Abstract
Photosynthesis was studied in four species of red marine macroalgae: Palmaria palmata, Laurencia pinnatifida, Lomentaria articulata and Delesseria sanguinea. The rate of O2 evolution for submersed photosynthesis was measured as a function of incident photon flux density at normal pH and inorganic carbon concentration (pH 8.0, 2 mol m−3), and as a function of inorganic carbon concentration at pH 8.0 at saturating and at limiting photon flux density. The rate of CO2 uptake was measured for emersed photosynthesis as a function of CO2 partial pressure at saturating photon flux density. Previous pH-drift results suggest that Palmaria and Laurencia are able to use HCO sup−inf3 as well as CO2 whereas Lomentaria and Delesseria are restricted to CO2. None of the algae are saturated by 2 mol m−3 inorganic carbon at high light (400 μmol m−2 s−1) but are saturated at low light (35 μmol m−2 s−1). The inorganic C concentration at which half the light-saturated rate of O2 evolution is achieved is higher for Palmaria and Laurencia (1.51 and 1.85 mol m−3) than for Lomentaria and Delesseria (0.772 and 0.841 mol m−3). The lower values for the latter two species could reflect their putative restriction to CO2. If expressed in terms of CO2, the half-saturation values yield 7.2 and 7.8 mmol m−3 respectively, which are very similar to values obtained previously during pH-drift experiments but at lower concentrations of HCO sup−inf3 , consistent with restriction to CO2. The photosynthetic conductance (m s−1), calculated from the initial slope for photosynthesis at low concentrations of inorganic carbon, correlates with the suggested ability to extract inorganic carbon based on pH-drift results. Calculations made assuming that CO2 is the only species diffusing across the boundary layer are consistent with boundary layer thicknesses of 20 and 19 μm for Lomentaria and Delesseria respectively, which is feasible given the rapid water movement in the experiments. For Laurencia however, an unreasonably small boundary layer thickness of 6 μm is necessary to explain the flux, which indicates co-diffusion by HCO sup−inf3 . In the apparent absence of external carbonic anhydrase, direct uptake of HCO sup−inf3 , rather than external conversion to CO2 is indicated in this species. In air, the CO2 concentration at which photosynthesis is half-maximal increases in the same order as the ability to raise pH in drift experiments. At 21 kPa the CO2 compensation partial pressures for Palmaria and Laurencia at 0.56 and 1.3 Pa are low enough to suggest a carbon-concentrating mechanism is operating, while those of Lomentaria at 1.8 Pa and particularly that of Delesseria at 4.5 Pa could be explained without a carbon-concentrating mechanism. The algae tested (all except Delesseria) showed more O2 evolution than could be accounted for with a photosynthetic quotient of 1.0 and uncatalysed conversion of HCO sup−inf3 to CO2 outside the cell in high light at pH 8.0 when high algal fresh weight per unit medium was used. These results are concordant with other data suggesting use of HCO sup−inf3 by Palmaria and Laurencia, but discordant with the rest of the available information in indicating use of HCO sup−inf3 by Lomentaria. The reason for this is unclear. The lightsaturated rate of O2 evolution on an algal area basis and the photon flux density needed to saturate photosynthesis were related partly to the habitat from which the seaweeds were collected, but more strongly to the ability to use HCO sup−inf3 . Values for the two users of HCO sup−inf3 , Palmaria (population used was intertidal; also occurs subtidally) and Laurencia (intertidal/shaded intertidal), were greater than for Lomentaria (shaded intertidal), which was greater than Delesseria (subtidal), both of which are believed to be restricted to CO2. In accordance with earlier δ13C data and, for Delesseria, estimates of the achieved growth rates in situ, carbon is likely to be saturating and use of HCO sup−inf3 is unlikely to occur in the normal low-light habitats of Lomentaria and Delesseria. Analysis of N-use efficiencies show that they are closer to the low-CO2-affinity Laminariales than the high-CO2-affinity Fucaceae.
Similar content being viewed by others
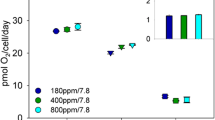
References
Axelsson L, Uusitalo J (1988) Carbon acquisition strategies for marine macroalgae. I. Utilization of proton exchanges visualized during photosynthesis in a closed system. Mar Biol 97: 295–300
Bauer H, Martha P, Kirchner-Heiss B, Mairhoffer I (1983) The CO2 compensation point of C3 plants — a re-examination. II. Intraspecific variability. Z Pflanzenphysiol 109: 143–154
Beer S, Israel A, Dreschler Z, Cohen Y (1990) Photosynthesis in Ulva fasciata. V. Evidence for an inorganic carbon concentrating system, and RUBISCO CO2 kinetics. Plant Physiol 94: 1542–1546
Beer S, Sand-Jensen K, Madsen TV, Nielsen SL (1991) The carboxylase activity of Rubisco and the photosynthetic performance in aquatic plants. Oecologia 87: 429–434
Ben-Zion M, Dubinsky Z (1988) An on-line system for measuring photosynthetic characteristics via an oxygen electrode. J Plankton Res 10: 555–558
Bowes G (1985) Pathways of CO2 fixation by aquatic organisms. In: Lucas WJ, Berry JA (eds) Inorganic carbon uptake by aquatic photosynthetic organisms. The American Society of Plant Physiologists, Rockville MD, pp 187–210
Cook CM, Colman B (1987) Some characteristics of photosynthetic inorganic carbon uptake of a marine macrophytic red alga. Plant Cell Environ 10: 275–278
Cook CM, Lanaras T, Colman B (1986) Evidence for bicarbonate transport in species of red and brown macrophytic marine algae. J Exp Bot 37: 977–984
Cook CM, Lanaras T, Roubelakis-Angelakis KA (1988) Bicarbonate transport and alkalization of the medium by four species of Rhodophyta. J Exp Bot 39: 1185–1198
Drew EA (1977) The physiology of photosynthesis and respiration in some antarctic marine algae. Br Antarct Surv Bull 46: 59–76
Giordano M, Maberly SC (1989) Distribution of carbonic anhydrase in British marine macroalgae. Oecologia 81: 534–539
Helder RJ (1985) Diffusion of inorganic carbon across and unstirred layer: a simplified quantitative approach. Plant Cell Environ 8: 399–408
Hilditch CM, Jones PB, Balding P, Smith AJ, Rogers LJ (1991) Ribulose bisphosphate carboxylases from macroalgae: properties during extraction and properties of the enzyme from Porphyra umbilicalis. Phytochemistry 30: 745–750
Holbrook GP, Beer S, Spencer WE, Reiskind JB, Davis JS, Bowes G (1988) Photosynthesis in marine macroalgae: evidence for carbon limitation. Can J Bot 66: 577–582
Jassby AD, Platt T (1976) Mathematical formulation of the relationship between photosynthesis and light for phytoplankton. Limnol Oceanogr 21: 540–547
Johnson KS (1982) Carbon dioxide hydration and dehydration kinetics in seawater. Limnol Oceanogr 27: 849–55
Johnston AM (1991) The acquisition of inorganic carbon by marine macroalgae. Can J Bot 69: 1123–1132
Johnston AM, Raven JA (1986a) The analysis of photosynthesis in air and water of Ascophyllum nodosum (L.) Le Jol. Oecologia 69: 288–95
Johnston AM, Raven JA (1986b) The utilization of bicarbonate ions by the macroalga Ascophyllum nodosum (L.) Le Jolis. Plant Cell Environ 9: 175–84
Johnston AM, Raven JA (1987) The C4-like characteristics of the intertidal macroalga Ascophyllum nodosum (L.) Le Jolis (Fucales, Phaeophyta). Phycologia 26: 159–166
Johnston AM, Raven JA (1989) Extraction, partial purification and characterization of phosphoenolpyruvate carboxykinase from Ascophyllum nodosum (Phaeophyceae). J Phycol 25: 568–76
Kain J (1984) Seasonal growth of two subtidal species of Rhodophyta off the Isle of Man. J Exp Mar Biol Ecol 82: 207–220
Kay E (1990) Photosynthesis and inorganic carbon accumulation mechanisms in marine intertidal macroalgae. PhD Thesis, University of Newcastle upon Tyne
Kremer BP (1981) Metabolic implications of non-photosynthetic carbon fixation in brown macroalgae. Phycologia 20: 242–50
Kuebler JE, Davison I, Yarish C (1990) Photosynthetic adaptation to temperature in the red algae Lomentaria baileyana and Lomentaria orcadensis. Br Phycol J 26: 9–19
Lüning K (1979) Growth studies of three Laminaria species (Phaeophyceae). Mar Ecol Prog Ser 1: 195–207
Maberly SC (1990) Exogenous sources of inorganic carbon for photosynthesis by marine macroalgae. J Phycol 26: 439–49
Maberly SC (1992) Carbonate ions appear to neither inhibit nor stimulate use of bicarbonate ions in photosynthesis by Ulva lactuca. Plant Cell Environ 15: 255–260
Maberly SC, Raven JA, Johnston AM (1992) Discrimination between 12C and 13C by marine plants. Oecologia 91: 481–492
Madsen TV, Maberly SC (1990) A comparison of air and water as environments for photosynthesis by the intertidal alga Fucus spiralis (Phaeophyta). J Phycol 26: 24–30
Oates BR (1986) Components of photosynthesis in the intertidal saccate alga Halosaccion americanum (Rhodophyta, Palmariales). J Phycol 22: 217–223
Raven JA (1970) Exogenous inorganic carbon sources in plant photosynthesis. Biol Rev 45: 167–211
Raven JA (1984) Energetics and transport in aquatic plants. A. R. Liss, New York
Raven JA, Johnston AM, MacFarlane JJ, bin Surif M, McInroy S (1987) Diffusion and active transport of inorganic carbon in freshwater and marine macroalgae. In: Biggins J (ed) Progress in photosynthesis research, Vol 4. Nijhoff, Dordrecht, pp 333–340
Raven JA, Johnston AM, MacFarlane JJ (1990a) Carbon metabolism. In: Sheath RG, Cole KM (eds) The biology of the red algae. Cambridge University Press, Cambridge, pp 41–60
Raven JA, Johnston AM, Handley LL, McInroy SG (1990b) Transport and assimilation of inorganic carbon by Lichina pygmaea under emersed and submersed conditions. New Phytol 114: 407–417
Reiskind JB, Bowes G (1991) The role of phosphoenolpyruvate carboxykinase in a marine macroalga with C4-like photosynthetic characteristics. Proc Natl Acad Sci USA 88: 2883–2887
Sand-Jensen K, Gordon DM (1984) Differential ability of marine and freshwater marcophytes to utilize HCO −3 and CO2 Mar Biol 80: 247–253
Seybold A, Egle K (1937) Quantitative Untersuchungen über die Chlorophylle und Carotinoide der Meeresalgen. Jahrb Wiss Bot 84: 50–80
Smith FA, Walker NA (1980) Photosynthesis by aquatic plants: effects of unstirred layers in relation to assimilation of CO2 and HCO −3 and to carbon isotope discrimination. New Phytol 86: 245–59
Smith RG, Bidwell RGS (1989a) Inorganic carbon uptake by photosynthetically active protoplasts of red macroalga, Chondrus crispus. Mar Biol 102: 1–4
Smith RG, Bidwell RGS (1989b) Mechanism of photosynthetic carbon dioxide uptake by the red macroalga, Chondrus crispus. Plant Physiol 89: 93–99
Surif MB, Raven JA (1989) Exogenous inorganic carbon sources for photosynthesis in seawater of the Fucales and Laminariales (Phaeophyta): ecological and taxonomic implications. Oecologia 78: 97–105
Surif MB, Raven JA (1990) Photosynthetic gas exchange under emersed conditions in eulittoral and normally submersed members of the Fucales and the Laminariales: interpretation in relation to C isotope ratio and N and water use efficiency. Oecologia 82: 68–80
Uusitalo J, Axelsson L, Carlberg S, Larsson C, Ryberg H (1990) CO2 storage and CO2 concentrating in brown seaweeds. II. Function in gas phase. In: Baltsheffsky M (ed) Current Research in Photosynthesis. Kluwer, Dordrecht, pp 521–524
Weyers JDB, Paterson NW, A'Brook R (1987) Towards a quantitative definition of plant hormone sensitivity. Plant Cell Environ 10: 1–10
Author information
Authors and Affiliations
Rights and permissions
About this article
Cite this article
Johnston, A.M., Maberly, S.C. & Raven, J.A. The acquisition of inorganic carbon by four red macroalgae. Oecologia 92, 317–326 (1992). https://doi.org/10.1007/BF00317457
Received:
Accepted:
Issue Date:
DOI: https://doi.org/10.1007/BF00317457