Abstract
Imazamox is an imidazolinone herbicide, a new class of pesticides, which can exist as cationic, anionic or neutral species in water. The adsorption isotherms of Imazamox onto Filtrasorb 400 (F400) activated carbon were determined varying the pH and the ionic strength of the aqueous medium. The results show that ionic strength has no significant effect on Imazamox uptake, contrary to pH, and that F400 has a high affinity for Imazamox. Moreover, it is found that Imazamox adsorbs onto F400 as its neutral form. The best fit of the experimental points is obtained with the Langmuir–Freundlich model, consistent with surface site heterogeneity. Finally, calculating Langmuir–Freundlich isotherms for various constant pH values, it is shown that the two plateaus observed in the experimental isotherms obtained at free pH are due to the variation of the pH along the isotherms.







Similar content being viewed by others
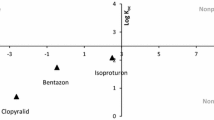
References
Council Directive 98/83/EC. Directive on the quality of water intended for human consumption. Official Journal of the European Communities L 330/332. 333.311.1998 (1998)
A.J. Krynitsky, S.J. Stout, H. Nejad, T.C. Cavalier, J. AOAC Int. 82, 956 (1999)
T.C. Farnham. The Pesticide Manual. A world Compendium, 12th edn. (British Crop Protection Council, UK, 2000)
H. Safarpour, R. Asiaie, S. Katz, J. Chromatogr. A 1036, 217 (2004)
E. Quivet, R. Faure, J. Georges, J.O. Paisse, B. Herbreteau, P. Lanteri, J. Agr. Food Chem. 54, 3641 (2006)
G. Mangels (ed.), Behavior of the Imidazolinone Herbicides in the Aquatic Environment. (CRC Press, Boca Raton, 1991)
Pesticide Fact Sheet-Imazamox (raptor pesticide) 22.05.1997: Office of Prevention, Pesticides and Toxic Substances (1997)
J.S. Noh, J.A. Schwarz, J. Colloid Interf. Sci. 130, 157 (1989)
C. Morlay, J.-P. Joly, J. Porous Mater. 17, 535 (2009). doi:10.1007/s10934-009-9322-3
K.S.W. Sing, D.H. Everett, R.A.W. Haul, L. Moscou, R.A. Pierotti, J. Rouquerol et al., Pure Appl. Chem. 57, 603 (1985)
C.H. Giles, A.P. D’Silva, I.A. Easton, J. Colloid Interface Sci. 47, 766 (1974)
A.M. Duda, M. Dyba, H. Kozlowski, G. Micera, A. Pusino, J. Agr. Food Chem. 44, 3698 (1996)
L.R. Radovic, C. Moreno-Castilla, J. Rivera-Utrilla, in Chemistry and Physics of Carbon, vol. 27, ed. by L.R. Radovic (Marcel Dekker, New-York, 2000), pp. 227
R. Sips, J. Chem. Phys. 18, 1024 (1950)
R. Sips, J. Chem. Phys. 16, 490 (1948)
R.W. Coughlin, F.S. Ezra, Environ. Sci. Technol. 2, 291 (1968)
S. Haydar, M.A. Ferro-Garcia, J. Rivera-Utrilla, J.P. Joly, Carbon 41, 387 (2003)
Acknowledgments
The authors wish to thank Chemviron Carbon (European Operations of Calgon Carbon Corporation, USA) who kindly provided them with Filtrasorb 400 samples. The corresponding author also gratefully acknowledges kind encouragement from James Hickman, Director of the NanoScience Technology Center (NSTC, University of Central Florida, USA), during her stay at NSTC.
Author information
Authors and Affiliations
Corresponding author
Rights and permissions
About this article
Cite this article
Morlay, C., Quivet, E., Pilshofer, M. et al. Adsorption of Imazamox herbicide onto Filtrasorb 400 activated carbon. J Porous Mater 19, 79–86 (2012). https://doi.org/10.1007/s10934-011-9450-4
Published:
Issue Date:
DOI: https://doi.org/10.1007/s10934-011-9450-4