Abstract
An integral treatment is proposed for the analysis of the forced convection flow of a nanofluid over a stretching sheet. The obtained results agree well with the numerical results. The results of the presented solution provide an analytic solution, which can be conveniently used in engineering applications. Four types of nanoparticles, i.e., alumina (Al2O3), silicon dioxide (SiO2), silver (Ag), and copper (Cu), dispersed in the base fluid of water are examined. The analytical results show that an increase in the volume fraction of nanoparticles increases the thickness of the thermal boundary layer. The reduced Nusselt number is a decreasing function of the volume fraction of nanoparticles.
Similar content being viewed by others
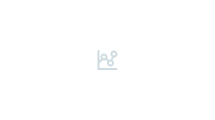
Abbreviations
- (ρc)f :
-
heat capacity of the fluid
- (ρc)p :
-
effective heat capacity of the nanoparticle material
- (ρc p )nf :
-
heat capacity of the nanofluid
- ∞ :
-
condition at infinity
- D B :
-
Brownian diffusion coefficient
- d p :
-
nanoparticle diameter
- D T :
-
thermophoretic diffusion coefficient
- g :
-
gravitational acceleration vector
- h :
-
local heat transfer coefficient
- h m :
-
local mass transfer coefficient
- h nf :
-
heat transfer coefficient of the nanofluid
- K :
-
thermal conductivity
- K B :
-
Boltzmann constant
- k f :
-
thermal conductivity of the fluid
- k nf :
-
nanofluid thermal conductivity
- k p :
-
nanoparticle thermal conductivity
- Le :
-
Lewis number
- N B :
-
Brownian motion parameter
- N T :
-
thermophoresis parameter
- Nu :
-
Nusselt number
- P :
-
pressure
- Pr :
-
Prandtl number
- Re x :
-
local Reynolds number
- Sh x :
-
local Sherwood number
- T :
-
temperature
- T ∞ :
-
ambient temperature attained as y tends to infinity
- T w :
-
temperature at the stretching surface
- u, v :
-
velocitycomponents
- u w :
-
velocity of the stretching sheet
- w:
-
condition at the stretching surface
- x, y :
-
Cartesian coordinates
- α :
-
thermal diffusivity
- β :
-
volumetric expansion coefficient of the fluid
- Δ:
-
boundary-layer thickness ratio
- δ c :
-
concentration boundary-layer thickness
- δ T :
-
thermal boundary-layer thickness
- τ :
-
heat capacity ration (ρc)p/(ρc)f
- θ(η):
-
dimensionless temperature profile
- μ f :
-
viscosity of the fluid
- μ nf :
-
viscosity of the nanofluid
- ρ f :
-
fluid density
- ρ nf :
-
nanofluid density
- ρ p :
-
nanoparticle mass density
- υ :
-
kinematic viscosity of the fluid
- φ :
-
nanoparticle volume fraction
- φ(η):
-
dimensionless concentration profile
- φ ∞ :
-
ambient concentration attained as y tends to infinity
- φ w :
-
ambient nanoparticle volume fraction
References
Altan, T., Oh, S. I., and Gegel, H. L. Metal Forming: Fundamentals and Applications, ASM International, Ohio (1979)
Fisher, E. G. Extrusion of Plastics, Wiley, New York (1976)
Karwe, M. V. and Jaluria, Y. Numerical simulation of thermal transport associated with a continuous moving flat sheet in materials processing. Journal of Heat Transfer, 119, 612–619 (1991)
Choi, S. U. S. Enhancing thermal conductivity of fluids with nanoparticle. Developments and Applications of Non-Newtonian Flows, 231, 99–105 (1995)
Eastman, J. A., Choi, S. U. S., Li, S., Yu, W., and Thompson, L. J. Anomalously increased effective thermal conductivities containing copper nanoparticles. Applied Physics Letters, 78, 718–720 (2001)
Choi, S. U. S., Zhang, Z. G., Yu, W., Lockwood, F. E., and Grulke, E. A. Anomalous thermal conductivity enhancement on nanotube suspension. Applied Physics Letters, 79, 2252–2254 (2001)
Sun, C. Z., Bai, B. F., Lu, W. Q., and Liu, J. Shear-rate dependent effective thermal conductivity of H2O+SiO2 nanofluid. Physics of Fluids, 25, 052002 (2013)
Sakiadis, B. C. Boundary-layer behavior on a continuous solid surface: II, the boundary layer on a continuous flat surface. AIChE Journal, 7, 221–225 (1961)
Crane, L. J. Flow past a stretching plate. Zeitschrift für Angewandte Mathematik und Physik, 21, 645–647 (1970)
Tsou, F. K., Sparrow, E. M., and Goldstein, R. J. Flow and heat transfer in the boundary layer in the continuous moving surfaces. International Journal of Heat and Mass Transfer, 10, 219–235 (1967)
Magyari, E. and Keller, B. Heat and mass transfer in the boundary layers on an exponentially stretching continuous surface. Journal of Physics D: Applied Physics, 32, 577–585 (1999)
Magyari, E. and Keller, B. Heat transfer characteristics of the separation boundary flow induced by a continuous stretching surface. Journal of Physics D: Applied Physics, 32, 2876–2881 (1999)
Wang, C. Y. Free convection on a vertical stretching surface. Journal of Applied Mathematics and Mechanics, 69, 418–420 (1989)
Gorla, R. S. R. and Sidawi, I. Free convection on a vertical stretching surface with suction and blowing. Applied Scientific Research, 52, 247–257 (1994)
Khan, W. A. and Pop, I. Boundary-layer flow of a nanofluid past a stretching sheet. International Journal of Heat and Mass Transfer, 53, 2477–2483 (2010)
Makinde, O. D. and Aziz, A. Boundary-layer flow of a nanofluids past a stretching sheet. International Journal of Thermal Sciences, 50, 1326–1332 (2011)
Rana, P. and Bhargava, R. Flow and heat transfer of a nanofluids over nonlinearly stretching sheet: a numerical study. Communications in Nonlinear Science and Numerical Simulation, 17, 212–226 (2012)
Noghrehabadi, A. R., Pourrajab, R., and Ghalambaz, M. Effect of partial slip boundary condition on the flow and heat transfer of nanofluids past stretching sheet prescribed constant wall temperature. International Journal of Thermal Sciences, 54, 253–261 (2012)
Noghrehabadi, A. R., Ghalambaz, M., and Ghanbarzadeh, A. Heat transfer of magnetohydrodynamic viscous nanofluids over an isothermal stretching sheet. Journal of Thermophysics and Heat Transfer, 26, 686–689 (2012)
Noghrehabadi, A. R., Saffarian, M. R., Pourrajab, R., and Ghalambaz, M. Entropy analysis for nanofluid flow over a stretching sheet in the presence of heat generation/absorption and partial slip. Journal of Mechanical Science and Technology, 27, 927–937 (2013)
Noghrehabadi, A. R., Pourrajab, R., and Ghalambaz, M. Flow and heat transfer of nanofluids over stretching sheet taking into account partial slip and thermal convective boundary conditions. Heat and Mass Transfer, 49, 1357–1366 (2013)
Bejan, A. Convection Heat Transfer, Wiley, New York (2004)
Oztop, H. F. and Abu-Nada, E. Numerical study of natural convection in partially heated rectangular enclosures filled with nanofluids. International Journal of Heat and Fluid Flow, 29, 1326–1336 (2008)
Kefayati, G. H., Hosseinzadeh, S. F., Gorji-Bandpy, M., and Sajjadi, H. Lattice Boltzman solution of natural convection in tall enclosures using water/SiO2 nanofluid. International Communications in Heat and Mass Transfer, 38, 798–805 (2011)
Buongiorno, J. Convective transport in nanofluids. Journal of Heat Transfer, 128, 240–250 (2006)
Brinkman, H. C. The viscosity of concentrated suspensions and solutions. Journal of Chemical Physics, 20, 571–581 (1952)
Khanafer, K. and Vafai, K. A critical synthesis of thermophysical characteristics of nanofluids. International Journal of Heat and Mass Transfer, 54, 4410–4428 (2011)
Yacob, N. A., Ishak, A., Pop, I., and Vajravelu, K. Boundary layer flow past a stretching/shrinking surface beneath an external uniform shear flow with a convective surface boundary condition in a nanofluids. Nanoscale Research Letters, 6, 314–321 (2011)
Maxwell, J. C. A. Treatise on Electricity and Magnetism, 2nd ed., Clarendon Press, Oxford (1881)
Buongiorno, J., Venerus, D. C., Prabhat, N., McKrell, T., Townsend, J., Christianson, R., and Leong, K. C. A benchmark study on the thermal conductivity of nanofluids. Journal of Applied Physics, 106, 094312 (2009)
Author information
Authors and Affiliations
Corresponding author
Rights and permissions
About this article
Cite this article
Noghrehabadi, A., Salamat, P. & Ghalambaz, M. Integral treatment for forced convection heat and mass transfer of nanofluids over linear stretching sheet. Appl. Math. Mech.-Engl. Ed. 36, 337–352 (2015). https://doi.org/10.1007/s10483-015-1919-6
Received:
Revised:
Published:
Issue Date:
DOI: https://doi.org/10.1007/s10483-015-1919-6