Abstract
A reproductive stage-structured deterministic differential equation model for the population dynamics of the human malaria vector is derived and analysed. The model captures the gonotrophic and behavioural life characteristics of the female Anopheles sp. mosquito and takes into consideration the fact that for the purposes of reproduction, the female Anopheles sp. mosquito must visit and bite humans (or animals) to harvest necessary proteins from blood that it needs for the development of its eggs. Focusing on mosquitoes that feed exclusively on humans, our results indicate the existence of a threshold parameter, the vectorial reproduction number, whose size increases with increasing number of gonotrophic cycles, and is also affected by the female mosquito’s birth rate, its attraction and visitation rate to human residences, and its contact rate with humans. A stability analysis of the model indicates that the mosquito can establish itself in the environment if and only if the value of the vectorial reproduction number exceeds unity and that mosquito eradication is possible if the vectorial reproduction number is less than unity, since, then, the trivial steady state which always exist is unique and is globally and asymptotically stable. When a persistent vector population steady state exists, it is locally and asymptotically stable for a range of reproduction numbers, but can also be driven to instability via a Hopf bifurcation as the reproduction number increases further away from unity. The model derivation identifies and characterizes control parameters relating to activities such as human-mosquito contact and the mosquito’s survival chances between blood meals and egg laying. Our results show that the total mosquito population size increases with increasing number of gonotrophic cycles. Therefore understanding the fundamental aspects of the mosquito’s behaviour provides a pathway for the study of human-mosquito contact and mosquito population control. Control of the mosquito population densities would ultimately lead to malaria control.





Similar content being viewed by others
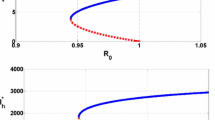
Notes
Females Anopheles can survive for up to a month (or more in captivity) but most probably do not live longer than 2–3 weeks in nature Giles and Warrel (2002).
A Metamorphic cycle starts when the female mosquito lays eggs on water (Eggs are laid every two to three days in batches and each batch can contain over one hundred eggs.). After about 2–3 days, the eggs hatch into larvae which live in a aquatic environment. The larvae eventually mature into pupae in about 4–10 days, and the pupae then change into adult free flying adult mosquitoes in about 2–4 days. The duration of the metamorphic cycle, from egg laying to an adult mosquito eclosion, varies between 7 and 20 days depending on the ambient temperature of the swamp and the species of mosquito involved.
The process of blood feeding, egg maturation and oviposition that is repeated several times during the mosquito’s entire reproductive life is referred to as the gonotrophic cycle. The mosquito’s first batch of eggs may require more than one blood meal to mature but subsequently, blood meals and oviposition alternate regularly.
In the context of malaria transmission, it is the questing vectors that are in at least their second or higher reproductive stage that can transmit infection.
After an unsuccessful interaction with the human, the vector may have a chance to try again, but for now, we have assumed for simplicity that the vector is killed in case of an unsuccessful interaction.
The most general form of the initial condition would take the form \(V_{i}(t_{0}) = V_{i}^{0}\), \(W_{i}(t_{0}) = W_{i}^{0}\) and \(U_{i+1}(t_{0}) = U_{i+1}^{0}\) for \(i\in \{0,1,2,3,\ldots ,N-1\}\), where \(t_{0}\) is some initial starting time and \(V_{i}^{0}\), \(W_{i}^{0}\) and \(U_{i}^{0}\) some prescribed constants. However, for simplicity, and without loss of generality, we have taken \(t_{0}=0\), together with the form (16) for \(V_{i}^{0}\), \(W_{i}^{0}\) and \(U_{i}^{0}\).
Recall that, for this model, all new births go to vectors of type \(V_{0}\).
References
Baton LA, Ranford-Cartwright LC (2005) Spreading the seeds of million-murdering death: metamorphoses of malaria in the mosquito. Trends Parasitol 21(12):573–580
Brännström Å, Sumpter DJT (2005) The role of competition and clustering in population dynamics. Proc R Soc B 272:2065–2072
Castãnera MB, Aparicio JP, Gurtler RE (2003) A stage-structured stochastic model of the population dynamics of Triatoma infestans, main vector of chagas disease. Ecol Model 162:33–53
Charlwood JD, Jones MDR (1979) Mating behaviour in the mosquito Anopheles gambiae s. l. i. Close range and contact behaviour. Physiol Entomol 4(2):111–120
Charlwood JD, Thompson R, Madsen H (2003) Observations on the swarming and mating behaviour of Anopheles funestus from southern Mozambique. Malar J 2(2). http://www.malariajournal.com/content/s/1/2
Chitnis N, Cushing JM, Hyman JM (2006) Bifurcation analysis of a mathematical model for malaria transmission. SIAM J Appl Math 67(1):24–45
Costantino RF, Desharnais RA, Cushing JM, Brian D (1998) Chaotic dynamics in an insect population. Science 275(5298):389–391
Craig GB Jr (1967) Mosquito, female monogamy induced by a male accessory gland substance. Science 156(781):1499–1501
Diekmann O, Heesterbeek JAP, Metz JAJ (1990) On the definition and the computation of the basic reproduction ratio \({R}_0\) in models for infectious diseases in heterogeneous populations. J Math Biol 28:365–382
Eichner Martin (1996) Difference equation model for malaria transmission and disease. Harvard School of Public Health, Boston
Giles HM, Warrel DA (2002) Bruce-Chwatt’s essential malariology, 4th edn. Hodder Arnold Publication, London
Gomulski L (1990) Polyandry in nulliparous Anopheles gambiae mosquitoes (diptera: Culicidae). Bull Entomol Res 80(4):393–396
Hahn W (1967) Stabilty of motion. Springer, New York
Hale HK (1969) Ordinary differential equations. Wiley, New York
Hastings IM, D’Alessandro U (2000) Modelling a predictable disaster: the rise and spread of drug-resistant malaria. Parasitol Today 16:340–347
James Powell A, Logan Jesse A (2005) Insect seasonality: a circle map analysis of the temperature-driven life cycles. Theor Popul Biol 67:161–179
Jang SR-J (2005) Contest and scramble competition with a dynamic resource. Nonlinear Anal 63:109–118
Klowden JM (2001) Sexual receptivity in Anopheles gambiae mosquitoes: absence of controle by male accessory gland substances. J Insect Physiol 47(7):661–666
Klowden Marc J, Briegel Hans (1994) Mosquito gonotrophic cycle and multiple feeding potential: contrast between Anopheles and Aedes (Diptera: Culicidae). J Med Entomol 31(4):618–622
Lutambi Angelina Mageni, Penny Melissa A, Chitnis Nakul, Smith Thomas (2013) Mathematical modelling of mosquito dispersal in a heterogeneous patchy environment. Math Biosci 241(2):198–216
Maynard-Smith J, Slatkin M (1973) The satbility of predator-prey systems. Ecology 54:384–391
McCall PJ, Kelly David W (2002) Learning and memory in disease vectors. Trends Parasitol 18(10):429–433
Nåsell Ingemar (1985) Hybrid models of tropical infections. Lecture notes in biomathematics. Springer, Berlin
Ngonghala CN, Ngwa GA, Teboh-Ewungkem MI (2012) Periodic oscillations and backward bifurcation in a model for the dynamics of malaria transmission. Math Biosci 240(1):45–62
Ngonghala CN, Teboh-Ewungkem MI, Ngwa GA (2014) Persistent oscillations and backward bifurcation in a malaria model with varying human and mosquito populations: implications for control. J Math Biol. doi:10.1007/s00285-014-0804-9
Ngwa GA, Niger AM, Gumel AB (2010) Mathematical assessment of the role of non-linear birth and maturation delay in the population dynamics of the malaria vector. Appl Math Comput 217:3286–3313
Ngwa GA, Shu WS (2000) A mathematical model for endemic malaria with variable human and mosquito populations. Math Comput Model 32(7):747–763
Ngwa Gideon A (2006) On the population dynamics of the malaria vector. Bull Math Biol 68(8):2161–2189
Nourridine Siewe, Teboh-Ewungkem Miranda I, Ngwa Gideon A (2011) A mathematical model of the population dynamics disease-transmitting vectors with spatial consideration. J Biol Dyn 5(4):335–365
Porphyre T, Bicout DJ, Sabatier P (2005) Modelling the abundance of mosquito vectors versus flooding dynamics. Ecol Model 183:173–181
Powel PD (2011) Calculatng determinants of block matrices. arXiv:1112.4379v1 [math.RA], 2011
Raffy M, Tran A (2005) On the dynamics of flying insects populations controlled by large scale information. Theor Popul Biol 68:91–104
Silver John B (1998) Mosquito ecology field sampling methods, 3rd edn. Springer, New York
Silvester John R (2000) Determinants of block matrices. Math Gaz 85(501):460–467
Takken W, Knols GJB (1999) Odor-mediated Behaviour of Afrotropical malaria mosquitoes. Annu Rev Entomol 44:131–157
Teboh-Ewungkem MI, Ngwa GA, Ngonghala CN (2013) Models and proposals for malaria: a review. Math Popul Stud 20(2):57–81
Teboh-Ewungkem MI, Wang M (2012) Male fecundity and optimal gametocyte sex ratios for plasmodium falciparum during incomplete fertilization. J Theor Biol 307:183–192
Teboh-Ewungkem MI, Yuster T (2010) A within-vector mathematical model of plasmodium falciparum and implications of incomplete fertilization on optimal gametocyte sex ratio. J Theor Biol 264(2):273–286
Thomas Moon Edward (1976) A statistical model for the dynamics of a mosquito vector (Culex tarsalis) population. Biometric 32(2):355–368
Tiemi Takahashi Lucy, Maidana Norberto Anibal, Jr Wilson Castro Ferrerira, Pulino Petronio, Yang Hyun Mo (2005) Mathematical models for Aedes aegypti dispersal dynamics: travelling waves by wing and wind. Bull Math Biol 67:509–528
van den Driessche P, Watmough James (2002) Reproduction numbers and sub-threshold endemic equilibria for compartmental models of disease transmission. Math Biosci 180:29–48
Verhulst PF (1838) Notice sur la loi que la population suit dans son acroissement. Corr Math Phys 10:113–121
Villarreal C, Fuentes-Maldonado G, Rodriguez MH, Yuval B (1994) Low rates of multiple fertilization in parous anopheles albimanus. J Am Mosq Control Assoc 10(1):67–69
Wanji S, Mafo SS, Tendongfor N, Tanga MC, Tchuente F, Bilong Bilong CF, Njine T (2009) Spatial distribution, environmental and physicochemical characterization of anopheles breeding sites in the mount cameroon region. J Vector Borne Dis 46:75–80
Yandel SB, Hogg BD (1988) Modelling insect natality using splines. Biometrics 44(2):385–395
Yuval B, Fritz GN (1994) Multiple mating in female mosquitoes-evidence from a field population of anopheles freeborni (diptera: Culicidae). Bull Entomol Res 84(01):137–139
Acknowledgments
GAN and MYF-N acknowledge support of the Cameroon Ministry of Higher Education through the initiative for the modernization of research in Cameroon’s Higher Education granting scheme for 2013. GAN, MIT-E and CNN acknowledge the support of NIMBioS through the investigative workshop grant that supported a workshop on Malaria Modeling and Control, co-organized by MIT-E et al. in 2011. They also acknowledge support of the NSF grant DMS-1261662 that supported a 2013 School on Stochastic Analysis, Financial, and Actuarial Mathematics with Applications, that enabled all five authors to meet. CNN was supported by a Postdoctoral Fellowship from the National Institute for Mathematical and Biological Synthesis, an Institute sponsored by the National Science Foundation, the U.S. Department of Homeland Security, and the U.S. Department of Agriculture through NSF Award #EF-0832858, with additional support from The University of Tennessee, Knoxville. TTW acknowledges AMMSI through the Department of Mathematics at the University of Buea for support towards his research. All authors acknowledge the constructive comments of the reviewers which greatly improved the clarity of our results.
Author information
Authors and Affiliations
Corresponding author
Rights and permissions
About this article
Cite this article
Ngwa, G.A., Wankah, T.T., Fomboh-Nforba, M.Y. et al. On a Reproductive Stage-Structured Model for the Population Dynamics of the Malaria Vector. Bull Math Biol 76, 2476–2516 (2014). https://doi.org/10.1007/s11538-014-0021-0
Received:
Accepted:
Published:
Issue Date:
DOI: https://doi.org/10.1007/s11538-014-0021-0