Abstract
Objective
We evaluated whether Pseudomonas aeruginosa associated nosocomial infections in our ICU originate mainly from patients' endogenous flora or from exogenous cross-transmission.
Design and setting
A 6-month prospective surveillance survey was performed according to standardized protocols at the interdisciplinary ICU of the Azienda Ospedaliera Cannizzaro.
Patients
The study analyzed 121 patients and focused on three different states: carriage upon admission, colonization of sterile sites, and infections during ICU stay.
Results
We identified 138 P. aeruginosa isolates from 45 patients. The cumulative incidence of P. aeruginosa sustained colonization in the ICU was 29.9/100 patients, and the incidence density was 16.2/1,000 patient-days. The cumulative incidence of P. aeruginosa-sustained infections in the ICU was 36.7/100 patients, and the incidence density was 19.9/1,000 patient-days. The most frequent infection type was ventilator-associated pneumonia. PFGE analysis of P. aeruginosa isolates led to the identification of a major clone represented by 60.8% of isolates involving 45.9% of patients. The impact of cross-transmission, i.e., the preventable proportion of P. aeruginosa acquisition, was estimated to be at least 59.5% of all colonization or infection episodes. Acquisition of multidrug-resistant P. aeruginosa was significantly associated with cross-transmission.
Conclusions
Our results suggest that the ICU personnel and environment served as reservoirs for cross-transmission and emphasize the importance of exogenous acquisition of multidrug-resistant P. aeruginosa, of reduction in antibiotic pressure, and prompt enforcement of infection control measures.
Similar content being viewed by others
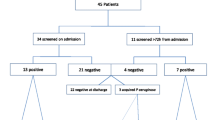
Introduction
Intensive care units (ICUs) worldwide are encountering the highest density of nosocomial infections (NI) and the spread of antibiotic-resistant pathogens responsible for emerging infection problems in the hospital [1–3]. In the rank order of pathogens causing ICU-related infections Pseudomonas aeruginosa has held a nearly unchanged position over recent decades [4]. A European survey showed that P. aeruginosa is one of the most frequent pathogens isolated from ICU-acquired infections [5, 6], and data derived from recent multicenter surveillance studies place this microrganism as the first Gram-negative species recovered in ICUs in Belgium [7] and Italy [8]. Several patient and pathogen-specific risk factors are associated with acquisition of this pathogen in ICUs, such as length of stay, severity of underlying disease and exposure to invasive procedures, on the one hand [5, 9], and virulence, adherence, and antimicrobial drug resistance on the other [10, 11]. The hospital environment, particularly moist sites, are known reservoirs of P. aeruginosa strains, often multidrug resistant (MDR) due to intrinsic and acquired determinants [12]. Although one possible explanation of the spread of antibiotic-resistant strains in ICUs is the selection exerted by extensive use of antibiotics, increased spread of MDR P. aeruginosa may be due to transmissions of resistant clones between patients [13]. Furthermore, it has been suggested that infection represents merely the tip of an iceberg, and that colonization reflects the submerged part. Colonization may be the first step of an endogenous infection, while the colonized patients represent a continuous exogenous source of microrganism for colonization/infection of other patients [10]. The relative importance of both colonization/infection pathways, essential to design appropriate prevention strategies, has rarely been exploited by active surveillance studies. The aim of our survey was to evaluate whether P. aeruginosa associated NI in our ICU originate mainly from patients' endogenous flora or from exogenous cross-transmission, by determining: (a) the occurrence of P. aeruginosa carriage upon admission, (b) the ICU- acquired P. aeruginosa infection and colonization rates by site, (c) the impact of cross-transmission using molecular typing data of the involved microrganisms, and (d) the occurrence of individual risk factors for MDR P. aeruginosa acquisition and their clinical impact. Preliminary results were presented in part at the 16th ECCMID [14].
Methods and materials
Patients and setting
During the study period 123 patients were admitted in the ICU for a total of 2,169 days; two patients staying less than 3 days were excluded. Patients characteristics are summarized in Table 1. The study was conducted at the Azienda Ospedaliera Cannizzaro, a 700-bed acute care hospital in Catania, Sicily, with a 12-bed interdisciplinary ICU. Between January and July 2004 all patients who stayed at least 48 h at the ICU were enrolled in the 6-month prospective surveillance survey. The ICU was supported by a single diagnostic microbiological laboratory. Patients were treated either in an eight-bed room or in four single isolation rooms. Each ICU room has its own sink and trolley with equipment for patient care. Hand washing with a detergent skin cleansing solution (clorhexidine) is required between patient contacts; caps, masks, shoe covers, and gloves are required at all times in the patient rooms and bathrooms. Sterile gloves are required for wound care procedures. Standard precautions were in place in the unit. Hand hygiene procedures were routinely emphasized. Other infection control measures consisted of cleaning once daily with tap water of the sink and environment surfaces using glutaraldehyde and sodium hypochlorite solutions.
Surveillance methodology
A 6-months surveillance prospective survey was performed from January to July 2004, according to the Hospital in Europe Link for Infection Control through Surveillance protocol [15]. An electronic data form was created using the SPSS Data Entry Enterprise Server. The study focused on three different states: carriage upon admission, colonization of sterile sites and infections during ICU stay. Patients with screening cultures testing positives in the absence of, or before isolation of, positive clinical specimens were considered to be carriers. Due to the lack of clinical data confirming infection patients with positive clinical specimens were considered to be colonized. When both clinical screening cultures testing positive on the same day, the patient was considered colonized [10]. Standard definitions of NI were used [15]. We included the following NI sites: pneumonia, bloodstream infections (BSI), central venous catheter-related BSI, and urinary tract infections (UTI). We considered only the first episode of infection or colonization per site and per patient. However, if infection developed after colonization at the same site, only the episode of infection was considered in the analysis. Finally, colonization and infections occurring during ICU stay in the carriers upon admission were not considered. Incidence rates of colonization and of infections were calculated both in terms of cumulative incidence and incidence density [16]. Calculation of National Nosocomial Infections Surveillance (NNIS) System device-associated infection rates and device utilization ratios were carried out in accordance with the methods described for the NNIS System, following the NNIS System report instructions [17]. The Simplified Acute Physiology Score (SAPS) II was used to assess patients' severity of illness at admission.
Bacterial isolates
During the study period routine clinical specimens and screening specimens (oropharyngeal, rectal, nasal, and axillary swabs) were collected and analyzed to identify P. aeruginosa. Screening cultures were collected from each patient on the day of admission. Clinical specimens were collected as requested by the medical staff. All isolates were identified by the Phoenix system (Becton Dickinson Diagnostic System, Pont de Claix, France). Antibiotic susceptibility was determined for the antimicrobial agents listed in Table 2, using an automated Phoenix microdilution system (Becton Dickinson Diagnostic System, Pont de Claix, France). Susceptibility criteria for the test method were in accordance with National Committee for Clinical Laboratory Standards Interpretative Criteria [18]. Isolates showing an intermediate level of susceptibility were classified as resistant. For each antimicrobial agent the resistance rate (RR) was calculated as the number of resistant isolates divided by the total number of isolates for which susceptibility testing had been carried out [19]. Antimicrobial RR were compared with reference RR percentiles distributions as reported by the Surveillance of Antimicrobial Use and Antimicrobial Resistance in Intensive Care Units (SARI) project [13] and by the Intensive Care Antimicrobial Resistance Epidemiology (ICARE) project [17]. MDR was defined as resistance of a P. aeruginosa isolate to at least three of the following four drugs: imipenem, ceftazidime, ciprofloxacin, and tobramycin [20]. We identified 138 P. aeruginosa isolates from 45 of the 121 patients, while 75 patients remained free of P. aeruginosa during their ICU stay.
Antibiotic use
The quantity of antimicrobial drugs was standardized by conversion to defined daily doses (DDD) [21]. To control for the population size at risk of receiving antimicrobials we determined the antimicrobial use density (AD) in WHO ATC groups [13].
Macrorestriction analysis and identification of cross-transmission episodes
Molecular typing by pulsed-field gel electrophoresis (PFGE) of the SpeI digested genomic DNA was performed as previously described [22]. Macrorestriction fragments were separated using a CHEF-DR III apparatus (Bio-Rad, Hercules, Calif., USA). Interpretation of genomic relatedness was performed using well established criteria [23–25]. The presence of two indistinguishable strains of P. aeruginosa in two patients was considered a single episode of cross-transmission [26].
Individual risk factors for MDR P. aeruginosa
To identify the individual risk factors for MDR P. aeruginosa a case-control study of the patients included in the surveillance was designed. All case patients were those with MDR P. aeruginosa while control patients were those with susceptible P. aeruginosa. The patients' characteristics and variables that were examined as possible risk factors are listed in Table 3. Patients' mortality and cross-transmission were also evaluated in relation to MDR P. aeruginosa infection.
Statistical analysis
Risk factors for MDR P. aeruginosa infections were identified by univariate analysis. The χ2 test or Fisher's exact test was used for categorical variables. Continuous variables were tested using Student's t test. All tests were two-tailed, and p < 0.05 was considered statistically significant. A statistical package (SPSS 14.0) was used for all analysis.
Results
Acquisition of P. aeruginosa colonization and infection during ICU stay
Two patients (1.7%) were carriers upon admission, and another two were colonized upon admission; these were not included in the surveillance. Thus 117 patients were eligible for follow-up of P. aeruginosa colonization and infection during ICU stay. Of these, 26 were colonized in the ICU (22.2%). The cumulative incidence of P. aeruginosa-sustained colonization in the ICU was 29.9/100 patients (35 episodes in 117 patients); the incidence density adjusted for the number of patient-days was 16.2/1,000 patient-days (35 episodes in 2,165 patient-days). Thirty patients were infected in the ICU (25.6%). The cumulative incidence of P. aeruginosa-sustained infections in the ICU was 36.7/100 patients (43 episodes in 117 patients); the incidence density was 19.9/1,000 patient-days (43 episodes in 2,165 patient-days). The cumulative incidence and the incidence density of infection were significantly higher than the cumulative incidence and the incidence density of colonization (p < 0.01). The proportion of each site-specific infection was: 51.2/100 infections for ICU-acquired pneumonia, 18.6/100 infections each for UTI and central line related local infections, 9.3/100 infections for BSI, and 2.3/100 infections for central line associated BSI. Site-specific incidence density values were: 10.2/1,000 patient-days for ICU-acquired pneumonia, 3.7/1,000 each for UTI and central line related local infections each, 1.8/1,000 for BSI, and 0.5/1,000 for central line associated BSI.
Device-associated infection rates and device utilization ratios
Device-associated infection rates and device utilization ratios were compared with the NNIS System reported rates and ratios [17]. Particularly the urinary catheter-associated UTI rate (8.1‰) was equal to the 90th percentile for the NNIS System. The urinary catheter utilization ratio was 0.91, between the 50th and 75th percentiles. The central line associated BSI rate was 4.6‰, between the 50th and 75th percentiles and the central line utilization ratio (0.99) was greater than the 90th percentile. The ventilator-associated pneumonia rate (30.5‰) was greater than the 90th percentile and the ventilator utilization ratio (0.88) was greater than the 90th percentile for the NNIS System.
Surveillance of antimicrobial resistance and antimicrobial use
Most of the P. aeruginosa isolates were resistant to multiple antibiotics (100 in 138 isolates, 72.5%); all isolates were resistant to trimethoprim/sulfamethoxazol. Comparison with reference RR percentiles distributions reported by the SARI [13] and ICARE projects [17] revealed high antimicrobial RR, greater than the 75th percentile, for penicillins with lactamase inhibitor, penicillins with extended spectrum, third generation cephalosporins, carbapenems, and quinolones (Table 2). The data on antimicrobial use were collected from the ICU covering 1070.4 DDD during the 6-months surveillance study. Table 4 provides data on the number of DDD, the AD values, and the comparison with reference AD percentile distributions reported by the SARI [19] and ICARE projects [17]. Comparison revealed high AD values, greater than the 75th percentile, for penicillins with lactamase inhibitor, trimethoprim/sulfamethoxazol, quinolones, aminoglycosides, carbapenems, and imidazole derivates. In addition to, AD values were lower than the 10th percentile for penicillins with extended spectrum and 3rd generation cephalosporins.
Macrorestriction analysis
Among the 138 P. aeruginosa isolates 102 collected from 37 patients were analyzed by macrorestriction. PFGE analysis of P. aeruginosa isolates led to the identification of 18 unrelated PFGE types, which we termed A–T, and five subtypes of clone A (termed A1–A5), one subtype each of clones D, G, and L (termed D1, G1, and L1, respectively). PFGE types A, D, E, F, G, H, and L were associated with cross-transmission of infection: clone A was represented by 62 of 102 isolates (60.8%) from 17 of 37 patients (45.9%); clone D was present in 4 isolates from 3 patients; clone E was present in 2 isolates from 2 patients; clone F was present in 5 isolates from 3 patients; clone G was present in 2 isolates from 2 patients; clone H was present in 2 isolates from 2 patients; and clone L was present in 7 isolates from 4 patients. The remaining PFGE types were single patterns associated with sporadic strains.
Identification of cross-transmission episodes
The total number of cross-transmission episodes was 15, thus the impact of P. aeruginosa cross-infection was estimated to be at least 44.1% (15 over 34 infections), but this figure was higher when cross-colonization was included (59.5%, 25 over 42 colonizations or infections), thus defining the preventable proportion of all P. aeruginosa-sustained cross-transmission episodes.
Antimicrobial susceptibility patterns of P. aeruginosa PFGE types
The analysis of antimicrobial susceptibility patterns showed that P. aeruginosa strains of PFGE profiles A, L, and S were all MDR. In addition to, strains of PFGE types A and S were resistant to all antimicrobials tested except to amikacin. Generally, all P. aeruginosa PFGE types were susceptible to amikacin and resistant to trimethoprim/sulfamethoxazol. Strains of PFGE types B, H, M, N, P, and R were susceptible to all antimicrobials tested except to trimethoprim/sulfamethoxazol. During the 6-month surveillance study 27 patients with MDR P. aeruginosa (case group) and 18 patients with susceptible P. aeruginosa (control group) were obtained. Proportions and means for the different variables in the two groups are listed in Table 3. Univariate analysis showed that MDR P. aeruginosa infections were only significantly related to cross transmission of infections (p = 0.002). Mortality was not significantly higher in MDR P. aeruginosa patients (32.0%) than in controls (33.3%) (odds ratio 1.063, 95% confidence interval 0.292–3.863). The results of individual risk factors univariate analysis are displayed in Table 3. No variable was found to be a significant risk factor for infection with MDR P. aeruginosa.
Discussion
Our study provided an epidemiological scenario of P. aeruginosa acquisition during ICU stay using a standardized protocol intended for advanced risk-adjusted comparison of rates with those reported by international networks such as NNIS, HELICS, and SARI. The low proportion of patients positive upon admission was consistent with the findings of other studies [10, 27, 28] while the cumulative incidence of P. aeruginosa acquisition during ICU stay was as high as 35.0/100 patients (42 colonized or infected patients in 117).
In our study we adopted the sophisticated approach of the P. aeruginosa surveillance program of Bertrand et al. [10]. Thus instead of limiting surveillance to infection, merely the tip of the iceberg, we also included surveillance of colonization [29]. Rates of infection were significantly higher than those of colonization. According to the definitions of Bertrand et al., if infection developed after colonization at the same site, only the episode of infection was considered in the analysis. This might explain the higher rate of infection than the colonization rate in our survey. However, colonization represented a substantial part of the bacterial load within the ICU and a continuous potential source of cross-transmission [10, 27].
Device-associated infection rates and device utilization ratios should be evaluated together for appropriate interpretation [17]. The most frequent infection type was ventilator-associated pneumonia, with a rate and a ventilator utilization ratio both exceeding the 90th percentile for the NNIS System, highlighting the need of reducing the use or limiting the duration with which such device is used in order to lower the ventilator-associated pneumonia rate in the unit. The urinary catheter associated UTI rate was equal to the 90th percentile for the NNIS System, but the urinary catheter utilization ratio was lower than the 75th percentile; the central-line utilization ratio exceeded the 90th percentile, but the central line associated BSI was lower than the 75th percentile. As such, device associated UTI and BSI cannot be defined as a problem in our ICU, but merely as an area for further investigation.
It has been suggested that high resistant rates of pathogens associated with NI in the ICUs are correlated with high rates of antibiotic use, but increased RR may also be due to transmission between patients [13]. Thus high RR of sporadic strains in the presence of high AD, as observed for penicillins with lactamase inhibitor, trimethoprim/sulfamethoxazol, quinolones, and carbapenems, would primarily suggest an overuse of these antimicrobials; on the other hand, low AD for third-generation cephalosporins and for penicillins with extended spectrum, in the presence of high RR, i.e., to ceftazidime, cefepime, and piperacillin, shown by P. aeruginosa strains of pulse type A, may be explained by cross-transmission. Evidence of such spread is reflected by the predominance of clone A, 60.8% of isolates involving 45.9% of patients. High AD in the presence of high RR of epidemic clones may indicate that both explanations hold true. In our study the acquisition of MDR P. aeruginosa was consistently associated with cross-transmission (p = 0.002).
Infections that seem to be most amenable to infection control measures are those that result from transmission between patients. However, efforts to measure the frequency of these events are scarce [3]. The aim of our survey was to evaluate, based on microbiological routine investigations, whether P. aeruginosa-associated NI in the ICU originate mainly from patients' endogenous flora or from exogenous cross-transmission. The impact of cross-transmission in our ICU, representing the preventable proportion of P. aeruginosa acquisition, was estimated to be at least 59.5% of all colonization/infection episodes, even higher than the estimate of about 50% reported in recent studies [3, 10]. In general, the estimated proportion of cases resulting from transmission vary depending on the occurrence of outbreaks in the period of study [30]. This study provides evidence that an outbreak of P. aeruginosa has occurred in our ICU.
In conclusion, although no periodical surveillance cultures were performed, our results suggest that the ICU personnel and environment serve as reservoirs for cross-transmission, and emphasize the importance of exogenous acquisition of MDR P. aeruginosa. Active surveillance of NI and antibiotic use is of paramount importance to address guidelines for empirical antibiotic regimens, reduction in antibiotic pressure and prompt enforcement of infection control measures in an evidence-based way.
References
Burke JP (2003) Infection control–a problem for patient safety. N Engl J Med 348:651–656
Wenzel RP, Thompson RL, Landry SM (1983) Hospital-acquired infections in intensive care unit patients: an overview with emphasis on epidemics. Infect Control 4:371–375
Grundmann H, Barwolff S, Tami A, Behnke M, Schwab F, Geffers C, Halle E, Gobel UB, Schiller R, Jonas D, Klare I, Weist K, Witte W, Beck-Beilecke K, Schumacher M, Ruden H, Gastmeier P (2005) How many infections are caused by patient-to-patient transmission in intensive care units? Crit Care Med 33:946–951
Trautmann M, Lepper PM, Haller M (2005) Ecology of Pseudomonas aeruginosa in the intensive care unit and the evolving role of water outlets as a reservoir of the organism. Am J Infect Control 33:41–49
Vincent JL, Bihari DJ, Suter PM, Bruining HA, White J, Nicolas-Chanoin MH, Wolff M, Spencer RC, Hemmer M (1995) The prevalence of nosocomial infection in intensive care units in Europe. Results of the European Prevalence of Infection in Intensive Care (EPIC) Study. EPIC International Advisory Committee. JAMA 274:639–644
Vincent JL (2000) Microbial resistance: lessons from the EPIC study. European prevalence of infection. Intensive Care Med 26(Suppl 1):S3–S8
Glupczynski Y, Delmee M, Goossens H, Struelens MJ (2001) Distribution and prevalence of antimicrobial resistance among gram-negative isolates in intensive care units (ICU) in Belgian hospitals between 1996 and 1999. Acta Clin Belg 56:297–306
Jones ME, Draghi DC, Thornsberry C, Karlowsky JA, Sahm DF, Wenzel RP (2004) Emerging resistance among bacterial pathogens in the intensive care unit–a European and North American Surveillance study (2000–2002). Ann Clin Microbiol Antimicrob 3:14
Rello J, Ollendorf DA, Oster G, Vera-Llonch M, Bellm L, Redman R, Kollef MH, VAP Outcomes Scientific Advisory Group (2002) Epidemiology and outcomes of ventilator-associated pneumonia in a large US database. Chest 122:2115–2121
Bertrand X, Thouverez M, Talon D, Boillot A, Capellier G, Floriot C, Helias JP (2001) Endemicity, molecular diversity and colonisation routes of Pseudomonas aeruginosa in intensive care units. Intensive Care Med 27:1263–1268
Aloush V, Navon-Venezia S, Seigman-Igra Y, Cabili S, Carmeli Y (2006) Multidrug-resistant Pseudomonas aeruginosa: risk factors and clinical impact. Antimicrob Agents Chemother 50:43–48
Deplano A, Denis O, Poirel L, Hocquet D, Nonhoff C, Byl B, Nordmann P, Vincent JL, Struelens MJ (2005) Molecular characterization of an epidemic clone of panantibiotic-resistant Pseudomonas aeruginosa. J Clin Microbiol 43:1198–1204
Meyer E, Jonas D, Schwab F, Rueden H, Gastmeier P, Daschner FD (2003) Design of a surveillance system of antibiotic use and bacterial resistance in German intensive care units (SARI). Infection 31:208–215
Agodi A, Barchitta M, Giaquinta L, Romeo MA, Denaro C (2006) Pseudomonas aeruginosa infection in ICU patients: highlighting the silent epidemic. Clin Microbiol Infect 12 (Suppl 4):P1022
Hospital In Europe Link For Infection Control Through Surveillance (2004) Surveillance of nosocomial infections in intensive care units. Protocol version 6.1, September; available at: http://Helics.Univ-Lyon1.Fr/
Horan TC, Emori TG (1997) Definitions of key terms used in the NNIS System. Am J Infect Control 25:112–116
National Nosocomial Infections Surveillance System (2004) Report: data summary from January 1992 through June 2004. Am J Infect Control 32:470–485
National Committee for Clinical Laboratory Standards (2002) Methods for dilution antimicrobial susceptibility test for bacteria that grow aerobically, 5th edn. Approved standard M7-A5. National Committee for Clinical Laboratory Standards, Wayne, Pa.
Meyer E, Schwab F, Jonas D, Rueden H, Gastmeier P, Daschner FD (2004) Surveillance of Antimicrobial Use and Antimicrobial Resistance in Intensive Care Units (SARI): I. Antimicrobial use in German intensive care units. Intensive Care Med 30:1089–1096
Jung R, Fish DN, Obritsch MD, MacLaren R (2004) Surveillance of multi-drug resistant Pseudomonas aeruginosa in an urban tertiary-care teaching hospital. J Hosp Infect 57:105–111
Anonymous (1999) ATC index with DDDs WHO collaborating centre for drug statistics methodology. WHO, Oslo
Agodi A (2000) Sistemi di tipizzazione epidemiologica dei microrganismi responsabili di infezioni in ospedale: uso e valutazione. Ann Ig 12:41–46
Struelens M (1996) Consensus guidelines for appropriate use and evaluation of microbial epidemiologic typing systems. Clin Microbiol Infect 2:2–11
Tenover F, Arbeit R, Goering R (1997) How to select and interpret molecular strain typing methods for epidemiological studies of bacterial infections: a review for healthcare epidemiologists. Infect Control Hosp Epidemiol 18:426–439
Tenover FC, Arbeit RD, Goering RV, Mickelsen PA, Murray BE, Persing DH, Swaminathan B (1995) Interpreting chromosomal DNA restriction patterns produced by pulsed field gel electrophoresis: criteria for bacterial strain typing. J Clin Microbiol 33:2233–2239
Weist K, Pollege K, Schultz I, Ruden H, Gastmeier P (2002) How many nosocomial infections are associated with cross-transmission? A prospective cohort study in a surgical intensive care unit. Infect Control Hosp Epidemiol 23:127–132
Bergmans DC, Bonten MJ, van Tiel FH, Gaillard CA, van der Geest S, Wilting RM, de Leeuw PW, Stobberingh EE (1998) Cross-colonisation with Pseudomonas aeruginosa of patients in an intensive care unit. Thorax 53:1053–1058
Cattoen C, Levent T, Granbastien B, Descamps D, Bouillet L, Coignard B, Beaucaire G, reseau de microbiologists de l'ARECLIN (1999) Observatoire regional Pseudomonas aeruginosa du Nord Pas de Calais: donnèes epidemiologiques et microbiologiques. Med Mal Infect 29:160–166
Langer M, Carretto E, Haeusler EA (2001) Infection control in ICU: back (forward) to surveillance samples? Intensive Care Med 27:1561–1563
Mikolajczyk RT, Sagel U, Bornemann R, Kramer A, Kretzschmar M (2007) A statistical method for estimating the proportion of cases resulting from cross-transmission of multi-resistant pathogens in an intensive care unit. J Hosp Infect 65:149–155
Author information
Authors and Affiliations
Corresponding author
Additional information
This work was supported by grants to A. A. from the University of Catania, Italy.
Rights and permissions
About this article
Cite this article
Agodi, A., Barchitta, M., Cipresso, R. et al. Pseudomonas aeruginosa carriage, colonization, and infection in ICU patients. Intensive Care Med 33, 1155–1161 (2007). https://doi.org/10.1007/s00134-007-0671-6
Received:
Accepted:
Published:
Issue Date:
DOI: https://doi.org/10.1007/s00134-007-0671-6