Abstract
Nitric oxide (NO), as a vital signaling molecule related to different physiological and pathological processes in living systems, is closely associated with cancer and cardiovascular disease. However, the detection of NO in real-time remains a difficulty. Here, PtBi alloy nanoparticles (NPs) were synthesized, dealloyed, and then fabricated to NP-based electrodes for the electrochemical detection of NO. Transmission electron microscopy (TEM), small-angle X-ray scattering (SAXS), and nitrogen physical adsorption/desorption show that dealloyed PtBi alloy nanoparticles (dPtBi NPs) have a porous nanostructure. Electrochemical impedance spectroscopy and cyclic voltammetry results exhibit that the dPtBi NP electrode possesses unique electrocatalytic features such as low charge transfer resistance and large electrochemically active surface area, which lead to its excellent NO electrochemical sensing performance. Owing to the higher density of catalytical active sites formed PtBi bimetallic interface, the dPtBi NP electrode displays superior electrocatalytic activity toward the oxidation of NO with a peak potential at 0.74 V vs. SCE. The dPtBi NP electrode shows a wide dynamic range (0.09–31.5 μM) and a low detection limit of 1 nM (3σ/k) as well as high sensitivity (130 and 36.5 μA μM−1 cm−2). Moreover, the developed dPtBi NP-based electrochemical sensor also exhibited good reproducibility (RSD 5.7%) and repeatability (RSD 3.4%). The electrochemical sensor was successfully used for the sensitive detection of NO produced by live cells. This study indicates a highly effective approach for regulating the composition and nanostructures of metal alloy nanomaterials, which might provide new technical insights for developing high-performance NO-sensitive systems, and have important implications in enabling real-time detection of NO produced by live cells.
Graphical Abstract







Similar content being viewed by others
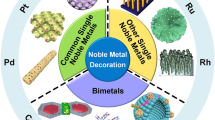
Data availability
Data generated or analyzed during this study are included in this published article and its supplementary file.
References
Lowenstein CJ, Snyder SH (1992) Nitric oxide, a novel biologic messenger. Cell 70(5):705–707. https://doi.org/10.1016/0092-8674(92)90301-R
Calas-Blanchard C, Catanante G, Noguer T (2014) Electrochemical sensor and biosensor strategies for ROS/RNS detection in biological systems. Electroanalysis 26(6):1277–1286. https://doi.org/10.1002/elan.201400083
Palmer RMJ, Ferrige AG, Moncada S (1987) Nitric oxide release accounts for the biological activity of endothelium-derived relaxing factor. Nature 327(6122):524–526. https://doi.org/10.1038/327524a0
Bogdan C (2001) Nitric oxide and the immune response. Nat Immunol 2(10):907–916. https://doi.org/10.1038/ni1001-907
Almeida B, Rogers KE, Nag OK, Delehanty JB (2021) Sensing nitric oxide in cells: historical technologies and future outlook. ACS Sensors 6(5):1695–1703. https://doi.org/10.1021/acssensors.1c00051
Liu Y-L, Qin Y, Jin Z-H, Hu X-B, Chen M-M, Liu R, Amatore C, Huang W-H (2017) A stretchable electrochemical sensor for inducing and monitoring cell mechanotransduction in real time. Angew Chem Int Ed 56(32):9454–9458. https://doi.org/10.1002/anie.201705215
Ignarro LJ, Buga GM, Wood KS, Byrns RE, Chaudhuri G (1987) Endothelium-derived relaxing factor produced and released from artery and vein is nitric oxide. Proc Natl Acad Sci USA 84(24):9265. https://doi.org/10.1073/pnas.84.24.9265
Bryan NS, Bian K, Murad F (2009) Discovery of the nitric oxide signaling pathway and targets for drug development. Front Biosci (Landmark Ed) 14(1):1–18. https://doi.org/10.2741/3228
Rapoport RM, Draznin MB, Murad F (1983) Endothelium-dependent relaxation in rat aorta may be mediated through cyclic GMP-dependent protein phosphorylation. Nature 306(5939):174–176. https://doi.org/10.1038/306174a0
Xu HY, Liao C, Liu YJ, Ye BC, Liu BH (2018) Iron phthalocyanine decorated nitrogen-doped graphene biosensing platform for real-time detection of nitric oxide released from living cells. Anal Chem 90(7):4438–4444. https://doi.org/10.1021/acs.analchem.7b04419
Wang MH, Zhu L, Zhang S, Lou YF, Zhao SR, Tan Q, He LH, Du M (2021) A copper(II) phthalocyanine-based metallo-covalent organic framework decorated with silver nanoparticle for sensitively detecting nitric oxide released from cancer cells. Sensor Actuat B-Chem 338:129826. https://doi.org/10.1016/j.snb.2021.129826
Wenninger N, Bernhart C, Kappaun W, Kollau A, Kalcher K, Ortner A (2023) High-performance amperometric determination of nitric oxide released by endothelial cells using flow injection analysis. Talanta 253:123810. https://doi.org/10.1016/j.talanta.2022.123810
Zhang R, Ye Z, Wang G, Zhang W, Yuan J (2010) Development of a ruthenium(II) complex based luminescent probe for imaging nitric oxide production in living cells. Chem-Eur J 16(23):6884–6891. https://doi.org/10.1002/chem.200903267
Zhang Z, Han X, Wang Z, Yang Z, Zhang W, Li J, Yang H, Ling XY, Xing B (2018) A live bacteria SERS platform for the in situ monitoring of nitric oxide release from a single MRSA. Chem Commun 54(51):7022–7025. https://doi.org/10.1039/C8CC02855A
Nagano T, Yoshimura T (2002) Bioimaging of nitric oxide. Chem Rev 102(4):1235–1270. https://doi.org/10.1021/cr010152s
Jo A, Do H, Jhon G-J, Suh M, Lee Y (2011) Electrochemical nanosensor for real-time direct imaging of nitric oxide in living brain. Anal Chem 83(21):8314–8319. https://doi.org/10.1021/ac202225n
Hong H, Sun J, Cai W (2009) Multimodality imaging of nitric oxide and nitric oxide synthases. Free Radic Biol Med 47(6):684–698. https://doi.org/10.1016/j.freeradbiomed.2009.06.011
Hu FX, Xie LJ, Bao SJ, Yu L, Li CM (2015) Shape-controlled ceria-reduced graphene oxide nanocomposites toward high-sensitive in situ detection of nitric oxide. Biosens Bioelectron 70:310–317. https://doi.org/10.1016/j.bios.2015.03.056
Rojas D, Hernandez-Rodriguez JF, Della Pelle F, Escarpa A, Compagnone D (2022) New trends in enzyme-free electrochemical sensing of ROS/RNS. Application to live cell analysis. Microchim Acta 189(3):102. https://doi.org/10.1007/s00604-022-05185-w
Li J, Jiang MY, Su MJ, Tian L, Shi WS, Yu CM (2021) Stretchable and transparent electrochemical sensor based on nanostructured Au on carbon nanotube networks for real-time analysis of H2O2 release from cells. Anal Chem 93(17):6723–6730. https://doi.org/10.1021/acs.analchem.1c00336
Wang Y, Zhou YT, Chen YJ, Yin ZH, Hao J, Li HM, Liu KP (2022) Simple and sensitive nitric oxide biosensor based on the electrocatalysis of horseradish peroxidase on AuNPs@ metal-organic framework composite-modified electrode. Microchim Acta 189(4):162. https://doi.org/10.1007/s00604-022-05268-8
Dou B, Li J, Jiang B, Yuan R, Xiang Y (2019) DNA-templated in situ synthesis of highly dispersed AuNPs on nitrogen-doped graphene for real-time electrochemical monitoring of nitric oxide released from live cancer cells. Anal Chem 91(3):2273–2278. https://doi.org/10.1021/acs.analchem.8b04863
Jin ZH, Liu YL, Chen JJ, Cai SL, Xu JQ, Huang WH (2017) Conductive polymer-coated carbon nanotubes to construct stretchable and transparent electrochemical sensors. Anal Chem 89(3):2032–2038. https://doi.org/10.1021/acs.analchem.6b04616
Liu P, Nørskov JK (2001) Ligand and ensemble effects in adsorption on alloy surfaces. Phys Chem Chem Phys 3(17):3814–3818. https://doi.org/10.1039/B103525H
Jiang B, Kani K, Iqbal M, Abe H, Kimura T, Hossain MSA, Anjaneyulu O, Henzie J, Yamauchi Y (2018) Mesoporous bimetallic RhCu alloy nanospheres using a sophisticated soft-templating strategy. Chem Mater 30(2):428–435. https://doi.org/10.1021/acs.chemmater.7b04307
Hammer B, Norskov JK (1995) Why gold is the noblest of all the metals. Nature 376(6537):238–240. https://doi.org/10.1038/376238a0
Hammer B, Nørskov JK (1995) Electronic factors determining the reactivity of metal surfaces. Surf Sci 343(3):211–220. https://doi.org/10.1016/0039-6028(96)80007-0
Hammer B, Morikawa Y, Nørskov JK (1996) CO chemisorption at metal surfaces and overlayers. Phys Rev Lett 76(12):2141–2144. https://doi.org/10.1103/PhysRevLett.76.2141
Liu N, Wang X, Wan Y (2015) First principle calculations of nitric oxide on metallic Pt (111) and Pt (100), and bimetallic Au/Pt (111) and Au/Pt (100) surfaces. Appl Surf Sci 328:591–595. https://doi.org/10.1016/j.apsusc.2014.12.079
Liu Z, Forsyth H, Khaper N, Chen A (2016) Sensitive electrochemical detection of nitric oxide based on AuPt and reduced graphene oxide nanocomposites. Analyst 141(13):4074–4083. https://doi.org/10.1039/C6AN00429F
Govindhan M, Chen A (2016) Enhanced electrochemical sensing of nitric oxide using a nanocomposite consisting of platinum-tungsten nanoparticles, reduced graphene oxide and an ionic liquid. Microchim Acta 183(11):2879–2887. https://doi.org/10.1007/s00604-016-1936-y
Ji Y, Wu Y, Zhao G, Wang D, Liu L, He W, Li Y (2015) Porous bimetallic Pt-Fe nanocatalysts for highly efficient hydrogenation of acetone. Nano Res 8(8):2706–2713. https://doi.org/10.1007/s12274-015-0777-z
Fu SF, Zhu CZ, Shi QR, Du D, Lin YH (2016) Enhanced electrocatalytic activities of three dimensional PtCu@Pt bimetallic alloy nanofoams for oxygen reduction reaction. Catal Sci Technol 6(13):5052–5059. https://doi.org/10.1039/c5cy02288f
Kim S, Ha Y, Kim SJ, Lee C, Lee Y (2019) Selectivity enhancement of amperometric nitric oxide detection via shape-controlled electrodeposition of platinum nanostructures. Analyst 144(1):258–264. https://doi.org/10.1039/c8an01518j
McCue I, Benn E, Gaskey B, Erlebacher J (2016) Dealloying and dealloyed materials. Annu Rev Mater Res 46(1):263–286. https://doi.org/10.1146/annurev-matsci-070115-031739
Liu ZG, Nemec-Bakk A, Khaper N, Chen AC (2017) Sensitive electrochemical detection of nitric oxide release from cardiac and cancer cells via a hierarchical nanoporous gold microelectrode. Anal Chem 89(15):8036–8043. https://doi.org/10.1021/acs.analchem.7b01430
Zhang D, Wu F, Peng M, Wang X, Xia D, Guo G (2015) One-step, facile and ultrafast synthesis of phase- and size-controlled Pt–Bi intermetallic nanocatalysts through continuous-flow microfluidics. J Am Chem Soc 137(19):6263–6269. https://doi.org/10.1021/jacs.5b01088
Dmitri IS, Michel HJK (2003) Small-angle scattering studies of biological macromolecules in solution. Rep Prog Phys 66(10):1735. https://doi.org/10.1088/0034-4885/66/10/R05
Wang H, Lin G, Li X, Lu W, Peng Z (2019) Self-standing hollow porous AuPt nanospheres and their enhanced electrocatalytic performance. J Colloid Interface Sci 554:396–403. https://doi.org/10.1016/j.jcis.2019.07.023
Zhu PH, Li SS, Zhou S, Ren N, Ge SG, Zhang Y, Wang YF, Yu JH (2021) In situ grown COFs on 3D strutted graphene aerogel for electrochemical detection of NO released from living cells. Chem Eng J 420:127559. https://doi.org/10.1016/j.cej.2020.127559
Lu J, Do I, Drzal LT, Worden RM, Lee I (2008) Nanometal-decorated exfoliated graphite nanoplatelet based glucose biosensors with high sensitivity and fast response. ACS Nano 2(9):1825–1832. https://doi.org/10.1021/nn800244k
Ganesamurthi J, Shanmugam R, Chen SM, Veerakumar P (2022) Bismuth sulfide/zinc-doped graphitic carbon nitride nanocomposite for electrochemical detection of hazardous nitric oxide. J Electroanal Chem 910:116174. https://doi.org/10.1016/j.jelechem.2022.116174
Ren H, Han Q, Zhang S, Huang Y, Chen Y, Dai H, Yan J, Lin Y (2020) A photothermal assisted in situ signal-amplified electrochemical immunoassay based on multifunctional probe for detecting autoimmune hepatitis marker. Sensors Actuators B Chem 309:127823. https://doi.org/10.1016/j.snb.2020.127823
Asset T, Gommes CJ, Drnec J, Bordet P, Chattot R, Martens I, Nelayah J, Job N, Maillard F, Dubau L (2019) Disentangling the degradation pathways of highly defective PtNi/C nanostructures - an Operando Wide and Small Angle X-ray Scattering Study. ACS Catal 9(1):160–167. https://doi.org/10.1021/acscatal.8b02665
Funding
This study was supported by the following grants: the Beijing Outstanding Young Scientist Program (BJJWZYJH01201910005017) and the National Natural Science Foundation of China (No. 22127805).
Author information
Authors and Affiliations
Contributions
Xiucheng Sun: data curation; validation; investigation; visualization; writing—original draft. Yong Yan: methodology; formal analysis; writing—review and editing. Yacheng Wang: methodology. Yaoyao Zhao: writing—review and editing. Xiangnan Dou: writing—review and editing. Dongtang Zhang: methodology, formal analysis, resources. Liping Lu: conceptualization; methodology; formal analysis; visualization; writing—review and editing. Guangsheng Guo: resources. Xiayan Wang: conceptualization; methodology; funding acquisition; project administration; writing—review and editing; supervision.
Corresponding authors
Ethics declarations
Conflict of interest
The authors declare no competing interests.
Additional information
Publisher's note
Springer Nature remains neutral with regard to jurisdictional claims in published maps and institutional affiliations.
Supplementary Information
Below is the link to the electronic supplementary material.
Rights and permissions
Springer Nature or its licensor (e.g. a society or other partner) holds exclusive rights to this article under a publishing agreement with the author(s) or other rightsholder(s); author self-archiving of the accepted manuscript version of this article is solely governed by the terms of such publishing agreement and applicable law.
About this article
Cite this article
Sun, X., Yan, Y., Wang, Y. et al. Sensitive electrochemical measurement of nitric oxide released from living cells based on dealloyed PtBi alloy nanoparticles. Microchim Acta 190, 277 (2023). https://doi.org/10.1007/s00604-023-05837-5
Received:
Accepted:
Published:
DOI: https://doi.org/10.1007/s00604-023-05837-5