Abstract
Stroke, the leading cause of long-term disability worldwide, is caused by the blockage or hemorage of cerebral arteries. The resultant cerebral ischemia causes local neuronal death and brain injury. Histone deacetylase 9 (HDAC9) has been reported to be elevated in ischemic brain injury, but its mechanism in stroke is still enigmatic. The present study aimed to unveil the manner of regulation of HDAC9 expression and the effect of HDAC9 activation on neuronal function in cerebral ischemia. MicroRNAs (miRNAs) targeting HDAC9 were predicted utilizing bioinformatics analysis. We then constructed the oxygen glucose deprivation (OGD) cell model and the middle cerebral artery occlusion (MCAO) rat model, and elucidated the expression of CCCTC binding factor (CTCF)/miR-383-5p/HDAC9. Targeting between miR-383-5p and HDAC9 was verified by dual-luciferase reporter assay and RNAi. After conducting an overexpression/knockdown assay, we assessed neuronal impairment and brain injury. We found that CTCF inhibited miR-383-5p expression via its enrichment in the promoter region of miR-383-5p, whereas the miR-383-5p targeted and inhibited HDAC9 expression. In the OGD model and the MCAO model, we confirmed that elevation of HDAC9 regulated by the CTCF/miR-383-5p/HDAC9 pathway mediated apoptosis induced by endoplasmic reticulum stress, while reduction of HDAC9 alleviated apoptosis and the symptoms of cerebral infarction in MCAO rats. Thus, the CTCF/miR-383-5p/HDAC9 pathway may present a target for drug development against ischemic brain injury.






Similar content being viewed by others
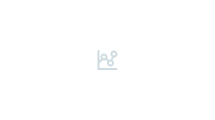
Data Availability
The datasets generated and analysed during the current study are available from the corresponding author on reasonable request.
References
Radak D, Katsiki N, Resanovic I, Jovanovic A, Sudar-Milovanovic E, Zafirovic S, Mousad SA, Isenovic ER (2017) Apoptosis and Acute Brain Ischemia in Ischemic Stroke. Curr Vasc Pharmacol 15(2):115–122. https://doi.org/10.2174/1570161115666161104095522
Liu X, Zhao S, Liu F, Kang J, Xiao A, Li F, Zhang C, Yan F et al (2014) Remote ischemic postconditioning alleviates cerebral ischemic injury by attenuating endoplasmic reticulum stress-mediated apoptosis. Transl Stroke Res 5(6):692–700. https://doi.org/10.1007/s12975-014-0359-5
Yang T, Sun Y, Li Q, Li S, Shi Y, Leak RK, Chen J, Zhang F (2020) Ischemic preconditioning provides long-lasting neuroprotection against ischemic stroke: The role of Nrf2. Exp Neurol 325:113142. https://doi.org/10.1016/j.expneurol.2019.113142
Mosch A, Ettl T, Mamilos A, Schreml S, Sporl S, Spanier G, Klingelhoffer C (2019) Physiological concentrations of denosumab enhance osteogenic differentiation in human mesenchymal stem cells of the jaw bone. Arch Oral Biol 101:23–29. https://doi.org/10.1016/j.archoralbio.2019.03.005
Alawieh A, Langley EF, Tomlinson S (2018) Targeted complement inhibition salvages stressed neurons and inhibits neuroinflammation after stroke in mice. Sci Transl Med 10(441):10.1126/scitranslmed.aao6459
Ejaz S, Emmrich JV, Sitnikov SL, Hong YT, Sawiak SJ, Fryer TD, Aigbirhio FI, Williamson DJ et al (2016) Normobaric hyperoxia markedly reduces brain damage and sensorimotor deficits following brief focal ischaemia. Brain 139(Pt 3):751–764. https://doi.org/10.1093/brain/awv391
Chamorro A, Dirnagl U, Urra X, Planas AM (2016) Neuroprotection in acute stroke: targeting excitotoxicity, oxidative and nitrosative stress, and inflammation. Lancet Neurol 15(8):869–881. https://doi.org/10.1016/S1474-4422(16)00114-9
Tymianski M (2011) Emerging mechanisms of disrupted cellular signaling in brain ischemia. Nat Neurosci 14(11):1369–1373. https://doi.org/10.1038/nn.2951
Kim HJ, Leeds P, Chuang DM (2009) The HDAC inhibitor, sodium butyrate, stimulates neurogenesis in the ischemic brain. J Neurochem 110(4):1226–1240. https://doi.org/10.1111/j.1471-4159.2009.06212.x
Wang B, Zhu X, Kim Y, Li J, Huang S, Saleem S, Li RC, Xu Y et al (2012) Histone deacetylase inhibition activates transcription factor Nrf2 and protects against cerebral ischemic damage. Free Radic Biol Med 52(5):928–936. https://doi.org/10.1016/j.freeradbiomed.2011.12.006
Chuang DM, Leng Y, Marinova Z, Kim HJ, Chiu CT (2009) Multiple roles of HDAC inhibition in neurodegenerative conditions. Trends Neurosci 32(11):591–601. https://doi.org/10.1016/j.tins.2009.06.002
Dietz KC, Casaccia P (2010) HDAC inhibitors and neurodegeneration: at the edge between protection and damage. Pharmacol Res 62(1):11–17. https://doi.org/10.1016/j.phrs.2010.01.011
Fessler EB, Chibane FL, Wang Z, Chuang DM (2013) Potential roles of HDAC inhibitors in mitigating ischemia-induced brain damage and facilitating endogenous regeneration and recovery. Curr Pharm Des 19(28):5105–5120. https://doi.org/10.2174/1381612811319280009
Pickell Z, Williams AM, Alam HB, Hsu CH (2020) Histone deacetylase inhibitors: a novel strategy for neuroprotection and cardioprotection following ischemia/reperfusion injury. J Am Heart Assoc 9(11):e016349. https://doi.org/10.1161/JAHA.120.016349
Ganai SA, Ramadoss M, Mahadevan V (2016) Histone Deacetylase (HDAC) Inhibitors - emerging roles in neuronal memory, learning, synaptic plasticity and neural regeneration. Curr Neuropharmacol 14(1):55–71. https://doi.org/10.2174/1570159x13666151021111609
Shein NA, Shohami E (2011) Histone deacetylase inhibitors as therapeutic agents for acute central nervous system injuries. Mol Med 17(5-6):448–456. https://doi.org/10.2119/molmed.2011.00038
Shi W, Wei X, Wang Z, Han H, Fu Y, Liu J, Zhang Y, Guo J et al (2016) HDAC9 exacerbates endothelial injury in cerebral ischaemia/reperfusion injury. J Cell Mol Med 20(6):1139–1149. https://doi.org/10.1111/jcmm.12803
Lu S, Li H, Li K, Fan XD (2018) HDAC9 promotes brain ischemic injury by provoking IkappaBalpha/NF-kappaB and MAPKs signaling pathways. Biochem Biophys Res Commun 503(3):1322–1329. https://doi.org/10.1016/j.bbrc.2018.07.043
Pei L, Meng S, Yu W, Wang Q, Song F, Ma L (2016) Inhibition of microRNA-383 ameliorates injury after focal cerebral ischemia via targeting PPARgamma. Cell Physiol Biochem 39(4):1339–1346. https://doi.org/10.1159/000447838
Perez-Rico YA, Barillot E, Shkumatava A (2020) Demarcation of topologically associating domains is uncoupled from enriched CTCF binding in developing zebrafish. iScience 23(5):101046. https://doi.org/10.1016/j.isci.2020.101046
Xu Y, Zhang Q, Yu S, Yang Y, Ding F (2011) The protective effects of chitooligosaccharides against glucose deprivation-induced cell apoptosis in cultured cortical neurons through activation of PI3K/Akt and MEK/ERK1/2 pathways. Brain Res 1375:49–58. https://doi.org/10.1016/j.brainres.2010.12.029
Negishi T, Ishii Y, Kyuwa S, Kuroda Y, Yoshikawa Y (2003) Primary culture of cortical neurons, type-1 astrocytes, and microglial cells from cynomolgus monkey (Macaca fascicularis) fetuses. J Neurosci Methods 131(1-2):133–140. https://doi.org/10.1016/j.jneumeth.2003.08.006
Tian T, Zeng J, Zhao G, Zhao W, Gao S, Liu L (2018) Neuroprotective effects of orientin on oxygen-glucose deprivation/reperfusion-induced cell injury in primary culture of rat cortical neurons. Exp Biol Med (Maywood) 243(1):78–86. https://doi.org/10.1177/1535370217737983
Zhang R, Wu Y, Xie F, Zhong Y, Wang Y, Xu M, Feng J, Charish J et al (2018) RGMa mediates reactive astrogliosis and glial scar formation through TGFbeta1/Smad2/3 signaling after stroke. Cell Death Differ 25(8):1503–1516. https://doi.org/10.1038/s41418-018-0058-y
Cheng X, Yang YL, Li WH, Liu M, Wang YH, Du GH (2020) Cerebral ischemia-reperfusion aggravated cerebral infarction injury and possible differential genes identified by RNA-Seq in rats. Brain Res Bull 156:33–42. https://doi.org/10.1016/j.brainresbull.2019.12.014
Chen J, Xuan Y, Chen Y, Wu T, Chen L, Guan H, Yang S, He J et al (2019) Netrin-1 alleviates subarachnoid haemorrhage-induced brain injury via the PPARgamma/NF-KB signalling pathway. J Cell Mol Med 23(3):2256–2262. https://doi.org/10.1111/jcmm.14105
Markus HS, Makela KM, Bevan S, Raitoharju E, Oksala N, Bis JC, O'Donnell C, Hainsworth A et al (2013) Evidence HDAC9 genetic variant associated with ischemic stroke increases risk via promoting carotid atherosclerosis. Stroke 44(5):1220–1225. https://doi.org/10.1161/STROKEAHA.111.000217
Lu Y, Tan L, Wang X (2019) Circular HDAC9/microRNA-138/Sirtuin-1 pathway mediates synaptic and amyloid precursor protein processing deficits in Alzheimer’s disease. Neurosci Bull 35(5):877–888. https://doi.org/10.1007/s12264-019-00361-0
Xu G, Li N, Zhang Y, Zhang J, Xu R, Wu Y (2019) MicroRNA-383-5p inhibits the progression of gastric carcinoma via targeting HDAC9 expression. Braz J Med Biol Res 52(8):e8341. https://doi.org/10.1590/1414-431X20198341
Watson LA, Wang X, Elbert A, Kernohan KD, Galjart N, Berube NG (2014) Dual effect of CTCF loss on neuroprogenitor differentiation and survival. J Neurosci 34(8):2860–2870. https://doi.org/10.1523/JNEUROSCI.3769-13.2014
Hirayama T, Tarusawa E, Yoshimura Y, Galjart N, Yagi T (2012) CTCF is required for neural development and stochastic expression of clustered Pcdh genes in neurons. Cell Rep 2(2):345–357. https://doi.org/10.1016/j.celrep.2012.06.014
Sams DS, Nardone S, Getselter D, Raz D, Tal M, Rayi PR, Kaphzan H, Hakim O et al (2016) Neuronal CTCF Is necessary for basal and experience-dependent gene regulation, memory formation, and genomic structure of BDNF and Arc. Cell Rep 17(9):2418–2430. https://doi.org/10.1016/j.celrep.2016.11.004
Kernohan KD, Vernimmen D, Gloor GB, Berube NG (2014) Analysis of neonatal brain lacking ATRX or MeCP2 reveals changes in nucleosome density, CTCF binding and chromatin looping. Nucleic Acids Res 42(13):8356–8368. https://doi.org/10.1093/nar/gku564
Prickett AR, Barkas N, McCole RB, Hughes S, Amante SM, Schulz R, Oakey RJ (2013) Genome-wide and parental allele-specific analysis of CTCF and cohesin DNA binding in mouse brain reveals a tissue-specific binding pattern and an association with imprinted differentially methylated regions. Genome Res 23(10):1624–1635. https://doi.org/10.1101/gr.150136.112
Yuan Y, Guo Q, Ye Z, Pingping X, Wang N, Song Z (2011) Ischemic postconditioning protects brain from ischemia/reperfusion injury by attenuating endoplasmic reticulum stress-induced apoptosis through PI3K-Akt pathway. Brain Res 1367:85–93. https://doi.org/10.1016/j.brainres.2010.10.017
Wu CX, Liu R, Gao M, Zhao G, Wu S, Wu CF, Du GH (2013) Pinocembrin protects brain against ischemia/reperfusion injury by attenuating endoplasmic reticulum stress induced apoptosis. Neurosci Lett 546:57–62. https://doi.org/10.1016/j.neulet.2013.04.060
Su Y, Li F (2016) Endoplasmic reticulum stress in brain ischemia. Int J Neurosci 126(8):681–691. https://doi.org/10.3109/00207454.2015.1059836
Xin Q, Ji B, Cheng B, Wang C, Liu H, Chen X, Chen J, Bai B (2014) Endoplasmic reticulum stress in cerebral ischemia. Neurochem Int 68:18–27. https://doi.org/10.1016/j.neuint.2014.02.001
Shu Q, Fan H, Li SJ, Zhou D, Ma W, Zhao XY, Yan JQ, Wu G (2018) Protective effects of Progranulin against focal cerebral ischemia-reperfusion injury in rats by suppressing endoplasmic reticulum stress and NF-kappaB activation in reactive astrocytes. J Cell Biochem 119(8):6584–6597. https://doi.org/10.1002/jcb.26790
Zeng Z, Huang N, Zhang Y, Wang Y, Su Y, Zhang H, An Y (2020) CTCF inhibits endoplasmic reticulum stress and apoptosis in cardiomyocytes by upregulating RYR2 via inhibiting S100A1. Life Sci 242:117158. https://doi.org/10.1016/j.lfs.2019.117158
Author information
Authors and Affiliations
Contributions
JS designed the study. QH collated the data. WJL and JML carried out data analyses and produced the initial draft of the manuscript. XCC revised the figures. JYZ and SRX contributed to drafting the manuscript. All authors have read and approved the final submitted manuscript.
Corresponding authors
Ethics declarations
Ethics Approval
This study was approved by the Institutional Review Board of The First Affiliated Hospital of Soochow University.
Consent to Participate
Not applicable.
Consent for Publication
Not applicable.
Conflict of Interest
the authors declare no competing interests.
Additional information
Publisher’s Note
Springer Nature remains neutral with regard to jurisdictional claims in published maps and institutional affiliations.
Supplementary Information

SUPPLEMENTARY FIGURE 1
(A) Flow cytometry of apoptosis of neurons upon oe-CTCF or si-CTCF treatment. (B) The apoptosis rate detected by flow cytometry upon sh-CTCF or miR-383-5p inhibitpr treatment. (PNG 336 kb)
ESM 1
(DOCX 20 kb)
Rights and permissions
About this article
Cite this article
Shen, J., Han, Q., Li, W. et al. miR-383-5p Regulated by the Transcription Factor CTCF Affects Neuronal Impairment in Cerebral Ischemia by Mediating Deacetylase HDAC9 Activity. Mol Neurobiol 59, 6307–6320 (2022). https://doi.org/10.1007/s12035-022-02840-4
Received:
Accepted:
Published:
Issue Date:
DOI: https://doi.org/10.1007/s12035-022-02840-4