E, Magistretti PJ (1988) K+ at concentrations reached in the extracellular space during neuronal activity promotes a Ca2+-dependent glycogen hydrolysis in mouse cerebral cortex. J Neurosci 8:1922–1928
Hyder F, Rothman DL (2012) Quantitative fMRI and oxidative neuroenergetics. Neuroimage 62:985–994
Ibrahim MZ (1975) Glycogen and its related enzymes of metabolism in the central nervous system. Adv Anat Embryol Cell Biol 52:3–89
Illes P, Verkhratsky A, Burnstock G, Franke H (2012) P2X receptors and their roles in astroglia in the central and peripheral nervous system. Neuroscientist 18:422–438
Jacobson KA, Gao ZG (2006) Adenosine receptors as therapeutic targets. Nat Rev Drug Discov 5:247–264
Kaufman E, Driscoll B (1992) Carbon dioxide fixation in neuronal and astroglial cells in culture. J Neurochem 58:258–262
Kirischuk S, Parpura V, Verkhratsky A (2012) Sodium dynamics: another key to astroglial excitability? Trends Neurosci 35:497–506
Kong EK, Peng L, Chen Y, Yu AC, Hertz L (2002) Up-regulation of 5-HT2B receptor density and receptor-mediated glycogenolysis in mouse astrocytes by long-term fluoxetine administration. Neurochem Res 27:113–120
Kuffler SW, Nicholls JG, Orkand RK (1966) Physiological properties of glial cells in the central nervous system of amphibia. J Neurophysiol 29:768–787
Kursar JD, Nelson DL, Wainscott DB, Baez M (1994) Molecular cloning, functional expression, and mRNA tissue distribution of the human 5-hydroxytryptamine2B receptor. Mol Pharmacol 46:227–234
Lalo U, Verkhratsky A, Pankratov Y (2012) Ionotropic ATP receptors in neuronal-glial communication. Semin Cell Dev Biol 22:220–228
Li B, Zhang S, Zhang H, Nu W, Cai L, Hertz L, Peng L (2008) Fluoxetine-mediated 5-HT2B receptor stimulation in astrocytes causes EGF receptor transactivation and ERK phosphorylation. Psychopharmacology (Berl) 201:443–458
Li B, Zhang S, Li M, Hertz L, Peng L (2010) Serotonin increases ERK1/2 phosphorylation in astrocytes by stimulation of 5-HT2B and 5-HT2C receptors. Neurochem Int 57:432–439
Li B, Zhang S, Zhang H, Hertz L, Peng L (2011) Fluoxetine affects GluK2 editing, glutamate-evoked Ca2+ influx and extracellular signal-regulated kinase phosphorylation in mouse astrocytes. J Psychiatry Neurosci 36:322–338
Li B, Dong L, Wang B, Cai L, Jiang N, Peng L (2012) Cell type-specific gene expression and editing responses to chronic fluoxetine treatment in the in vivo mouse brain and their relevance for stress-induced anhedonia. Neurochem Res 37:2480–2495
Liedtke CM, Cole TS (2002) Activation of NKCC1 by hyperosmotic stress in human tracheal epithelial cells involves PKC-delta and ERK. Biochim Biophys Acta 1589:77–88
Linden J, Taylor HE, Robeva AS, Tucker AL, Stehle JH, Rivkees SA, Fink JS, Reppert SM (1993) Molecular cloning and functional expression of a sheep A3 adenosine receptor with widespread tissue distribution. Mol Pharmacol 44:524–532
Lothman E, Lamanna J, Cordingley G, Rosenthal M, Somjen G (1975) Responses of electrical potential, potassium levels, and oxidative metabolic activity of the cerebral neocortex of cats. Brain Res 88:15–36
Macaulay N, Zeuthen T (2012) Glial K+ clearance and cell swelling: key roles for cotransporters and pumps. Neurochem Res 37:2299–2309
Magistretti PJ (1988) Regulation of glycogenolysis by neurotransmitters in the central nervous system. Diabete Metab 14:237–246
Mangia S, Giove F, DiNuzzo M (2012) Metabolic pathways and activity-dependent modulation of glutamate concentration in the human brain. Neurochem Res 37:2554–2561
Martinez-Hernandez A, Bell KP, Norenberg MD (1977) Glutamine synthetase: glial localization in brain. Science 195:1356–1358
McKenna MC (2013) Glutamate pays its own way in astrocytes. Front Endocrinol. doi:10.3389/fendo.2013.00191
Meier SD, Kafitz KW, Rose CR (2008) Developmental profile and mechanisms of GABA-induced calcium signaling in hippocampal astrocytes. Glia 56:1127–1137
Neary JT, van Breemen C, Forster E, Norenberg LO, Norenberg MD (1988) ATP stimulates calcium influx in primary astrocyte cultures. Biochem Biophys Res Commun 157:1410–1416
Norenberg MD, Martinez-Hernandez A (1979) Fine structural localization of glutamine synthetase in astrocytes of rat brain. Brain Res 161:303–310
Obel LF, Andersen KM, Bak LK, Schousboe A, Waagepetersen HS (2012) Effects of adrenergic agents on intracellular Ca2+ homeostasis and metabolism of glucose in astrocytes with an emphasis on pyruvate carboxylation, oxidative decarboxylation and recycling: implications for glutamate neurotransmission and excitotoxicity. Neurotox Res 21:405–417
Offermanns S, Simon MI (1995) G alpha 15 and G alpha 16 couple a wide variety of receptors to phospholipase C. J Biol Chem 270:15175–15180
Öz G, Tesfaye N, Kumar A, Deelchand DK, Eberly LE, Seaquist ER (2012) Brain glycogen content and metabolism in subjects with type 1 diabetes and hypoglycemia unawareness. J Cereb Blood Flow Metab 32:256–263
Ozawa E (1972) Activation of muscular phosphorylase b kinase by a minute amount of Ca ion. J Biochem 71:321–331
Ozawa E (2011) Regulation of phosphorylase kinase by low concentrations of Ca ions upon muscle contraction: the connection between metabolism and muscle contraction and the connection between muscle physiology and Ca-dependent signal transduction. Proc Jpn Acad Ser B Phys Biol Sci 87:486–508
Patel AB, Lai JC, Chowdhury GM, Hyder F, Rothman DL, Shulman RG, Behar KL (2014) Direct evidence for activity-dependent glucose phosphorylation in neurons: implications for the astrocyte-to-neuron lactate shuttle. Proc Natl Acad Sci USA doi:10.1073/pnas.1403576111
Pedersen SF, O’Donnell ME, Anderson SE, Cala PM (2006) Physiology and pathophysiology of Na+/H+ exchange and Na+-K+-2Cl− cotransport in the heart, brain, and blood. Am J Physiol Regul Integr Comp Physiol 291:R1–R25
Porter RH, Benwell KR, Lamb H, Malcolm CS, Allen NH, Revell DF, Adams DR, Sheardown MJ (1999) Functional characterization of agonists at recombinant human 5-HT2A, 5-HT2B and 5-HT2C receptors in CHO-K1 cells. Br J Pharmacol 128:13–20
Ransom CB, Ransom BR, Sontheimer H (2000) Activity-dependent extracellular K+ accumulation in rat optic nerve: the role of glial and axonal Na+ pumps. J Physiol 522:427–442
Sajjadi FG, Firestein GS (1993) cDNA cloning and sequence analysis of the human A3 adenosine receptor. Biochim Biophys Acta 1179:105–107
Sandén N, Thorlin T, Blomstrand F, Persson PA, Hansson E (2000) 5-Hydroxytryptamine2B receptors stimulate Ca2+ increases in cultured astrocytes from three different brain regions. Neurochem Int 36:427–434
Scemes E, Spray DC (2012) Extracellular K+ and astrocyte signaling via connexin and pannexin channels. Neurochem Res 37:2310–2316
Schousboe A, Bak LK, Waagepetersen HS (2013) Astrocytic control of biosynthesis and turnover of the neurotransmitters glutamate and GABA. Front Endocrinol (Lausanne) 4:102
Seidel JL, Shuttleworth CW (2011) Contribution of astrocyte glycogen stores to progression of spreading depression and related events in hippocampal slices. Neuroscience 192:295–303
Semplicini A, Serena L, Valle R, Ceolotto G, Felice M, Fontebasso A, Pessina AC (1995) Ouabain-inhibiting activity of aldosterone antagonists. Steroids 60:110–113
Sibson NR, Dhankhar A, Mason GF, Rothman DL, Behar KL, Shulman RG (1998) Stoichiometric coupling of brain glucose metabolism and glutamatergic neuronal activity. Proc Natl Acad Sci U S A 95:316–321
Sickmann HM, Walls AB, Schousboe A, Bounman SD, Waaqepetersen HS (2009) Functional significance of brain glycogen in sustaining glutamatergic neurotransmission. J Neurochem 109:80–86
Sickmann HM, Waagepetersen HS, Schousboe A, Benie AJ, Bounman SD (2012) Brain glycogen and its role in supporting glutamate and GABA homeostasis in a type 2 diabetes rat model. Neurochem Int 60:267–275
Somjen GG, Kager H, Wadman WJ (2008) Computer simulations of neuron-glia interactions mediated by ion flux. J Comput Neurosci 25:349–365
Song H, Thompson SM, Blaustein MP (2013) Nanomolar ouabain augments Ca2+ signalling in rat hippocampal neurones and glia. J Physiol 591:1671–1689
Sorg O, Pellerin L, Stolz M, Beggah S, Magistretti PJ (1995) Adenosine triphosphate and arachidonic acid stimulate glycogenolysis in primary cultures of mousecerebral cortical astrocytes. Neurosci Lett 188:109–112
Subbarao KV, Hertz L (1990) Effect of adrenergic agonists on glycogenolysis in primary cultures of astrocytes. Brain Res 536:220–226
Swanson RA, Morton MM, Sagar SM, Sharp FR (1992) Sensory stimulation induces local cerebral glycogenolysis: demonstration by autoradiography. Neuroscience 51:451–461
van Calker D, Müller M, Hamprecht B (1979) Adenosine regulates via two different types of receptors, the accumulation of cyclic AMP in cultured brain cells. J Neurochem 33:999–1005
van Ree JM, Hijman R, Jolles J, De Wied D (1985) Vasopressin and related peptides: animal and human studies. Prog Neuropsychopharmacol Biol Psychiatry 9:551–559
Verkhratsky A, Krishtal OA, Burnstock G (2009) Purinoceptors on neuroglia. Mol Neurobiol 39:190–208
Ververken D, Van Veldhoven P, Proost C, Carton H, De Wulf H (1982) On the role of calcium ions in the regulation of glycogenolysis in mouse brain cortical slices. J Neurochem 38:1286–1295
Walls AB, Heimbürger CM, Bouman SD, Schousboe A, Waagepetersen HS (2009) Robust glycogen shunt activity in astrocytes: Effects of glutamatergic and adrenergic agents. Neuroscience 158:284–292
Walz W (2000) Role of astrocytes in the clearance of excess extracellular potassium. Neurochem Int 36:291–300
Walz W, Hertz L (1982) Ouabain-sensitive and ouabain-resistant net uptake of potassium into astrocytes and neurons in primary cultures. J Neurochem 39:70–77
Walz W, Hertz L (1984) Intense furosemide-sensitive potassium accumulation in astrocytes in the presence of pathologically high extracellular potassium levels. J Cereb Blood Flow Metab 4:301–304
Wang F, Smith NA, Xu Q, Fujita T, Matsuda T, Takano T, Bekar L, Nedergaard M (2012a) Astrocytes modulate neural network activity by Ca2+ -dependent uptake of extracellular K+. Sci Signal 5:ra26
Wang F, Xu Q, Wang W, Takano T, Nedergaard M (2012b) Bergmann glia modulate cerebellar Purkinje cell bistability via Ca2+-dependent K+ uptake. Proc Natl Acad Sci U S A 109:7911–7916
Xia M, Zhu Y (2011) Signaling pathways of ATP-induced PGE2 release in spinal cordastrocytes are EGFR transactivation-dependent. Glia 59:664–674
Xiong ZQ, Stringer JL (2000) Sodium pump activity, not glial spatial buffering, clears potassium after epileptiform activity induced in the dentate gyrus. J Neurophysiol 83:1443–1451
Xu J, Song D, Xue Z, Gu L, Hertz L, Peng L (2013) Requirement of glycogenolysis for uptake of increased extracellular K+ in astrocytes: potential implications for K+ homeostasis and glycogen usage in brain. Neurochem Res 38:472–485
Xu J, Song D, Bai Q, Hertz L, Peng L (2014a) Basic mechanisms leading to stimulation of glycogenolysis by isoproterenol, EGF, elevated extracellular K+ concentrations, or GABA. Neurochem Res 39:661–667
Xu J, Song D, Bai Q, Zhou L, Cai L, Hertz L, Peng L (2014b) Role of glycogenolysis in stimulation of ATP release from cultured mouse astrocytes by transmitters and high K+ concentrations. ASN Neuro 6(1), art:e0000x. doi:10.1042/AN200130040
Yan E, Li B, Gu L, Hertz L, Peng L (2013) Mechanisms for L-channel-mediated increase in [Ca2+]i and its reduction by anti-bipolar drugs in cultured astrocytes combined with its mRNA expression in freshly isolated cells support the importance of astrocytic L-channels. Cell Calcium 54:335–342
Yang IH, Tsai YT, Chiu SJ, Liu LT, Lee HH, Hou MF, Hsu WL, Chen BK, Chang WC (2013) Involvement of STIM1 and Orai1 in EGF-mediated cell growth in retinal pigment epithelial cells. J Biomed Sci 20:41
Young SZ, Platel JC, Nielsen JV, Jensen NA, Bordey A (2010) GABAA increases calcium in subventricular zone astrocyte-like cells through L- and T-type voltage-gated calcium channels. Front Cell Neurosci 4:8
Zhang X, Peng L, Chen Y, Hertz L (1993) Stimulation of glycogenolysis in astrocytes by fluoxetine, an antidepressant acting like 5-HT. Neuroreport 4:1235–1238
Zhang S, Li B, Lovatt D, Xu J, Song D, Goldman SA, Nedergaard M, Hertz L, Peng L (2010) 5-HT2B receptors are expressed on astrocytes from brain and in culture and are a chronic target for all five conventional ‘serotonin-specific reuptake inhibitors’. Neuron Glia Biol 6:113–125
Zhou QY, Li C, Olah ME, Johnson RA, Stiles GL, Civelli O (1992) Molecular cloning and characterization of an adenosine receptor: the A3 adenosine receptor. Proc Natl Acad Sci U S A 89:7432–7436
Author information
Authors and Affiliations
Corresponding author
Rights and permissions
About this article
Cite this article
Hertz, L., Xu, J., Song, D. et al. Astrocytic glycogenolysis: mechanisms and functions. Metab Brain Dis 30, 317–333 (2015). https://doi.org/10.1007/s11011-014-9536-1
Received:
Accepted:
Published:
Issue Date:
DOI: https://doi.org/10.1007/s11011-014-9536-1