Abstract
The knitted cap was the ubiquitous and most visible garment men wore throughout early modern society, from apprentices to royals. Documentary evidence also suggests that red was a conventional color for specific garments in the sixteenth century, including knitted caps. However, most knitted caps in museum collections are now a muddy brown characteristic of archaeological textiles, and their original color has seldom been studied. Considering the potential of microspectrofluorimetry for analyzing dyes in ancient textiles and manuscript illuminations, this technique was tested on six caps dating from the fifteenth to sixteenth centuries examined in the Knitting in Early Modern Europe research project. The colors are in good preservation condition in two samples, whereas the others show extensive degradation. The emission and excitation spectra acquired allowed the identification of the similarities and differences between samples, grouping them into different dye sources such as cochineal-based and madder-based (RED1 and RED2, respectively). For the coif cap and split-brimmed cap in good condition, analysis through HPLC–MS confirmed the sources of the dyes as Rubia tinctorum and cochineal. It also disclosed the source for another coif cap as Kermes vermilio (RED4). The other knitted items are possibly madder-based (RED3), but HPLC–MS did not allow confirmation of the madder source. The continuing development of a database of excitation and emission spectra acquired from historical textiles, such as the knitted caps, will support the identification of dye sources and specific formulations. Although this technique demands a comprehensive database of references for comparison with the complex signals identified, it allows for rapid spectra acquisition, providing tremendously valuable information.
Similar content being viewed by others
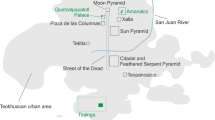
Early modern knitted textiles
The knitted cap
The ubiquitous and most visible knitted garment worn by men throughout early modern society from apprentices to royals was the knitted cap. They were manufactured by cappers and supplied by haberdashers, who also sold knitted stockings often described by color whereas the caps rarely were [1]. However, documentary sources suggest that caps were dyed: for example, there are 78 “colored” caps and 36 “uncolored” caps in the 1561 inventory of capper Roger Yelfe of Worcester, England [2]. A book of instructions from 1548 gives instructions for dyeing caps black, red, and scarlet [3]. Martin Luther, despite wearing a black knitted cap in his iconic portrait, actually wore a red one most of the time as did many school pupils [4, 5].
Documentary evidence also suggests that red was a conventional color for garments worn close to the body in the sixteenth century, partly for the prophylactic properties it was thought to provide [6]. A study of buried fabrics investigated the role of dyestuffs in the decomposition of textiles. There were indications that madder has a preservative effect on wool fibers and that it easily migrates to surrounding textiles during burial [7]. This suggests that knitted items dyed with madder may be better preserved than those treated with other dyestuffs and that items that were originally undyed may be contaminated by their near neighbors in the ground.
Most extant knitted caps in museum collections are now a muddy brown characteristic of archaeological textiles. Among the one hundred knitted caps, cap fragments, and cap linings examined in the Knitting in Early Modern Europe (KEME) study [8], many appeared red-brown either to the naked eye or under magnification (see Fig. 1). It is challenging to describe color accurately without recourse to a specific reference system such as CIELAB or Munsell. Only some of these caps were subject to this examination owing to the limited facilities and time available. In contrast, other categories of knitted caps are more yellow or darker brown. The red-brown caps were chosen for analysis because of previous work showing knitted red archaeological material yielded evidence of dyeing [9]. A total of 31 red-brown items were sampled taking care not to compromise the knitted structure according to a protocol drawn up in collaboration with curators and conservators at the Museum of London (see “Experimental” section, Table 3). A subset of six samples were selected from the 31 identified as red-brown.
The six samples under investigation here are from a variety of museum collections (see Table 1). Three (inventory numbers 742, 1981.10 and D10318) benefit from detailed information about their provenance but the other three (inventory numbers 22387, 22389, and 22390) do not because they were excavated more than a hundred years ago before conventional archaeological context recording began. However, a newspaper dated 1904 reported discoveries in the peat soil at Moorfields in London from which they all come: “the colours of these caps are very diverse; some are black, some are light, some a dark brown, some murrey (a kind of red), and others a distinct crimson… It is somewhat curious to remark that the murrey and reds have kept their colours better than the browns” [10].
The ground yarn of all the knitted items is paler than the napped surface which was produced by fulling and finishing it. These were the processes of agitating the knitted fabric when wet, and then raising and trimming the surface so that the knitted loops were compacted and obscured [11]. The caps were dyed after fulling so that sometimes the inner core of the yarn was still a natural sheep’s color. A surviving lining at the Museum of London has an unusually rich purple-red surface although the ground yarn is brown showing the fulled nap to have retained the dye (inventory number A6346) [12]. Unfortunately, it was not suitable for sampling. One of the sampled caps which is not recorded as archaeological but as part of a costume preserved for its commemorative value in the sixteenth century is of a striking bright red color. It was catalogued at the Bernisches Historisches Museum in 1884 as a velvet cap (inventory number 742) such was the quality of its fulled surface [13].
How to identify the original color of the knitted caps
Knitted garments such as colored caps and stockings are found in early modern inventories, depicted in portraits, and referred to in contemporary dyeing manuals [3, 14, 15]. Several studies have demonstrated the usefulness of different techniques for investigating early modern material dating from the fifteenth and sixteenth centuries [16,17,18,19,20,21]. An unidentified fragment of knitted fabric dated 1400 to 1450 was analyzed and found to have been dyed with kermes “using spectroscopic and chromatographic techniques” although the specific techniques used were not identified [22]. A sixteenth-century knitted cap was investigated for dyes and mordants using Fourier transform infrared spectroscopy (FTIR) and thin layer chromatography (TLC); the latter indicated that the caps were dyed and mordanted but no mention was made of the dyestuffs or the mordants used [23]. Two samples from a seventeenth-century knitted cap from Dava Moor, Scotland (inventory number H.NA 478) in the collection of National Museums Scotland showed evidence of indigotin as a result of high-performance liquid chromatography with diode array detection (HPLC–DAD) [9].
This shows the need for new approaches for studying the original color of these knitted garments. The potential of microspectrofluorimetry for analyzing dyes in ancient textiles and manuscript illuminations suggested its usefulness in testing this set of six caps dating from the fifteenth to the sixteenth centuries (Table 1). The colors are in good preservation condition in two samples, whereas the others show signs of extensive degradation. Excitation and emission spectra were acquired and compared to an existing database of reference spectra, considering the bands' maxima and overall shapes. It was possible to assign the dye source to five of the six samples based on this comparison and the assumption that cochineal or madder were the most likely dyes in the garments. The assignment was complemented by HPLC–MS (high-performance liquid chromatography coupled with mass spectrometry), which also found the presence of secondary dyes, and identified one sample as being possibly dyed with kermes.
In-depth research into natural dyes’ formulations
Historical written sources commonly mention dye-mordant complexes for red and yellow dyes, while vat dyeing methods are typical for indigo blues [24, 25]. The primary sources for natural red organic colorants, either identified in artworks or described in medieval treatises and recipe books in European civilization, were madder (Rubia spp.), lac dye (Kerria spp.,), cochineal (Porphyrophora spp., Dactylopius spp.), and kermes (Kermes vermilio). All are composed of anthraquinones hydroxy derivatives, with the first being derived from the roots of a plant, while the other three are animal-based (see Fig. 2) [24, 25].
Dyes are water-soluble substances, which can be used in three different ways: (i) as a complex dye with a metal ion, a mordant (the most common being aluminum in the form of alum, which forms a bridge between the textile fiber and the dye); (ii) as a vat dye, which works by converting a dye which is not soluble, into a water-soluble form, and precipitating it inside the fiber; (iii) as a direct dye, which binds directly to the textile.
Natural dyes are very complex materials, and organic colors are not all prepared alike. Differences in pH, extraction solutions and time, temperature, mordant types, and concentrations, and the addition of color modulators, or other agents, have a significant impact on the final color obtained. Hence, the formulations, i.e. the recipes, can reveal much about the history of technology in the preparation of these colors.
Molecular fluorescence as a tool for the study of natural dyes
Microspectrofluorimetry for dye analysis has been explored for the past 15 years, demonstrating the importance of this technique [26,27,28,29,30,31]. It offers high sensitivity and selectivity, good spatial resolution, and fast data acquisition; it can also be used in situ without any contact with the sample or artwork.
The simultaneous acquisition of emission and excitation spectra in the same microspot is essential for identifying ancient dyes. The corrected excitation spectrum can be identical to the absorption spectrum, and an emission spectrum reflects the probability of transition from electronic states and the associated vibrational levels. For this reason, a well-resolved emission spectrum can be characteristic of a compound. However, historical dyes usually exhibit broad bands due to the environment of their discovery context and their state of preservation. Thus, these broad bands make it challenging to acquire a unique fingerprint that allows unequivocal identification of the molecule, as in Raman spectra. Yet, these broad bands encompass essential information about the formulations behind the colors, which have been proven using a comprehensive database of references for the most common dyes used in Europe in the past, such as madder and cochineal. Another relevant factor in identifying ancient dyes is related to the amount of light emitted, measured by the quantum yield of fluorescence. The most common natural dyes used in the past can be considered weak emitters as is the case with alizarin, or moderate emitters such as purpurin [27]. The information acquired with microspectrofluorimetry has been combined with other techniques, such as SERS or HPLC–DAD–MS, to provide conclusive dye identification [26,27,28,29,30,31,32,33,34,35], see Fig. 3
The source used for dyeing the textile, the recipe, and its preservation condition can be identified using molecular excitation and emission spectra, together with a comprehensive database of references and a chemometrics approach. It is important to validate this information further using complementary techniques such as HPLC–DAD–MS
In the case of Rubia tinctorum, following extraction and dyeing, the main dyes found in the textiles are complexes of alizarin and purpurin with aluminum ions. As the purpurin complex emits ten-fold more than alizarin, the emission and excitation spectra are usually dominated by the purpurin-Al3+-complex. Therefore, the assignment to madder is based on the fact that it was one of the most common sources of dye in the medieval and early modern eras. In the case of cochineal-based dyes, the main chromophore is the Al3+-carminic acid complex, which, depending on the animal source, extraction conditions, preservation conditions, and other factors, may be pinpointed with chemometrics. However, the extensive database required for such accuracy is still under construction, and in this paper, it will be identified as cochineal-based.
Results and discussion
The six samples ranged from a few individual fibers (inventory number 742) to small fragments of approximately 0.9 cm × 0.9 cm (inventory number 22390). Some were of dense material (inventory number 1981.10) while others were fluffy (inventory number D10318A) (Table 1 and Fig. 4).
Microspectrofluorimetry
Although some samples had the muddy brown appearance characteristic of archaeological textiles, two were of a beautiful bright red (samples 742 and 1981.10), indicating a good state of preservation. After analysis by microspectrofluorimetry, the data was analyzed, and the signals were grouped into four different red types: RED1 to 4. The interpretation of the spectral data took into account the maxima of excitation and emission, the intensities of the spectra and the spectral shape. The signals obtained can be found in Additional file 1: Figure S1.
In the two red samples, RED1 and RED2, two different dye sources were identified, madder and cochineal-based (Figs. 5 and 6, and Table 2). Sample 1981.10, RED1, shows excitation maxima at 515 nm and 545 nm, with an emission maximum at 585 nm and a shoulder at 565 nm (Fig. 6 and Table 1). Sample 742, RED2, has an excitation maximum at 522 and 555 nm, with an emission maximum at 575 nm and a shoulder at 610 nm (Fig. 6 and Table 2).
Excitation (left spectrum) and emission (right spectrum) of both purpurin and carminic acid aluminum references prepared at a concentration of 10–4 M in methanol:water (70:30, v/v) with Al3+ (× 100) at pH 2.4 (purpurin) and pH 3.5 (carminic acid), applied in filter paper. For more details see [27]
Excitation (left spectrum) and emission (right spectrum) of the four types of red dyes found in the six samples, compared with known reds identified in Andean textiles: RED1 (1981.10, black) and MFA 21.2581 (magenta); RED2 (742, black) and MFA 10.267 (red); RED3 (22389, black) and MFA 31.499 (magenta); and RED4 (D10318). The colors in the spectra match the aluminum ion complexes of purpurin (magenta) and cochineal (red)
Three of the more degraded samples (22387, 22389 and 22390) showed the same excitation (505 nm) and emission (560 nm) spectra, and were grouped as RED3. The other muddy brown sample D10318, RED4, shows an excitation maximum of 545 nm, with an emission maximum of 565 nm and a shoulder at 585 nm (Fig. 5 and Table 2). The shift in both excitation and emission spectra indicates that most samples are not likely to be from the same dye source, although probably from the same molecular family of anthraquinones.
By comparing these results with a database of references (pure compounds complexed with aluminum, Al3+), RED1 appears to be a match with a purpurin–aluminum complex (madder), while RED2 may be a carminic–aluminum complex (cochineal) (see Figs. 5 and 6). These signals were compared with the data acquired from red Andean textiles belonging to the Museum of Fine Arts in Boston (MFA-Boston), which are described below [28, 32, 34].
Samples D10318, 22387, 22389 and 22390 are somewhat more complicated to identify. The extensive degradation caused by their archaeological conditions has left a black coating on the fibres making it hard to find an optimal location for analysis. Nevertheless, samples 22387, 22389 and 22390 are considered to be madder-based but more degraded. This is shown by the intensity of their spectra, which are much lower than RED1, 1981.10. It was not possible to provide a suggested attribution for sample D10318 because of the lack of reference materials providing a match with its fluorescence signals having been compared with aluminum ion complexes of madder, cochineal and kermes (Additional file 1: Figure S2).
RED1 and 2, samples 1981.10 and 742, appear to be in a state of good preservation. Not only because they maintain a visible red and pink color respectively, but because the spectral shapes are similar to those found in the Andean textiles and in the pure single molecule reference material. The intensity signals, although lower than those reported for the Andean textiles and for the reference materials, are still much higher than those samples considered to be in a poor state of preservation (22387, 22389 and 22390).
The comparison between the knitted cap samples and data acquired from the Andean textiles [28, 32, 34], which have been fully characterized by HPLC–DAD and SEM, corroborates the attributions. A previous collaboration with MFA-Boston permitted the analysis of a collection of samples from 76 reds from Andean textiles dating from 200 BCE to the fifteenth century CE which were created by Paracas, Nasca, Wari Huari, Chancay, and Lambayeque cultures, and which are in an excellent state of preservation. Previous studies with SEM–EDX screening confirmed the use of aluminum ions as a mordant [28, 32, 34]. Microspectrofluorimetry was used, together with HPLC–DAD in selected samples, to enable a conclusive identification of carminic acid in Chancay textiles (1000 to 1476), and purpurin and pseudo-purpurin in Paracas (200 BCE to 300 CE) and Nasca textiles (0 to 300 CE) [28, 32, 34]. Represented in Fig. 6 are samples MFA 21.2581 (s6), and MFA 31.499 (s1), which were both identified as pseudo-purpurin/purpurin complexes (such as those found in madder reds) and MFA 10.267 (s1), a carminic-acid complex (cochineal). The match between these samples and those from the knitted caps is quite close, with a few exceptions in the excitation spectra. In particular, RED3, despite its degradation and poor signal-to-noise ratio, presents a very good match with sample MFA 31.499 (s1), identified as a pseudo-purpurin and purpurin complex. By comparing the spectral shape and, in particular, the emission maxima, a match between the two is suggested.
There are several species of madder plants, all with different proportions of alizarin, purpurin, and pseudo-purpurin. Alizarin is considered the main compound present in the roots of Rubia spp., while other species such as Relbunium L. (species used in the Andean textiles), have purpurin and pseudo-purpurin in higher concentration. However, as discussed, alizarin is a very weak emitter, emitting 10× less than purpurin [27, 28, 32]. Therefore, the spectra obtained for the knitted caps are dominated by the Al3+-purpurin-based complexes. Considering the high proportion of purpurin and pseudo-purpurin in Andean textiles, this would explain the lower intensity for RED1 and RED2, which were possibly dyed with madder. This also explains the shifts in the excitation spectra.
High-performance liquid chromatography coupled with mass spectrometry
HPLC–MS was used to confirm the spectrofluorimetry attributions. A soft-extraction method was used to prevent the degradation of the molecules on approximately 0.3 mg of fibers [27, 28, 36]. For more details see HPLC–MS in “Experimental” section.
This corroborated the identifications for the red samples in good preservation condition: 1981.10, RED1, by the presence of alizarin and purpurin and 742, RED2, by the presence of carminic acid [37,38,39]. In two of the more degraded samples 22387 and 22390, RED3, alizarin and purpurin were not detected; in 22387, xanthopurpurin was the major dye identified; in 22389, traces of rubiadin were detected. This is probably due to their poor preservation condition or because the dye has leached into them from other items in the ground. Further studies using an extended database of aged samples analyzed by microspectrofluorimetry will be necessary to confirm madder as the dye source.
For sample D10318, it was possible to identify kermes as the main dye pointing to the use of Kermes vermilio as the animal source (Table 3). The presence of kermes in sample D10318 is very interesting and may provide clues to the social and economic status of the cap’s owner and its dating. Kermes was an expensive dye in the sixteenth century which became less common with the rise of cochineal in the seventeenth century.
There were minute amounts of flavokermesic and xanthopurpurin on sample 742, indicating a formulation in which madder and kermes/lac dye were added to the dye bath. This could also account for the shift in the excitation spectrum for sample 742 in relation to the cochineal-aluminum reference and the Andean textile MFA 10.267 (s1). The HPLC–MS figures can be found in Additional file 1.
HPLC–MS analysis also enabled the identification of what may be trace amounts of rubiadin in sample 22387, RED3. Xanthopurpurin and rubiadin are molecules present in many madder species [39]. Understanding why these are the only identifiable markers for madder in these samples is an ongoing study.
The identification of xanthopurpurin resulted in MS2 fragmentation signals m/z 202 and m/z 195, which accords with previous references [37, 39]. However, other fragmentation signals were also found suggesting the presence of different isomers or other compounds, especially in sample D0318.
Conclusions
Microspectrofluorimetry was used to analyze six samples from a selection of 31 red-brown knitted caps, cap fragments, and cap linings examined in the Knitting in Early Modern Europe study [8]. The emission and excitation spectra acquired allowed the identification of the similarities and differences between samples, grouping them into different dye sources such as cochineal-based and madder-based. Analysis through HPLC–MS confirmed the sources of the dyes, RED1 to Rubia tinctorum and RED2 to cochineal. It also disclosed the source for RED4 as Kermes vermilio. This is an exciting result, as it may shed new light on the status of the cap’s owner since kermes was a costly dye. On the other hand, for the more degraded samples included in RED3, the signals did not yet allow the confirmation of madder as the source.
Microspectrofluorimetry can be used on an entire dataset, with the attribution being confirmed by the use of HPLC–MS, which is performed only for selected samples, instead of extracting and analyzing hundreds of samples. The samples that were not extracted and analyzed by HPLC–MS are still stored, allowing them to be studied further by analytical techniques.
An additional observation is that in the absence of a scientific archaeological recording of an excavation, newspaper reports or other contemporary literature may provide credible grounds for understanding the condition of textiles when they were first unearthed. The newspaper report of 1904 is the only information available about the more degraded samples RED3 (22387, 22389, and 22390). They were part of a group for which “the murrey and reds have kept their colours better than the browns”. These colors are not apparent to the naked eye today, yet the analysis reported here corroborates that the three caps were dyed red with madder or were contaminated with madder while buried. This points to potential new sources of information about textiles for which little is known about their provenance, based on an extended database of aged and unaged references. In the future, this may lead to chronological and location details for the manufacture of the textiles, as has been proven in previous works on the study of natural dyes in medieval illuminations [31].
Experimental
Materials
Spectroscopic or equivalent grade solvents and Millipore-filtered water were used during all the experimental work. Qualitative filter paper from Filter Lab was used. Each chromophore (purpurin, and carminic acid) was characterized at different pHs (according to their acid–base equilibrium) and complexed with Al3+, both in homogeneous media (aqueous solution) and heterogeneous media (filter paper). Solutions were prepared in methanol:water (70:30, v/v), at 5 × 10–4 M. Adjustment of pH values was carried out with the addition of hydrochloric acid (1 M and 0.1 M) and sodium hydroxide (1 M and 0.1 M). Complexation of molecules with Al3+ (where Al3+ is present × 100 in respect to the molecule) was performed with the addition of AlCl3 (0.1 M). Six drops of each solution were then applied on filter paper with a micro-pipette (10 µl). Three replicates for each reference were prepared, and analyses were generally carried out on the same day (or the day after) after application on filter paper.
Data acquired in these reference materials compose a database, which is compared with the case studies. These references are thoroughly analyzed by microspectrofluorimetry, FORS, absorbance, infrared, SERS and HPLC–DAD.
Andean textiles references
A previous collaboration with the Museum of Fine Arts in Boston (MFA-Boston) allowed for the analysis of a collection of samples of 76 reds from Andean textiles, dated from 200 BCE to the fifteenth century CE and created by the Paracas, Nasca, Wari Huari, Chancay and Lambayeque cultures. The samples used for comparison in this study were: MFA 21.2581 [sample 6, Paracas 200 BCE to 200 CE (skirt)], MFA 10.267 (sample 1, Lambayeque A.D. 1000-1476 (tapestry strip with figures and large birds), and MFA 31.499 (sample 1, Paracas, 100 BCE to 100 CE). The HPLC–DAD–MS, SEM–EDS, and fluorescence data acquired in these samples are part of the database of historical materials to be further compared with case studies, if necessary. For more information on these samples and their characterization, see [26,27,28, 30,31,32, 34].
The knitted caps
Six caps were selected from the one hundred knitted caps, cap fragments, and cap linings examined in the Knitting in Early Modern Europe study [38]. The inventory number of the caps to which the samples belong are D10318, (Nationalmuseet Denmark), 742 (Bernisches Historisches Museum), 22387, 22389, 22390 (Museum of London) and 1981.10 (Historisches Museum Basel).
Sampling
Permission for sampling the caps in the KEME study was hard won. Museums are reluctant to permit destructive sampling and especially so in textiles perceived to be vulnerable to cutting, such as knitting, which is often thought to unravel easily. However, fulled knitted fabric is much more stable than unfulled knitted fabric since the loops of the knitting are meshed together to form a textile that will withstand napping (raising the hairs to create a surface texture similar to velvet in the best examples), shearing and cutting. Sampling was achieved by developing a protocol for examining the knitted items and determining whether they were suitable for sampling and, if so, where. This was devised in collaboration with curatorial and conservation staff at the Museum of London (see Table 4).
Stereomicroscope photographs
The photographs were taken using a Leica KL 1500 LCD microscope, (7.1× to 115× objective) and a Leica Digilux digital camera, with external illumination via optical fibers.
Molecular fluorescence in the visible
Fluorescence excitation and emission spectra were recorded with a Jobin–Yvon/Horiba SPEX Fluorog 3-2.2 spectrofluorometer coupled to an Olympus BX51M confocal microscope, with spatial resolution controlled by a multiple-pinhole turret, corresponding to a minimum 2 μm and maximum 60 μm spot, equipped with a 50× objective. Beam-splitting is obtained with standard dichroic filters mounted at a 45° angle in a two-place filter holder. For a dichroic filter of 600 nm, excitation may be carried out up to about 590 nm, and emission collected after 610 nm. Optimization of the signal was performed daily for all pinhole apertures through mirror alignment, following the manufacturer’s instructions, using a rhodamine standard (or other adequate references). For this study, one set of dichroic filters was employed: 500 nm and 600 nm, exciting at 490 nm and reading the emission signal at 610 nm, respectively. For the study of the Andean textiles two sets of dichroic filters were used: 500 nm and 570 nm for purpurin-based textiles (exciting at 490 nm and reading the emission at 590 nm), and 525 nm and 600 nm for cochineal-based textiles (exciting at 515 nm and reading the emission at 610 nm), see Table 5.
Emission from a continuous 450-W xenon lamp, providing an intense broad spectrum from the UV to near-IR, is directed into a double-grating monochromator, and spectra are acquired after focusing on the sample (eye view) followed by signal intensity optimization (detector reading). The pinhole aperture that controls the measurement area is selected based on the signal-to-noise ratio. In this work, due to very weak signals, a 30-μm spot was used (pinhole 8) with the following slits set: emission slits = 3/3/3 mm (6 nm bandpass) and excitation slits = 5/3/0.8 mm (final bandpass of 2 nm). Emission and excitation spectra were acquired on the same spot.
When needed the spectra were normalized by area. When comparing spectra acquired in different samples, one must take into account 4 different signals: the maxima of excitation and emission, the signal-to-noise ratio, the intensity of the signals, and the shape.
HPLC–MS
The dyes from the textiles were extracted by placing them in a microtube, circa 0.3 mg of textile with a 400 µL solution of oxalic acid (0.2 M):methanol:acetone:water (0.1:3:3:4, v:v) at 60 °C for 30 min. The solution was left to evaporate, and the residues were then dissolved in 400 µL of methanol/water, 7:3 (v/v); the tubes were centrifuged, and the upper 25 µL of the solution was removed for analysis.
All samples were analyzed by HPLC-ESI-Q-Orbitrap-MS. The analyses were performed in a HPLC Vanquish (Thermo Fischer Scientific, Bremen, Germany) coupled to an Orbitrap Exploris 120 mass spectrometer (Thermo Fischer Scientific, Bremen, Germany) controlled by Orbitrap Exploris Tune Application 2.0.185.35 and Xcalibur 4.4.16.14. The capillary voltage of the electrospray ionization source (ESI) was set to 3.5 kV and 2.5 kV for positive and negative mode. SRM mode was used for all possible compounds. The capillary temperature was 300 °C. The sheath gas, auxiliary gas, and sweep gas flow rates were at 50, 10, 1 (arbitrary unit as provided by the software settings). The resolution of MS scan was 60,000. Data-dependent MS/MS was performed on HCD using nitrogen as gas with collision energy settings of 30 V. The m/z range were 100–1000 Da.
The stationary phase was an Agilent Poroshell 120 CS-C18 column (150 × 4.6 mm i.d., 2.7 µm) at 35 °C. The mobile phases were composed by solvent A, 100% (v/v) methanol, and solvent B, 0.3% (v/v) formic acid. The flow rate was 0.30 mL/min, the injection volume was 0.15 mL, and the gradient method started with an isocratic 7% A gradient, followed by a linear gradient ranging from 7% A to 15% A in 8 min, then reaching 75% A in 17 min, 80% A in 5 min, and 95% A in 15 min.
Availability of data and materials
All data generated during this study are either included in this published article or available from the corresponding authors upon reasonable request.
Abbreviations
- HRMS/MS:
-
High-Resolution Mass Spectrometry with high-resolution mass spectrometric detection
- KEME:
-
Knitting in Early Modern Europe
- µRaman:
-
Micro-Raman spectroscopy
- MFA:
-
Museum of Fine Arts, Boston
- SERS:
-
Surface-enhanced Raman spectroscopy
- UV:
-
Ultraviolet
- UV–VIS:
-
UV–Visible
- SEM:
-
Scanning electron microscopy
References
Staniland K. Thomas Deane’s shop in the royal exchange. In: Saunders A, editor. The royal exchange, vol. 52. London: London Topographical Society Publication; 1997. p. 59–67.
Dyer A. Inventories of Worcester tradesmen in Miscellany II, new series 5. Worcestershire Historical Society; 1967. p. 19–21.
Rosetti G. The Plictho: instructions in the art of the dyers which teaches the dyeing of woolen cloths, linens, cottons, and silk by the great art as well as by the common… Edelstein S, Borghetty H, editors. Cambridge: MIT Press; 1548/1969, p. 105–106.
Rublack U. Dressing up: cultural identity in renaissance Europe. Oxford: Oxford University Press; 2010. p. 97.
Huggett J, Mikhaila N. Small boys and youths. In: Malcolm-Davies J, editor. The Tudor child: clothing and culture 1485 to 1625. Lightwater: Fat Goose Press; 2013. p. 34.
Borde A. A dietary of health. London: Thomas Colwell; 1542.
Ringgaard M, Scharff A. The impact of dyes and natural pigmentation of wool on the preservation of archaeological textiles. In: Andersson Strand E, Gleba M, Mannering U, Munkholt C, Ringgaard M, editors. North European Symposium for Archaeological Textiles X. Oxford: Oxbow Books. 2010; p. 221–224, 221.
Malcolm-Davies J. Knitting in Early Modern Europe (KEME); 2023. https://kemeresearch.com. Accessed 19 Feb 2023.
Surowiec I, Quye A, Trojanowicz M. Liquid chromatography determination of natural dyes in extracts from historical Scottish textiles excavated from peat bogs. J Chrom A. 2006;1112:209–17.
Anon. Clothes relics of the Tudor period. Linlithgow Gazette; 1904. p. 7.
Malcolm-Davies J. An Early Modern mystery: a pilot study of knitting, napping and capping. Arch Tex Rev. 2016;58:57–74.
Malcolm-Davies J, Mikhaila N. The Typical Tudor: reconstructing everyday 16th century dress. Lightwater: Fat Goose Press; 2023. p. 163.
Maeder E. The restoration of a 16th century Swiss mercenary soldier’s costume in the Historical Museum, Bern, Switzerland. In: Pertegato F, editor. Conservation and Restoration of Textiles: International Conference, Como. Milan: CISST; 1980. p. 224–8.
Emmison F. Essex wills: the archdeaconry courts 1583–1592, publication, vol. 101. Chelmsford: Essex Record Office, Publication; 1989. p. 5.
Van Heemskerck M. Portrait of a Young Student, Museum Boijmans Van Beuningen, Rotterdam, Netherlands; 1531. www.boijmans.nl/en/collection/artworks/2821/portrait-of-a-young-student. Accessed 3 Feb 2023.
Peacock D. Dyeing Winchcombe kersies and other kersey cloth in 16th century Newbury. Tex Hist. 2006;37(2):187–202.
National Archives of Scotland, PA5/6(1), f. 42r-48r, Records of the Parliament of Scotland to 1707, 1458/3/14. https://www.rps.ac.uk/trans/1458/3/12. Accessed 31 Mar 2020.
Vanden Berghe I, Gleba M, Mannering U. Towards the identification of dyestuffs in early Iron Age Scandinavian peat bog textiles. J Arch Sci. 2009;36:1910–21.
Dyer J, Tamburini D, O’Connell ER, Harrison A. A multispectral imaging approach integrated into the study of Late Antique textiles from Egypt. PLoS ONE. 2018;13(10):1–33.
Ballard M, Mina L, Hacke M, Speakman R. Portable and Micro-XRF: non-destructive approaches to screen textiles and identify[ing] Mordants, poster presented at the 30th annual Dyes in History and Archaeology meeting, October 13–14, Derby, UK; 2011.
Degano I, Ribechini E, Modugno F, Colombini M. Analytical methods for the characterization of organic dyes in artworks and in historical textiles. App Spec Rev. 2009;44(5):363–410. https://doi.org/10.1080/05704920902937876.
Walton P. The textiles. In: Harbottle B, Ellison M, editors. An excavation in the castle ditch, Newcastle upon Tyne, 1974–76, Archaeologia Aeliana, 1981; series 5, vol 9: p. 190–228, 198.
Botticello J. The conservation of two Tudor knitted woollen flat caps, unpublished undergraduate thesis, Camberwell College, London, 2003, appendix IV.
Cardon D. Natural dyes: sources, tradition, technology and science. London: Archetype Publications; 2007. (ISBN: 190498200x).
Kirby J, van Bommel M, Verhecken A. Natural colorants for dyeing and lake pigments: practical recipes and their historical sources. London: Archetype Publications Ltd in association with CHARISMA; 2014. (ISBN: 78-1909492158).
Melo MJ, Claro A. Bright light: microspectrofluorimetry for the characterization of lake pigments and dyes in works of art. Acc Chem Res. 2010;43(6):857–66. https://doi.org/10.1021/ar9001894.
Claro A, Melo MJ, Schäfer S, Seixas de Melo JS, Pina F, van den Berg KJ, Burnstock A. The use of microspectrofluorimetry for the characterization of lake pigments. Talanta. 2008;74(4):922–9. https://doi.org/10.1016/j.talanta.2007.07.036.
Claro A, Melo MJ, Seixas de Melo JS, van den Berg KJ, Burnstock A, Montague M, Newman R. Identification of red colourants in van Gogh paintings and ancient andean textiles by microspectrofluorimetry. J Cult Herit. 2010;11:27–34. https://doi.org/10.1016/j.culher.2009.03.006.
Castro R, Pozzi F, Leona M, Melo MJ. Combining SERS and microspectrofluorimetry with historically accurate reconstructions for the characterization of lac dye paints in medieval manuscript illuminations. J Raman Spectrosc. 2014;45:1172–9. https://doi.org/10.1002/jrs.4608.
Nabais P, Melo MJ, Lopes JA, Vitorino T, Neves A, Castro R. Microspectrofluorimetry and chemometrics for the identification of medieval lake pigments. Herit Sci. 2018;6:1–1.
Nabais P, Melo MJ, Lopes JA, Vieira M, Castro R, Romani A. Organic colorants based on lac dye and brazilwood as markers for a chronology and geography of medieval scriptoria: a chemometrics approach. Herit Sci. 2021;9(1):1–8.
Claro A. An interdisciplinary approach to the study of colour in Portuguese manuscript illuminations. Doctoral dissertation. Lisbon: Universidade Nova de Lisboa, Faculdade de Ciências e Tecnologia; 2009.
Castro R. The book of birds in Portuguese scriptoria: preservation and access. Doctoral dissertation. Lisbon: Universidade Nova de Lisboa, Faculdade de Ciências e Tecnologia; 2016.
Nabais P. Identity and connections within medieval heritage: color in the illuminated manuscript through the eyes of the molecular sciences and humanities. Doctoral dissertation. Lisbon: Universidade Nova de Lisboa, Faculdade de Ciências e Tecnologia; 2019.
Valeur B, Berberan-Santos MN. Molecular fluorescence: principles and applications. 2nd ed. Weinheim: Wiley; 2012.
Zhang X, Laursen R. Development of mild extraction methods for the analysis of natural dyes in textiles of historical interest using LC-diode array detector-MS. Anal Chem. 2005;77:2022–5. https://doi.org/10.1021/ac048380k.
Lech K. Universal analytical method for characterization of yellow and related natural dyes in liturgical vestments from Krakow. J Cult Herit. 2020;46:108–18.
Shahid M, Wertz J, Degano I, Aceto M. Mohd Ibrahim Khan, Anita Quye, Analytical methods for determination of anthraquinone dyes in historical textiles: A review. Anal Chim Acta. 2019;1083:58–87. https://doi.org/10.1016/j.aca.2019.07.009.
Rafaëlly L, Héron S, Nowik W, Tchapla A. Optimisation of ESI-MS detection for the HPLC of anthraquinone dyes. Dyes Pigments. 2008;77:191–203. https://doi.org/10.1016/j.dyepig.2007.05.007.
Acknowledgements
The authors would like to thank the Bernisches Historisches Museum, the Historisches Museum Basel, the Museum of London, and Nationalmuseet Denmark for allowing access to the knitted textiles. It is a pleasure to thank Meredith Montague and Richard Newman for the spectacular time at the MFA—Museum of Fine Arts, Boston, and the fantastic research. This publication is based upon work from COST Action EuroWeb, CA19131, supported by COST (European Cooperation in Science and Technology).
Funding
Open access funding provided by Royal Library, Copenhagen University Library. This work received support from PT national funds (FCT/MCTES, Fundação para a Ciência e Tecnologia and Ministério da Ciência, Tecnologia e Ensino Superior) through the project REVIVE (2022.01243; https://doi.org/10.54499/2022.01243.PTDC), the projects UIDB/50006/2020 and UIDP/50006/2020, and through a CEEC junior contract awarded to Paula Nabais (2021.01344.CEECIND) and Natércia Teixeira (CEECIND/00025/2018/CP1545/CT0009). It was also supported through a Marie Skłodowska Curie Research Fellowship (grant agreement 656748) and a Stiftelsen Agnes Geijers fond for Nordisk textilforskning grant number FS2019-0056 to Jane Malcolm-Davies; and a Janet Arnold Award from Society of Antiquaries of London to Beatrice Behlen, Museum of London.
Author information
Authors and Affiliations
Contributions
JMD and BB secured permission for sampling and arranged for samples to be taken at the Museum of London (by curatorial and conservation staff). JMD collected all the other samples and researched the provenance and acquisition of the knitted items with additional information researched by BB. PN and MJM coordinated the acquisition and interpretation of the molecular fluorescence measurements. NT secured the HPLC–MS analysis and interpretation. All authors contributed to the content, revision, and approval of the article’s final version.
Corresponding authors
Ethics declarations
Competing interests
The authors declare that they have no competing interests.
Additional information
Publisher's Note
Springer Nature remains neutral with regard to jurisdictional claims in published maps and institutional affiliations.
Supplementary Information
Additional file 1.
Fluorimetry and HPLC-ESI-Q-Orbitrap-MS data.
Rights and permissions
Open Access This article is licensed under a Creative Commons Attribution 4.0 International License, which permits use, sharing, adaptation, distribution and reproduction in any medium or format, as long as you give appropriate credit to the original author(s) and the source, provide a link to the Creative Commons licence, and indicate if changes were made. The images or other third party material in this article are included in the article's Creative Commons licence, unless indicated otherwise in a credit line to the material. If material is not included in the article's Creative Commons licence and your intended use is not permitted by statutory regulation or exceeds the permitted use, you will need to obtain permission directly from the copyright holder. To view a copy of this licence, visit http://creativecommons.org/licenses/by/4.0/. The Creative Commons Public Domain Dedication waiver (http://creativecommons.org/publicdomain/zero/1.0/) applies to the data made available in this article, unless otherwise stated in a credit line to the data.
About this article
Cite this article
Nabais, P., Malcolm-Davies, J., Melo, M.J. et al. Early modern knitted caps (fifteenth to sixteenth centuries): analyzing dyes in archaeological samples using microspectrofluorimetry complemented by HPLC–MS. Herit Sci 11, 220 (2023). https://doi.org/10.1186/s40494-023-01020-4
Received:
Accepted:
Published:
DOI: https://doi.org/10.1186/s40494-023-01020-4