Abstract
Osteoporosis is a common skeletal disease characterized by low bone mass and microarchitectural deterioration of bone tissue, with a consequent increase in bone fragility and susceptibility to fracture. We previously demonstrated that Col1a1-SOX9 transgenic (TG) mice, in which SOX9 specifically expresses in osteoblasts driven by a 2.3-kb Col1a1 promoter, display osteopenia during the early postnatal stage. In this study, to further analyze the osteopenia phenotype and especially the effect of the osteoblast-specific expression of SOX9 on bone mechanical properties, we performed bone geometry and mechanical property analysis of long bones from Col1a1-SOX9 TG mice and wild-type littermates (WT) at different time points. Interestingly, after body weight adjustment, TG mice had similar whole-bone strength as WT mice but significantly thinner cortical bone, lower elastic modulus, and higher moment of inertia. Thus, osteoblast-specific SOX9 expression results in altered bone structure and material properties. Furthermore, the expression levels of Pcna, Col1a1, osteocalcin, and the Opg/Rankl ratio in TG mice were significantly lower until 4 months of age compared with WT mice, suggesting that TG mice have dysregulated bone homeostasis. Finally, bone marrow stromal cells (MSCs) isolated from TG mice display enhanced adipocyte differentiation and decreased osteoblast differentiation in vitro, suggesting that osteoblast-specific expression of SOX9 can lead to altered mesenchymal stem cell differentiation potentials. In conclusion, our study implies that SOX9 activity has to be tightly regulated in the adult skeleton to ensure optimal bone quality.






Similar content being viewed by others
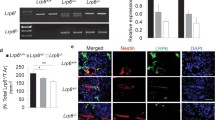
References
Ducy P (2000) Cbfa1: a molecular switch in osteoblast biology. Dev Dyn 219:461–471
Schroeder TM, Jensen ED, Westendorf JJ (2005) Runx2: a master organizer of gene transcription in developing and maturing osteoblasts. Birth Defects Res C Embryo Today 75:213–225
Ducy P, Zhang R, Geoffroy V, Ridall AL, Karsenty G (1997) Osf2/Cbfa1: a transcriptional activator of osteoblast differentiation. Cell 89:747–754
Komori T, Yagi H, Nomura S, Yamaguchi A, Sasaki K, Deguchi K, Shimizu Y, Bronson RT, Gao YH, Inada M, Sato M, Okamoto R, Kitamura Y, Yoshiki S, Kishimoto T (1997) Targeted disruption of Cbfa1 results in a complete lack of bone formation owing to maturational arrest of osteoblasts. Cell 89:755–764
Otto F, Thornell AP, Crompton T, Denzel A, Gilmour KC, Rosewell IR, Stamp GW, Beddington RS, Mundlos S, Olsen BR, Selby PB, Owen MJ (1997) Cbfa1, a candidate gene for cleidocranial dysplasia syndrome, is essential for osteoblast differentiation and bone development. Cell 89:765–771
Ducy P, Starbuck M, Priemel M, Shen J, Pinero G, Geoffroy V, Amling M, Karsenty G (1999) A Cbfa1-dependent genetic pathway controls bone formation beyond embryonic development. Genes Dev 13:1025–1036
Liu W, Toyosawa S, Furuichi T, Kanatani N, Yoshida C, Liu Y, Himeno M, Narai S, Yamaguchi A, Komori T (2001) Overexpression of Cbfa1 in osteoblasts inhibits osteoblast maturation and causes osteopenia with multiple fractures. J Cell Biol 155:157–166
Geoffroy V, Kneissel M, Fournier B, Boyde A, Matthias P (2002) High bone resorption in adult aging transgenic mice overexpressing cbfa1/runx2 in cells of the osteoblastic lineage. Mol Cell Biol 22:6222–6233
Mundlos S, Otto F, Mundlos C, Mulliken JB, Aylsworth AS, Albright S, Lindhout D, Cole WG, Henn W, Knoll JH, Owen MJ, Mertelsmann R, Zabel BU, Olsen BR (1997) Mutations involving the transcription factor CBFA1 cause cleidocranial dysplasia. Cell 89:773–779
Lee B, Thirunavukkarasu K, Zhou L, Pastore L, Baldini A, Hecht J, Geoffroy V, Ducy P, Karsenty G (1997) Missense mutations abolishing DNA binding of the osteoblast-specific transcription factor OSF2/CBFA1 in cleidocranial dysplasia. Nat Genet 16:307–310
Zhou G, Chen Y, Zhou L, Thirunavukkarasu K, Hecht J, Chitayat D, Gelb BD, Pirinen S, Berry SA, Greenberg CR, Karsenty G, Lee B (1999) CBFA1 mutation analysis and functional correlation with phenotypic variability in cleidocranial dysplasia. Hum Mol Genet 8:2311–2316
Otto F, Kanegane H, Mundlos S (2002) Mutations in the RUNX2 gene in patients with cleidocranial dysplasia. Hum Mutat 19:209–216
Vaughan T, Pasco JA, Kotowicz MA, Nicholson GC, Morrison NA (2002) Alleles of RUNX2/CBFA1 gene are associated with differences in bone mineral density and risk of fracture. J Bone Miner Res 17:1527–1534
Doecke JD, Day CJ, Stephens AS, Carter SL, van Daal A, Kotowicz MA, Nicholson GC, Morrison NA (2006) Association of functionally different RUNX2 P2 promoter alleles with BMD. J Bone Miner Res 21:265–273
Ralston SH, de Crombrugghe B (2006) Genetic regulation of bone mass and susceptibility to osteoporosis. Genes Dev 20:2492–2506
Akiyama H (2008) Control of chondrogenesis by the transcription factor Sox9. Mod Rheumatol 18:213–219
Lefebvre V, Huang W, Harley VR, Goodfellow PN, de Crombrugghe B (1997) SOX9 is a potent activator of the chondrocyte-specific enhancer of the pro alpha1(II) collagen gene. Mol Cell Biol 17:2336–2346
Bi W, Huang W, Whitworth DJ, Deng JM, Zhang Z, Behringer RR, de Crombrugghe B (2001) Haploinsufficiency of Sox9 results in defective cartilage primordia and premature skeletal mineralization. Proc Natl Acad Sci USA 98:6698–6703
Akiyama H, Chaboissier MC, Martin JF, Schedl A, de Crombrugghe B (2002) The transcription factor Sox9 has essential roles in successive steps of the chondrocyte differentiation pathway and is required for expression of Sox5 and Sox6. Genes Dev 16:2813–2828
Zhou G, Zheng Q, Engin F, Munivez E, Chen Y, Sebald E, Krakow D, Lee B (2006) Dominance of SOX9 function over RUNX2 during skeletogenesis. Proc Natl Acad Sci USA 103:19004–19009
Akiyama H, Kim JE, Nakashima K, Balmes G, Iwai N, Deng JM, Zhang Z, Martin JF, Behringer RR, Nakamura T, de Crombrugghe B (2005) Osteo-chondroprogenitor cells are derived from Sox9 expressing precursors. Proc Natl Acad Sci USA 102:14665–14670
Yin T, Li L (2006) The stem cell niches in bone. J Clin Invest 116:1195–1201
Caplan AI (2008) All MSCs are pericytes? Cell Stem Cell 3:229–230
Karsenty G, Kronenberg HM, Settembre C (2009) Genetic control of bone formation. Annu Rev Cell Dev Biol 25:629–648
Luo D, Renault VM, Rando TA (2005) The regulation of Notch signaling in muscle stem cell activation and postnatal myogenesis. Semin Cell Dev Biol 16:612–622
Lefebvre V, Smits P (2005) Transcriptional control of chondrocyte fate and differentiation. Birth Defects Res C Embryo Today 75:200–212
Komori T (2006) Regulation of osteoblast differentiation by transcription factors. J Cell Biochem 99:1233–1239
Farmer SR (2006) Transcriptional control of adipocyte formation. Cell Metab 4:263–273
Crisan M, Yap S, Casteilla L, Chen CW, Corselli M, Park TS, Andriolo G, Sun B, Zheng B, Zhang L, Norotte C, Teng PN, Traas J, Schugar R, Deasy BM, Badylak S, Buhring HJ, Giacobino JP, Lazzari L, Huard J, Peault B (2008) A perivascular origin for mesenchymal stem cells in multiple human organs. Cell Stem Cell 3:301–313
Kobayashi H, Gao Y, Ueta C, Yamaguchi A, Komori T (2000) Multilineage differentiation of Cbfa1-deficient calvarial cells in vitro. Biochem Biophys Res Commun 273:630–636
Enomoto H, Furuichi T, Zanma A, Yamana K, Yoshida C, Sumitani S, Yamamoto H, Enomoto-Iwamoto M, Iwamoto M, Komori T (2004) Runx2 deficiency in chondrocytes causes adipogenic changes in vitro. J Cell Sci 117:417–425
Jepsen KJ, Pennington DE, Lee YL, Warman M, Nadeau J (2001) Bone brittleness varies with genetic background in A/J and C57BL/6J inbred mice. J Bone Miner Res 16:1854–1862
Merciris D, Schiltz C, Legoupil N, Marty-Morieux C, de Vernejoul MC, Geoffroy V (2007) Over-expression of TIMP-1 in osteoblasts increases the anabolic response to PTH. Bone 40:75–83
Zheng Q, Zhou G, Morello R, Chen Y, Garcia-Rojas X, Lee B (2003) Type X collagen gene regulation by Runx2 contributes directly to its hypertrophic chondrocyte-specific expression in vivo. J Cell Biol 162:833–842
Meirelles Lda S, Nardi NB (2003) Murine marrow-derived mesenchymal stem cell: isolation, in vitro expansion, and characterization. Br J Haematol 123:702–711
Nadri S, Soleimani M, Hosseni RH, Massumi M, Atashi A, Izadpanah R (2007) An efficient method for isolation of murine bone marrow mesenchymal stem cells. Int J Dev Biol 51:723–729
Kalajzic I, Kalajzic Z, Kaliterna M, Gronowicz G, Clark SH, Lichtler AC, Rowe D (2002) Use of type I collagen green fluorescent protein transgenes to identify subpopulations of cells at different stages of the osteoblast lineage. J Bone Miner Res 17:15–25
Glantz SA, Slinker BK (2001) Primer of applied regression & analysis of variance. McGraw-Hill, New York
Khosla S (2001) Minireview: the OPG/RANKL/RANK system. Endocrinology 142:5050–5055
Corsi KA, Pollett JB, Phillippi JA, Usas A, Li G, Huard J (2007) Osteogenic potential of postnatal skeletal muscle-derived stem cells is influenced by donor sex. J Bone Miner Res 22:1592–1602
Rossert J, Eberspaecher H, de Crombrugghe B (1995) Separate cis-acting DNA elements of the mouse pro-alpha 1(I) collagen promoter direct expression of reporter genes to different type I collagen-producing cells in transgenic mice. J Cell Biol 129:1421–1432
Yin D, Wang Z, Gao Q, Sundaresan R, Parrish C, Yang Q, Krebsbach PH, Lichtler AC, Rowe DW, Hock J, Liu P (2009) Determination of the fate and contribution of ex vivo expanded human bone marrow stem and progenitor cells for bone formation by 2.3ColGFP. Mol Ther 17:1967–1978
Ammann P, Rizzoli R (2003) Bone strength and its determinants 14 suppl. Osteoporos Int 3:S13–S18
Xiao ZS, Hjelmeland AB, Quarles LD (2004) Selective deficiency of the “bone-related” Runx2-II unexpectedly preserves osteoblast-mediated skeletogenesis. J Biol Chem 279:20307–20313
Xiao Z, Awad HA, Liu S, Mahlios J, Zhang S, Guilak F, Mayo MS, Quarles LD (2005) Selective Runx2-II deficiency leads to low-turnover osteopenia in adult mice. Dev Biol 283:345–356
Cheng A, Genever PG (2010) SOX9 determines RUNX2 transactivity by directing intracellular degradation. J Bone Miner Res 25:2404–2413
Schmidt K, Schinke T, Haberland M, Priemel M, Schilling AF, Mueldner C, Rueger JM, Sock E, Wegner M, Amling M (2005) The high mobility group transcription factor Sox8 is a negative regulator of osteoblast differentiation. J Cell Biol 168:899–910
Engler AJ, Sen S, Sweeney HL, Discher DE (2006) Matrix elasticity directs stem cell lineage specification. Cell 126:677–689
Shih YR, Tseng KF, Lai HY, Lin CH, Lee OK (2011) Matrix stiffness regulation of integrin-mediated mechanotransduction during osteogenic differentiation of human mesenchymal stem cells. J Bone Miner Res 26:730–738
Rodriguez JP, Garat S, Gajardo H, Pino AM, Seitz G (1999) Abnormal osteogenesis in osteoporotic patients is reflected by altered mesenchymal stem cells dynamics. J Cell Biochem 75:414–423
Rodriguez JP, Montecinos L, Rios S, Reyes P, Martinez J (2000) Mesenchymal stem cells from osteoporotic patients produce a type I collagen-deficient extracellular matrix favoring adipogenic differentiation. J Cell Biochem 79:557–565
Oh CD, Maity SN, Lu JF, Zhang J, Liang S, Coustry F, de Crombrugghe B, Yasuda H (2010) Identification of SOX9 interaction sites in the genome of chondrocytes. PLoS One 5:e10113
Ikegami D, Akiyama H, Suzuki A, Nakamura T, Nakano T, Yoshikawa H, Tsumaki N (2011) Sox9 sustains chondrocyte survival and hypertrophy in part through Pik3ca-Akt pathways. Development 138:1507–1519
Pritchett J, Athwal V, Roberts N, Hanley NA, Hanley KP (2011) Understanding the role of SOX9 in acquired diseases: lessons from development. Trends Mol Med 17:166–174
Acknowledgement
This work was supported by NIH grant DE15139 and a Case start-up fund (G. Z.) as well as fellowship support from the Department of Anatomy, Case Western Reserve University (M. M. C.). We thank Chiderah Okoye and Justin Daggett for assistance with image analysis, Teresa Pizzuto for expert histology work, and Dr. David Rowe for 2.3 Col1a1-GFP mice. We also thank Valerie Schmedlen for editorial assistance and Dr. Shunichi Murakami for critical reading of the manuscript.
Author information
Authors and Affiliations
Corresponding author
Additional information
The authors have stated that they have no conflict of interest.
Electronic supplementary material
Below is the link to the electronic supplementary material.
Rights and permissions
About this article
Cite this article
Liang, B., Cotter, M.M., Chen, D. et al. Ectopic Expression of SOX9 in Osteoblasts Alters Bone Mechanical Properties. Calcif Tissue Int 90, 76–89 (2012). https://doi.org/10.1007/s00223-011-9550-9
Received:
Accepted:
Published:
Issue Date:
DOI: https://doi.org/10.1007/s00223-011-9550-9