Abstract
Diffuse large B cell lymphoma (DLBCL) is a highly heterogeneous disease with varying therapeutic responses and prognoses. Angiogenesis is a crucial factor in lymphoma growth and progression, but no scoring model based on angiogenesis-related genes (ARGs) has been developed for prognostic evaluation of DLBCL patients. In this study, we used univariate Cox regression to identify prognostic ARGs and found two distinct clusters of DLBCL patients in the GSE10846 dataset based on the expression of these prognostic ARGs. These two clusters had different prognoses and immune cell infiltration. Using LASSO regression analysis, we constructed a novel seven-ARG-based scoring model in GSE10846 dataset, and it was further validated in the GSE87371 dataset. The DLBCL patients were divided into high- and low-score groups based on the median risk score as a cut-off. The high-score group had a worse prognosis and showed higher expression of immune checkpoints, M2 macrophages, myeloid-derived suppressor cells, and regulatory T cells, indicating a stronger immunosuppressive environment. DLBCL patients in high-score group were resistant to doxorubicin and cisplatin, which are components of frequently used chemotherapy regimens, but more sensitive to gemcitabine and temozolomide. Using RT-qPCR, we found that two candidate risk genes, RAPGEF2 and PTGER2, were over-expressed in DLBCL tissues compared with control tissues. Taken together, the ARG-based scoring model provides a promising direction for the prognosis and immune status of DLBCL patients, and benefits the development of personalized treatment for DLBCL patients.










Similar content being viewed by others
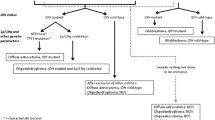
Data availability
The datasets generated and/or analyzed during the current study are available in the GEO dataset (https://www.ncbi.nlm.nih.gov/geo/).
References
Susanibar-Adaniya S, Barta SK. 2021 Update on Diffuse large B cell lymphoma: a review of current data and potential applications on risk stratification and management. Am J Hematol. 2021;96:617–29.
Borsarelli Carvalho Brito A, Torresan Delamain M, de Oliveira C, Antonio de Souza C, Vassallo J, Lima SPC. Polymorphisms in key regulator genes of the intrinsic apoptosis pathway in risk and clinical presentation of diffuse large B-cell lymphoma. Hematol Oncol. 2017;35:911–3.
Li S, Young KH, Medeiros LJ. Diffuse large B-cell lymphoma. Pathology. 2018;50:74–87.
Rutherford SC, Leonard JP. DLBCL cell of origin: what role should it play in care today? Oncology (Williston Park). 2018;32:445–9.
Jiang L, Li N. B-cell non-Hodgkin lymphoma: importance of angiogenesis and antiangiogenic therapy. Angiogenesis. 2020;23:515–29.
Wu J, Ma S, Sun L, et al. Prognostic value of microvessel density in non-hodgkin lymphoma: a meta-analysis. Acta Haematol. 2021;144:603–12.
Solimando AG, Annese T, Tamma R, et al. New insights into diffuse large B-cell lymphoma pathobiology. Cancers (Basel). 2020;12:1869.
Brito ABC, Delamain MT, Fanelli MF, et al. Angiogenesis’ related genetic variants alter clinical features and prognosis of diffuse large B-cell lymphoma patients. Tumour Biol. 2021;43:129–40.
Ribatti D, Nico B, Ranieri G, Specchia G, Vacca A. The role of angiogenesis in human non-Hodgkin lymphomas. Neoplasia. 2013;15:231–8.
Cardesa-Salzmann TM, Colomo L, Gutierrez G, et al. High microvessel density determines a poor outcome in patients with diffuse large B-cell lymphoma treated with rituximab plus chemotherapy. Haematologica. 2011;96:996–1001.
Lenz G, Wright G, Dave SS, et al. Stromal gene signatures in large-B-cell lymphomas. N Engl J Med. 2008;359:2313–23.
Arneth B. Tumor Microenvironment. Medicina (Kaunas). 2019;56:15.
Lei X, Lei Y, Li JK, et al. Immune cells within the tumor microenvironment: biological functions and roles in cancer immunotherapy. Cancer Lett. 2020;470:126–33.
Tamma R, Ranieri G, Ingravallo G, et al. Inflammatory cells in diffuse large B cell lymphoma. J Clin Med. 2020;9:2418.
Nicholas NS, Apollonio B, Ramsay AG. Tumor microenvironment (TME)-driven immune suppression in B cell malignancy. Biochim Biophys Acta. 2016;1863:471–82.
Jiang X, Wang J, Deng X, et al. Role of the tumor microenvironment in PD-L1/PD-1-mediated tumor immune escape. Mol Cancer. 2019;18:10.
Wight JC, Chong G, Grigg AP, Hawkes EA. Prognostication of diffuse large B-cell lymphoma in the molecular era: moving beyond the IPI. Blood Rev. 2018;32:400–15.
Wang L, Li LR. R-CHOP resistance in diffuse large B-cell lymphoma: biological and molecular mechanisms. Chin Med J (Engl). 2020;134:253–60.
Major A, Smith SM. DA-R-EPOCH vs R-CHOP in DLBCL: how do we choose? Clin Adv Hematol Oncol. 2021;19:698–709.
Ting CY, Gan GG, Bee-Lan Ong D, Tan SY, Bee PC. Extranodal site of diffuse large B-cell lymphoma and the risk of R-CHOP chemotherapy resistance and early relapse. Int J Clin Pract. 2020;74:e13594.
He MY, Kridel R. Treatment resistance in diffuse large B-cell lymphoma. Leukemia. 2021;35:2151–65.
Crombie JL, Armand P. Diffuse large B-cell lymphoma and high-grade B-cell lymphoma: genetic classification and its implications for prognosis and treatment. Hematol Oncol Clin North Am. 2019;33:575–85.
Wang L, Li LR, Young KH. New agents and regimens for diffuse large B cell lymphoma. J Hematol Oncol. 2020;13:175.
Schmitz R, Wright GW, Huang DW, et al. Genetics and pathogenesis of diffuse large B-cell lymphoma. N Engl J Med. 2018;378:1396–407.
He J, Chen Z, Xue Q, et al. Identification of molecular subtypes and a novel prognostic model of diffuse large B-cell lymphoma based on a metabolism-associated gene signature. J Transl Med. 2022;20:186.
Cui Y, Leng C. A glycolysis-related gene signatures in diffuse large B-Cell lymphoma predicts prognosis and tumor immune microenvironment. Front Cell Dev Biol. 2023;11:1070777.
Luo C, Nie H, Yu L. Identification of aging-related genes associated with prognostic value and immune microenvironment characteristics in diffuse large B-cell lymphoma. Oxid Med Cell Longev. 2022;2022:3334522.
Qiu CJ, Wang XB, Zheng ZR, et al. Development and validation of a ferroptosis-related prognostic model in pancreatic cancer. Invest New Drugs. 2021;39:1507–22.
Li X-y, You J-x, Zhang L-y, Su L-x, Yang X-t. A novel model based on necroptosis-related genes for predicting prognosis of patients with prostate adenocarcinoma. Front Bioeng Biotechnol. 2022;9:1419.
Zhao J, Liu Z, Zheng X, Gao H, Li L. Prognostic model and nomogram construction based on a novel ferroptosis-related gene signature in lower-grade glioma. Front Genet. 2021;12:753680.
Tang B, Zhang X, Yang X, Wang W, Li R, Liu Y. Construction and validation of an angiogenesis-related scoring model to predict prognosis, tumor immune microenvironment and therapeutic response in hepatocellular carcinoma. Front Immunol. 2022;13:1013248.
Tan X, Liao Z, Zou S, Ma L, Wang A. VASH2 promotes cell proliferation and resistance to doxorubicin in non-small cell lung cancer via AKT signaling. Oncol Res. 2020;28:3–11.
Zhang Y, Xue X, Zhao X, et al. Vasohibin 2 promotes malignant behaviors of pancreatic cancer cells by inducing epithelial-mesenchymal transition via Hedgehog signaling pathway. Cancer Med. 2018;7:5567–76.
Yamamoto M, Ozawa S, Koyanagi K, et al. Clinicopathological role of vasohibin in gastroenterological cancers: a meta-analysis. Tohoku J Exp Med. 2022;256:291–301.
Iwamoto K, Takahashi H, Okuzaki D, et al. Syntenin-1 promotes colorectal cancer stem cell expansion and chemoresistance by regulating prostaglandin E2 receptor. Br J Cancer. 2020;123:955–64.
Wu Q, Hu Y, Ma Q, et al. Comprehensive analysis of RAPGEF2 for predicting prognosis and immunotherapy response in patients with hepatocellular carcinoma. J Oncol. 2022;2022:6560154.
Khanna P, Soh HJ, Chen CH, et al. ACE2 abrogates tumor resistance to VEGFR inhibitors suggesting angiotensin-(1–7) as a therapy for clear cell renal cell carcinoma. Sci Transl Med. 2021;13:0170.
Zhang Q, Lu S, Li T, et al. ACE2 inhibits breast cancer angiogenesis via suppressing the VEGFa/VEGFR2/ERK pathway. J Exp Clin Cancer Res. 2019;38:173.
Yin R, Guo L, Gu J, Li C, Zhang W. Over expressing miR-19b-1 suppress breast cancer growth by inhibiting tumor microenvironment induced angiogenesis. Int J Biochem Cell Biol. 2018;97:43–51.
Zhang F, Zhou Q. Knockdown of BRCC3 exerts an anti-tumor effect on cervical cancer in vitro. Mol Med Rep. 2018;18:4886–94.
Tu Z, Xu B, Qu C, et al. BRCC3 acts as a prognostic marker in nasopharyngeal carcinoma patients treated with radiotherapy and mediates radiation resistance in vitro. Radiat Oncol. 2015;10:123.
Hu Y, Zhang Y, Ding M, Xu R. Long noncoding RNA TMPO-AS1/miR-126-5p/BRCC3 axis accelerates gastric cancer progression and angiogenesis via activating PI3K/Akt/mTOR pathway. J Gastroenterol Hepatol. 2021;36:1877–88.
Ran H, Tsutsumi R, Araki T, Neel BG. Sticking it to cancer with molecular glue for SHP2. Cancer Cell. 2016;30:194–6.
Nozawa Y, Abe M, Wakasa H, et al. Establishment and characterization of an Epstein-Barr virus negative B-cell lymphoma cell line and successful heterotransplantation. Tohoku J Exp Med. 1988;156:319–30.
Hui L, Chen Y. Tumor microenvironment: sanctuary of the devil. Cancer Lett. 2015;368:7–13.
Cioroianu AI, Stinga PI, Sticlaru L, et al. Tumor microenvironment in diffuse large B-cell lymphoma: role and prognosis. Anal Cell Pathol (Amst). 2019;2019:8586354.
Quail DF, Joyce JA. Microenvironmental regulation of tumor progression and metastasis. Nat Med. 2013;19:1423–37.
Marinaccio C, Ingravallo G, Gaudio F, et al. Microvascular density, CD68 and tryptase expression in human diffuse large B-cell lymphoma. Leuk Res. 2014;38:1374–7.
Cencini E, Fabbri A, Schiattone L, et al. Prognostic impact of tumor-associated macrophages, lymphocyte-to-monocyte and neutrophil-to-lymphocyte ratio in diffuse large B-cell lymphoma. Am J Blood Res. 2020;10:97–108.
Najafi M, Hashemi Goradel N, Farhood B, et al. Macrophage polarity in cancer: a review. J Cell Biochem. 2019;120:2756–65.
Pan Y, Yu Y, Wang X, Zhang T. Tumor-associated macrophages in tumor immunity. Front Immunol. 2020;11:583084.
Dysthe M, Parihar R. Myeloid-derived suppressor cells in the tumor microenvironment. Adv Exp Med Biol. 2020;1224:117–40.
Albini A, Bruno A, Noonan DM, Mortara L. Contribution to tumor angiogenesis from innate immune cells within the tumor microenvironment: implications for immunotherapy. Front Immunol. 2018;9:527.
Khalaf K, Hana D, Chou JT, Singh C, Mackiewicz A, Kaczmarek M. Aspects of the tumor microenvironment involved in immune resistance and drug resistance. Front Immunol. 2021;12:656364.
Song Y, Fu Y, Xie Q, Zhu B, Wang J, Zhang B. Anti-angiogenic agents in combination with immune checkpoint inhibitors: a promising strategy for cancer treatment. Front Immunol. 2020;11:1956.
Autio M, Leivonen SK, Bruck O, et al. Immune cell constitution in the tumor microenvironment predicts the outcome in diffuse large B-cell lymphoma. Haematologica. 2021;106:718–29.
Muris JJ, Meijer CJ, Cillessen SA, et al. Prognostic significance of activated cytotoxic T-lymphocytes in primary nodal diffuse large B-cell lymphomas. Leukemia. 2004;18:589–96.
Zheng W, Qian C, Tang Y, et al. Manipulation of the crosstalk between tumor angiogenesis and immunosuppression in the tumor microenvironment: insight into the combination therapy of anti-angiogenesis and immune checkpoint blockade. Front Immunol. 2022. https://doi.org/10.3389/fimmu.2022.1035323.
Tian L, Goldstein A, Wang H, et al. Mutual regulation of tumour vessel normalization and immunostimulatory reprogramming. Nature. 2017;544:250–4.
Newport EL, Pedrosa AR, Njegic A, Hodivala-Dilke KM, Munoz-Felix JM. Improved immunotherapy efficacy by vascular modulation. Cancers. 2021;13(5207):8906.
Yi M, Jiao D, Qin S, Chu Q, Wu K, Li A. Synergistic effect of immune checkpoint blockade and anti-angiogenesis in cancer treatment. Mol Cancer. 2019;18:60.
Huang Y, Kim BYS, Chan CK, Hahn SM, Weissman IL, Jiang W. Improving immune-vascular crosstalk for cancer immunotherapy. Nat Rev Immunol. 2018;18:195–203.
Khan KA, Kerbel RS. Improving immunotherapy outcomes with anti-angiogenic treatments and vice versa. Nat Rev Clin Oncol. 2018;15:310–24.
Ren S, Xiong X, You H, Shen J, Zhou P. the combination of immune checkpoint blockade and angiogenesis inhibitors in the treatment of advanced non-small cell lung cancer. Front Immunol. 2021;12:689132.
Chambers A, Kundranda M, Rao S, Mahmoud F, Niu J. Anti-angiogenesis revisited: combination with immunotherapy in solid tumors. Curr Oncol Rep. 2021;23:100.
Finn RS, Qin S, Ikeda M, et al. Atezolizumab plus bevacizumab in unresectable hepatocellular carcinoma. N Engl J Med. 2020;382:1894–905.
Motzer R, Alekseev B, Rha SY, et al. Lenvatinib plus pembrolizumab or everolimus for advanced renal cell carcinoma. N Engl J Med. 2021;384:1289–300.
Santini FC, Rudin CM. Atezolizumab for the treatment of non-small cell lung cancer. Expert Rev Clin Pharmacol. 2017;10:935–45.
Qiu L, Zhao X, Shi W, et al. Real-world treatment efficacy of anti-programmed death-1 combined with anti-angiogenesis therapy in non-small cell lung cancer patients. Medicine (Baltimore). 2020;99:e20545.
Socinski MA, Jotte RM, Cappuzzo F, et al. Atezolizumab for first-line treatment of metastatic nonsquamous NSCLC. N Engl J Med. 2018;378:2288–301.
Jiang P, Gu S, Pan D, et al. Signatures of T cell dysfunction and exclusion predict cancer immunotherapy response. Nat Med. 2018;24:1550–8.
Keenan TE, Burke KP, Van Allen EM. Genomic correlates of response to immune checkpoint blockade. Nat Med. 2019;25:389–402.
Funding
The study was supported by National Natural Science Foundation of China (81800203), Doctoral Startup Scientific Research Foundation of Liaoning Province (20180540088) and 1 + X program for clinical competency enhancement–improvement of MDT project, the Second Affiliated Hospital of Dalian Medical University (2022MDTZY02).
Author information
Authors and Affiliations
Contributions
YL and LL performed the data analysis and interpreted the data. NZ collected samples. JW and XS contributed to the experiments of this study. JW prepared the draft. YL and BT performed the visualization and revised the draft. BT, YL and XW designed the research and supervised all the work. All authors contributed to the article and approved the submitted version.
Corresponding authors
Ethics declarations
Conflict of interests
The authors declare that the research was conducted in the absence of any commercial or financial relationships that could be construed as a potential conflict of interest.
Ethical approval
The study was approved by the Ethics Committee of the Second Affiliated Hospital of Dalian Medical University (No.2023–138).
Informed consent
Informed consent was obtained from all subjects involved in the study.
Additional information
Publisher's Note
Springer Nature remains neutral with regard to jurisdictional claims in published maps and institutional affiliations.
Supplementary Information
Below is the link to the electronic supplementary material.

Rights and permissions
Springer Nature or its licensor (e.g. a society or other partner) holds exclusive rights to this article under a publishing agreement with the author(s) or other rightsholder(s); author self-archiving of the accepted manuscript version of this article is solely governed by the terms of such publishing agreement and applicable law.
About this article
Cite this article
Liu, Y., Wang, J., Shen, X. et al. A novel angiogenesis-related scoring model predicts prognosis risk and treatment responsiveness in diffuse large B-cell lymphoma. Clin Exp Med 23, 3781–3797 (2023). https://doi.org/10.1007/s10238-023-01127-9
Received:
Accepted:
Published:
Issue Date:
DOI: https://doi.org/10.1007/s10238-023-01127-9