Abstract
Functional electrical stimulation has been widely used for the restoration of bladder functions after spinal cord injury or other neurological disorders. However, most of the neuroprostheses for bladder control are still imperfect due to lack of the feedback information about the state of the controlled bladder. The purpose of this study is to develop an implantable system which allows us to stimulate the nerves and record the nerve signals related to the condition of the bladder. The proposed stimulator consists of three parts: a digital-to-analog converter (DAC), a current driver, and a switch network. Using the same current source with a switch network eliminates the need for separate current sources for anodic and cathodic sections and reduce the need for interconnect lines of control signals which is an area-saved and power-efficient configuration. A symmetrical regulated cascode current driver is used to implement a high voltage compliance and a high output impedance which improves its ability with load. The amplitude, frequency and the pulse width of the stimulating current are adjusted by encoding the DAC and switch sequences, respectively. In addition, we also present two-stage fully differential capacitively-coupled amplifiers for neural recording. The neural amplifier’s parameters are carefully chosen according to the characteristics of neural signal; meanwhile, we analyzed theoretically the main noise sources, especially the pseudo-resistor in the feedback path which gives little attention by previous studies. The integrated neural stimulating and recording frontend for bladder control prosthesis has been designed and simulated, using a TSMC’s 0.18-μm CMOS process. The proposed stimulator can provide a symmetrical cathodic-first biphasic current pulse with interphasic gap, a low headroom voltage of 0.168 V corresponding to 2.48 mA full-scale current, an adjustable pulse width of 100–500 μs and frequency of 1–40 Hz. The recording amplifier with a low input-referred noise of 3.62 μV, an NEF of 3.88 and a low power dissipation of 7.2 μW has a gain of 61.6 dB and a frequency bandwidth from 300 Hz to 5.3 kHz. Both circuit analysis and simulations are presented to examine the performance of the proposed designs.















Similar content being viewed by others
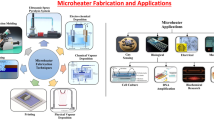
References
Lee, J. W., Kim, D., Yoo, S., Lee, H., Lee, G.-H., & Nam, Y. (2015). Emerging neural stimulation technologies for bladder dysfunctions. International Neurourology Journal, 19(1), 3–11.
Fowler, C. J., Griffiths, D., & de Groat, W. C. (2008). The neural control of micturition. Nature Reviews Neuroscience, 9(6), 453–466.
Rijkhoff, N., Wijkstra, H., Van Kerrebroeck, P., & Debruyne, F. (1997). Urinary bladder control by electrical stimulation: Review of electrical stimulation techniques in spinal cord injury. Neurourology and Urodynamics, 16(1), 39–53.
Wyndaele, J. J., Brauner, A., Geerlings, S. E., Bela, K., Peter, T., & Bjerklund-Johanson, T. E. (2012). Clean intermittent catheterization and urinary tract infection: Review and guide for future research. BJU International, 110(11c), E910–E917.
Schwantes, U., Grosse, J., & Wiedemann, A. (2015). Refractory overactive bladder: A common problem? International Urogynecology Journal, 26(10), 1407–1414.
Stein, R., Schröder, A., & Thüroff, J. W. (2012). Bladder augmentation and urinary diversion in patients with neurogenic bladder: Surgical considerations. Journal of Pediatric Urology, 8(2), 153–161.
Granger, N., Chew, D., Fairhurst, P., Fawcett, J., Lacour, S., Craggs, M., et al. (2013). Use of an implanted sacral nerve stimulator to restore urine voiding in chronically paraplegic dogs. Journal of Veterinary Internal Medicine, 27(1), 99–105.
Kirkham, A., Knight, S., Craggs, M., Casey, A., & Shah, P. (2002). Neuromodulation through sacral nerve roots 2 to 4 with a Finetech-Brindley sacral posterior and anterior root stimulator. Spinal Cord, 40(6), 272–281.
Yoo, P. B., Klein, S. M., Grafstein, N. H., Horvath, E. E., Amundsen, C. L., Webster, G. D., et al. (2007). Pudendal nerve stimulation evokes reflex bladder contractions in persons with chronic spinal cord injury. Neurourology and Urodynamics, 26(7), 1020–1023.
Bhadra, N., Grünewald, V., Creasey, G. H., & Mortimer, J. T. (2006). Selective activation of the sacral anterior roots for induction of bladder voiding. Neurourology and Urodynamics, 25(2), 185–193.
Boger, A. S., Bhadra, N., & Gustafson, K. J. (2012). High frequency sacral root nerve block allows bladder voiding. Neurourology and Urodynamics, 31(5), 677–682.
Lombardi, G., & Del Popolo, G. (2009). Clinical outcome of sacral neuromodulation in incomplete spinal cord injured patients suffering from neurogenic lower urinary tract symptoms. Spinal Cord, 47(6), 486–491.
Choudhary, M., Mastrigt, R., & Asselt, E. (2015). Effect of tibial nerve stimulation on bladder afferent nerve activity in a rat detrusor overactivity model. International Journal of Urology, 23(3), 253–258.
McGee, M. J., & Grill, W. M. (2014). Selective co-stimulation of pudendal afferents enhances bladder activation and improves voiding efficiency. Neurourology and Urodynamics, 33(8), 1272–1278.
Boger, A., Bhadra, N., & Gustafson, K. J. (2008). Bladder voiding by combined high frequency electrical pudendal nerve block and sacral root stimulation. Neurourology and Urodynamics, 27(5), 435–439.
Noblett, K. L., & Cadish, L. A. (2014). Sacral nerve stimulation for the treatment of refractory voiding and bowel dysfunction. American Journal of Obstetrics and Gynecology, 210(2), 99–106.
Spinelli, M. (2007). The future of the sacral nerve stimulation. Pelviperineology, 26, 17–18.
Bruns, T., Gaunt, R., & Weber, D. (2012). Selective recording and stimulation with a sacral dorsal root ganglia neural interface. In International Functional Electrical Stimulation Society Annual Meeting (pp. 9–12).
Ren, J., Chew, D. J., Biers, S., & Thiruchelvam, N. (2015). Electrical nerve stimulation to promote micturition in spinal cord injury patients: A review of current attempts. Neurourology and Urodynamics, 35(3), 365–370.
Wei, X., Rijkhoff, N. J., Santa, W. A., Anderson, J. A., Afshar, P., Schindeldecker, W. J., et al. Functional electrical stimulation as a neuroprosthetic methodology for enabling closed-loop urinary incontinence treatment. In 2011 5th international IEEE/EMBS conference on neural engineering (NER), 2011 (pp. 650–654).
Horvath, E. E., Yoo, P. B., Amundsen, C. L., Webster, G. D., & Grill, W. M. (2010). Conditional and continuous electrical stimulation increase cystometric capacity in persons with spinal cord injury. Neurourology and Urodynamics, 29(3), 401–407.
Wark, H. A. C., Black, S. R., Mathews, K. S., Cartwright, P. C., Gustafson, K. J., & Normann, R. A. (2015). Restoration from acute urinary dysfunction using utah electrode arrays implanted into the feline pudendal nerve. Neuromodulation: Technology at the Neural Interface, 18(4), 317–323.
Bruns, T. M., Weber, D. J., & Gaunt, R. A. (2015). Microstimulation of afferents in the sacral dorsal root ganglia can evoke reflex bladder activity. Neurourology and Urodynamics, 34(1), 65–71.
Uranga, A., Barniol, N., Marin, D., Villa, R., & Aguilo, J. (2002). An integrated implantable electrical sacral root stimulator for bladder control. Neuromodulation: Technology at the Neural Interface, 5(4), 238–247.
Mounaim, F., & Sawan, M. (2007). Miniature implantable system dedicated to bi-channel selective neurostimulation. In IEEE International Symposium on Circuits and Systems, 2007. ISCAS 2007 (pp. 2072–2075).
Sawan, M., Ba, A., Mounaim, F., Corcos, J., & Elhilali, M. (2008). Biomedical circuits and systems dedicated for sensing and neurostimulation: Case study on urinary bladder dysfunctions. Turkish Journal of Electrical Engineering & Computer Sciences, 16(3), 171–187.
Fjorback, M. V., Hansen, J., Dalmose, A., Rijkhoff, N., & Sinkjær, T. (2003). A portable device for experimental treatment of neurogenic detrusor overactivity. Neuromodulation: Technology at the Neural Interface, 6(3), 158–165.
Li, Y.-T., Chen, J.-J. J., Chen, L.-T., Lin, W.-S., & Chu, C.-H. (2012). Wireless implantable biomicrosystem for bladder pressure monitoring and nerve stimulation. In IEEE Biomedical Circuits and Systems Conference (BioCAS), 2012 (pp. 296–299).
Huang, C.-Y., Lee, S.-Y., Hong, J.-H., Liang, M.-C., & Hsieh, C.-H. (2012). Burst-pulse control of microstimulator for bladder controller. In IEEE Asia Pacific Conference on Circuits and Systems (APCCAS), 2012, (pp. 84–87).
Lin, Y.-T., Lai, C.-H., Kuo, T.-S., Chen, C.-C., Chen, Y.-L., Young, S.-T., et al. (2014). Dual-channel neuromodulation of pudendal nerve with closed-loop control strategy to improve bladder functions. Journal of Medical and Biological Engineering, 34(34), 82–89.
Melgaard, J., & Rijkhoff, N. J. (2014). Detecting urinary bladder contractions: Methods and devices. Journal of Sensor Technology, 4(04), 165.
Majerus, S. J., Fletter, P. C., Damaser, M. S., & Garverick, S. L. (2011). Low-power wireless micromanometer system for acute and chronic bladder-pressure monitoring. IEEE Transactions on Biomedical Engineering, 58(3), 763–767.
Tan, R., McClure, T., Lin, C., Jea, D., Dabiri, F., Massey, T., et al. (2009). Development of a fully implantable wireless pressure monitoring system. Biomedical Microdevices, 11(1), 259–264.
Hansen, J., Borau, A., Rodríguez, A., Vidal, J., Sinkjær, T., & Rijkhoff, N. J. (2007). Urethral sphincter EMG as event detector for Neurogenic detrusor overactivity. IEEE Transactions on Biomedical Engineering, 54(7), 1212–1219.
Wenzel, B. J., Boggs, J. W., Gustafson, K. J., Creasey, G. H., & Grill, W. M. (2006). Detection of neurogenic detrusor contractions from the activity of the external anal sphincter in cat and human. Neurourology and Urodynamics, 25(2), 140–147.
Wenzel, B. J., Boggs, J. W., Gustafson, K. J., & Grill, W. M. (2005). Detecting the onset of hyper-reflexive bladder contractions from the electrical activity of the pudendal nerve. IEEE Transactions on Neural Systems and Rehabilitation Engineering, 13(3), 428–435.
Kurstjens, G., Borau, A., Rodriguez, A., Rijkhoff, N., & Sinkjær, T. (2005). Intraoperative recording of electroneurographic signals from cuff electrodes on extradural sacral roots in spinal cord injured patients. The Journal of Urology, 174(4), 1482–1487.
Jezernik, S., Grill, W. M., & Sinkjaer, T. (2001). Detection and inhibition of hyperreflexia-like bladder contractions in the cat by sacral nerve root recording and electrical stimulation. Neurourology and Urodynamics, 20(2), 215–230.
Chew, D. J., Zhu, L., Delivopoulos, E., Minev, I. R., Musick, K. M., Mosse, C. A., et al. (2013). A microchannel neuroprosthesis for bladder control after spinal cord injury in rat. Science Translational Medicine, 5(210), 210ra155-210ra155.
Williams, I., & Constandinou, T. G. (2013). An energy-efficient, dynamic voltage scaling neural stimulator for a proprioceptive prosthesis. IEEE Transactions on Biomedical Circuits and Systems, 7(2), 129–139.
Thurgood, B. K., Warren, D. J., Ledbetter, N. M., Clark, G. A., & Harrison, R. R. (2009). A wireless integrated circuit for 100-channel charge-balanced neural stimulation. IEEE Transactions on Biomedical Circuits and Systems, 3(6), 405–414.
Ortmanns, M., Rocke, A., Gehrke, M., & Tiedtke, H.-J. (2007). A 232-channel epiretinal stimulator ASIC. IEEE Journal of Solid-State Circuits, 42(12), 2946–2959.
Constandinou, T. G., Georgiou, J., & Toumazou, C. (2008). A partial-current-steering biphasic stimulation driver for vestibular prostheses. IEEE Transactions on Biomedical Circuits and Systems, 2(2), 106–113.
Merrill, D. R., Bikson, M., & Jefferys, J. G. (2005). Electrical stimulation of excitable tissue: design of efficacious and safe protocols. Journal of Neuroscience Methods, 141(2), 171–198.
Liu, X., Demosthenous, A., & Donaldson, N. (2008). An integrated implantable stimulator that is fail-safe without off-chip blocking-capacitors. IEEE Transactions on Biomedical Circuits and Systems, 2(3), 231–244.
Noorsal, E., Sooksood, K., Xu, H., Hornig, R., Becker, J., & Ortmanns, M. (2012). A neural stimulator frontend with high-voltage compliance and programmable pulse shape for epiretinal implants. IEEE Journal of Solid-State Circuits, 47(1), 244–256.
Loi, D., Carboni, C., Angius, G., Angotzi, G. N., Barbaro, M., Raffo, L., et al. (2011). Peripheral neural activity recording and stimulation system. IEEE Transactions on Biomedical Circuits and Systems, 5(4), 368–379.
Mendez, A., Sawan, M., Minagawa, T., & Wyndaele, J.-J. (2013). Estimation of bladder volume from afferent neural activity. IEEE Transactions on Neural Systems and Rehabilitation Engineering, 21(5), 704–715.
Lee, J., Kang, J., Park, S., Seo, J.-S., Anders, J., Guilherme, J., et al. (2009). A 2.5 mW 80 dB DR 36 dB SNDR 22 MS/s logarithmic pipeline ADC. IEEE Journal of Solid-State Circuits, 44(10), 2755–2765.
Behzad, R. (2001). Design of analog CMOS integrated circuits. International Edition.
Steyaert, M., Sansen, W., & Chang, Z. (1987). A micropower, low noise monolithic instrumentation amplifier for medical purpose. IEEE Journal of Solid State Circuits, 22, 1163–1168.
Acknowledgements
This work was supported by National Natural Science Foundations of China (90307013, 90707005, 61534003, 61401097), Key Program of Jiangsu Province (BE2016738), Science and Technology Pillar Program of Jiangsu Province (BE2013706) and Fundamental Research Funds for the Central Universities (2242016K40100).
Author information
Authors and Affiliations
Corresponding authors
Ethics declarations
Conflict of interest
The authors declare no conflict of interest.
Rights and permissions
About this article
Cite this article
Tao, W., Li, F., Wang, C. et al. Design of integrated neural stimulating and recording frontend for bladder control prosthesis. Analog Integr Circ Sig Process 91, 403–416 (2017). https://doi.org/10.1007/s10470-017-0962-y
Received:
Revised:
Accepted:
Published:
Issue Date:
DOI: https://doi.org/10.1007/s10470-017-0962-y