Abstract
Determination of optimum conditions for waste oil (used motor oils, transformer oils) regeneration using vacuum distillation was attempted including the search for the optimum temperature difference between the heating surface and the boiling point of the liquid. Optimum temperature allowed the distillation equipment to operate at maximum performance. Equipment suitable for the measurement of boiling curves under atmospheric pressure and vacuum conditions was assembled. These curves were used to determine the optimum temperature difference of various substances including waste oils. Properties of pure substances, for example water, ethanol, hexane, heptane, isooctane, decane, or dodecane, were measured with this equipment under atmospheric pressure. Results of various liquids measurements under vacuum and atmospheric pressure are presented.
Similar content being viewed by others
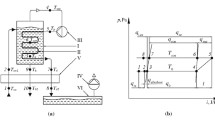
References
Addoms, J. N. (1948). Heat transfer at high rates to water boiling outside cylinders. D.Sc. Thesis, Massachusetts Institute of Technology, Cambridge, MA, USA.
Auracher, H., & Buchholz, M. (2005). Experiments on the fundamental mechanisms of boiling heat transfer. Journal of the Brazilian Society of Mechanical Sciences and Engineering, 27, 1–22. DOI: 10.1590/s1678-58782005000100001.
Berenson, P. J. (1962). Experiments on pool-boiling heat transfer. International Journal of Heat and Mass Transfer, 5, 985–999. DOI: 10.1016/0017-9310(62)90079-0.
Bernardin, J. D., & Mudawar, I. (1999). The Leidenfrost point: Experimental study and assessment of existing models. Journal of Heat Transfer, 121, 894–903. DOI: 10.1115/1.2826080.
Bonilla, C. F., Grady, J. J., & Avery, G. W. (1965). Pool boiling heat transfer from scored surfaces. Chemical Engineering Progress Symposium Series, 61(57), 280–288.
Chai, L. H., & Shoji, M. (2001). Boiling curves - bifurcation and catastrophe. International Journal of Heat and Mass Transfer, 44, 4175–4179. DOI: 10.1016/s0017-9310(01)00059-x.
Cichelli, M. T., & Bonilla, C. F. (1945). Heat transfer to liquids boiling under pressure. Transactions of AIChE, 41, 755–787.
Clark, H. B., Strenge, P. S., & Westwater, J. W. (1959). Active sites of nucleate boiling. Chemical Engineering Progress Symposium Series, 55(29), 103–110.
Cooke, D., & Kandlikar, S. G. (2011). Pool boiling heat transfer and bubble dynamics over plain and enhanced microchannels. Journal of Heat Transfer, 133(5), 052902. DOI: 10.1115/1.4003046.
Cryder, D. S., & Finalbargo, A. C. (1937). Heat transmission from metal surfaces to boiling liquids: Effect of temperature of liquid on liquid film coefficient. Transactions of AIChE, 50, 346–362.
Farber, E. A., & Scorah, E. L. (1948). Heat transfer to water boiling under pressure. Transactions of the ASME, 70, 369–384.
Griffith, P., & Wallis, J. D. (1960). The role of surface conditions in nucleate boiling. Chemical Engineering Progress Symposium Series, 56, 49–63.
Hsu, S. T., & Schmidt, F. W. (1961). Measured variations in local surface temperatures in pool boiling of water. Journal of Heat Transfer, 83, 254–260. DOI: 10.1115/1.3682252.
Kim, S. H., Ahn, S. H., Kim, J. W., Kaviany, M., & Kim, H. M. (2013). Dynamics of water droplet on a heated nanotubes surface. Applied Physics Letters, 102(23), 233901. DOI: 10.1063/1.4809944.
Kurihara, H. M., & Myers, J. E. (1960). The effects of superheat and surface roughness on boiling coefficients. AIChE Journal, 6, 83–86. DOI: 10.1002/aic.690060117.
Lee, Y., Pioro, I., & Park, H. J. (1994). An experimental study on a plate type two-phase closed thermosyphon. In Y. Kobayashi, & K. Oshima (Eds.), Proceedings of the 4th International Heat Pipe Symposium, May 16–18, 1994. Tsukuba, Japan: Japan Association for Heat Pipes.
Misale, M., Guglielmini, G., & Priarone, A. (2011). Nucleate boiling and critical heat flux of HFE-7100 in horizontal narrow spaces. Experimental Thermal and Fluid Science, 35, 772–779. DOI: 10.1016/j.expthermflusci.2010.06.009.
Nakoryakov, V. E., Misyura, S. Ya., & Elistratov, S. L. (2012). The behavior of water droplets on the heated surface. International Journal of Heat and Mass Transfer, 55, 6609–6617. DOI: 10.1016/j.ijheatmasstransfer.2012.06.069.
Nukiyama, S. (1934). Maximum and minimum values of heat Q transmitted from metal to boiling water under atmospheric pressure. Journal of Japanese Society of Mechanical Engineering, 37, 367–374. (in Japanese)
Pioro, I. L., Park, H. J., & Lee, Y. (1996). Heat transfer in a two-phase closed thermosyphon: Horizontal flat plate type. In Proceedings of the 5th International Symposium on Thermal Engineering and Science for Cold Regions, May 19–22, 1996 (pp. 489–494). Ottawa, Canada.
Pioro, I. (1997). Boiling heat transfer characteristics of thin liquid layers in a horizontally flat two-phase thermosyphon. In Proceedings of the 10th International Heat Pipe Conference, September 21–25, 1997 (Paper H1-5). Stuttgart, Germany.
Pioro, L. S., & Pioro, I. L. (1997). Industrial two-phase thermosyphons (Chapters 2 and 3). New York, NY, USA: Begell House.
Rops, C. M., Lindken, R. H., Velthuis, J. F. M., & Westerweel, J. (2009). Enhanced heat transfer in confined pool boiling. International Journal of Heat and Fluid Flow, 30, 751–760. DOI: 10.1016/j.ijheatfluidflow.2009.03.007.
Tolubinskiy, V. I. (1980). Heat transfer under boiling (Chapters 4 and 5). Kiev, URSS: Naukova Dumka. (in Russian)
Tong, L. S., & Tang, Y. S. (1997). Boiling heat transfer and two-phase flow (2nd ed.). Washington, DC, USA: Taylor & Francis.
Wang, X. F., Rahman, Md. A., Jacobi, A. M., & Hrnjak, P. S. (2013). Dynamic wetting behavior and water drops on microgrooved surfaces. Heat Transfer Engineering, 34, 1088–1098. DOI: 10.1080/01457632.2013.763544.
Yang, L., & Shivpuri, R. (2007). A water evaporation based model for lubricant dryoff on die surfaces heated beyond the Leidenfrost point. Journal of Manufacturing Science and Engineering, 129, 717–725. DOI: 10.1115/1.2738126.
Young, R. K., & Hammel, R. L. (1965). Higher coefficients for heat transfer with nucleate boiling. Chemical Engineering Progress Symposium Series, 61(59), 264–270.
Author information
Authors and Affiliations
Corresponding author
Additional information
Dedicated to the memory of professor Elemír Kossaczký
Rights and permissions
About this article
Cite this article
Nemsilová, L., Timár, P., Timár, P. et al. Measurement of critical heat flux conditions under vacuum. Chem. Pap. 68, 1767–1773 (2014). https://doi.org/10.2478/s11696-014-0621-z
Received:
Revised:
Accepted:
Published:
Issue Date:
DOI: https://doi.org/10.2478/s11696-014-0621-z